Exploring the Diverse Roles of RNA Probes
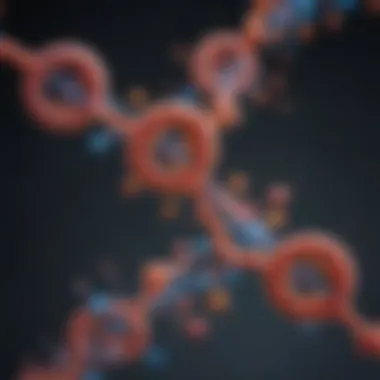
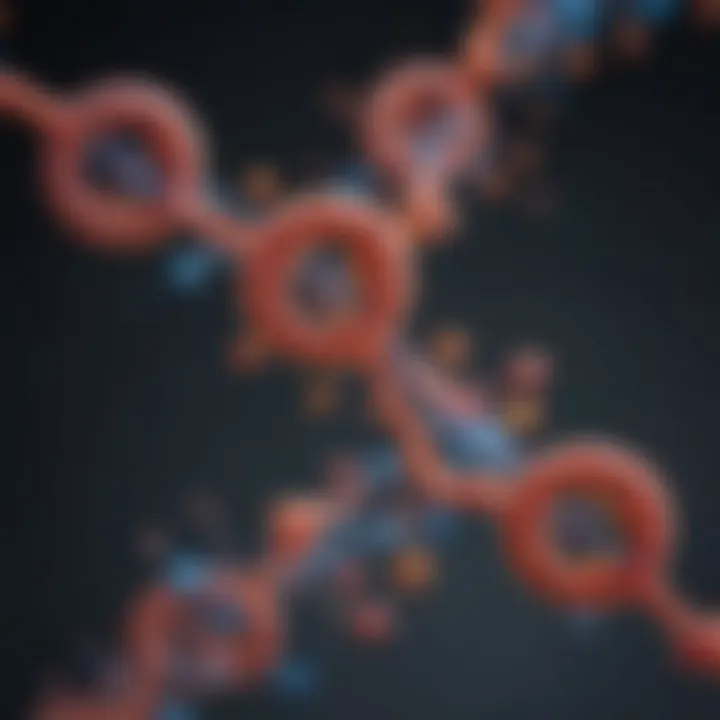
Intro
In the landscape of molecular biology, RNA probes have emerged as vital tools that bridge the knowledge gap between genetic sequences and their functional roles within cells. These meticulously designed strands of RNA provide scientists with the ability to detect, quantify, and analyze specific nucleic acid targets, ultimately unraveling the complex tapestry of gene expression. With a variety of applications spanning diagnostics, research, and therapeutic fields, understanding RNA probes is truly pivotal for anyone navigating this energetic domain of science.
Capturing the Essence of RNA Probes
RNA probes are unique in their ability to bind specifically to complementary RNA targets. This binding forms the foundation for numerous investigative techniques, allowing for precise exploration of genes and their expression patterns. With distinct types like labeled, antisense, and hybridization probes, they play a role in diverse methodologies, such as RNA in situ hybridization and quantitative PCR. Each variation brings a different strength to the table, allowing researchers to tailor their approach based on the particularities of their study.
Historical Significance
The journey of RNA probes is like a fascinating tale of scientific evolution. From the early days of molecular biology when basic techniques were developed, to contemporary advancements that refine these methods, the historical evolution paints a vivid picture. Notably, the discovery of RNA itself brought a new dimension to how genetic information is transmitted and expressed. As researchers navigated the complex world of RNA, they began to harness its potential, creating probes that could track its presence and abundance across different biological contexts.
Implications for Genetic Research
RNA probes are more than mere tools; they represent deeper insights into the realms of genetics and beyond. They have found substantial relevance in understanding diseases at the molecular level, leading to earlier diagnostics and more tailored therapeutics. For instance, in cancer research, different RNA probes can help identify variations in gene expression that signal tumor presence or progression. The implications of their use ripple through chemistry, medicine, and beyond, illuminating pathways for future scientific endeavors.
Through this exploration, we aim to underscore the indispensable role RNA probes play in modern research and diagnostics, serving not just as instruments, but as keys unlocking new realms of genetic knowledge.
Preface to RNA Probes
The growing field of molecular biology continually reveals the profound significance of RNA probes. These small yet powerful tools are at the forefront of genetic research and diagnostics. Understanding RNA probes is crucial for anyone diving into gene expression analysis, pathogen detection, or even innovative therapeutic strategies. With the increasing complexity of biological systems uncovered by advances in technology, RNA probes have become indispensable in unraveling the intricate mechanisms behind gene regulation and function.
Definition and Importance of RNA Probes
RNA probes are essentially single-stranded RNA molecules designed to bind to complementary RNA sequences. This binding facilitates the detection and quantification of specific RNA targets within cells or tissues. Their importance cannot be overstated. For instance, in gene expression studies, RNA probes enable researchers to ascertain the presence and levels of specific transcripts, providing insights into cellular activity and function.
The applications extend beyond mere academic interest; they play a pivotal role in clinical diagnostics, especially in identifying pathologically altered RNA associated with diseases. Moreover, RNA probes assist in monitoring therapeutic responses, tailoring treatments based on individual patient needs. The versatility of these probes makes them invaluable in both basic and applied sciences.
Historical Context and Development
The journey of RNA probes began in the late twentieth century, when molecular biologists sought effective methods to manipulate and understand RNA. Early probes were relatively unsophisticated, often relying on radioactive labeling which presented challenges regarding safety and waste management. Over time, advancements in chemistry and biophysics transformed these crude tools into sophisticated entities capable of precise targeting.
For example, the introduction of fluorescent labeling marked a significant leap forward, allowing for real-time imaging of RNA in living cells. Innovations did not stop there; as researchers learned more about RNA structures, the design of probes became more refined, encompassing considerations of stability, binding efficiency, and specificity. This evolution mirrors the broader trends in molecular biology, wherein technological advancements continually redefine research capabilities.
"The history of RNA probes is a story of ingenuity, where each advancement reflects our growing understanding of molecular mechanisms."
Fundamentals of RNA Probes
Understanding the fundamentals of RNA probes is pivotal in the landscape of molecular biology. They serve as the backbone of various techniques to investigate gene expression, cellular pathways, and more. Knowing the key elements—like composition, binding mechanisms, and underlying principles—lays the groundwork for deeper discussions on their applications and effectiveness.
Composition and Structure
Nucleotide Sequence
The nucleotide sequence of an RNA probe dictates its specificity and efficiency in binding to target RNA molecules. A well-designed sequence is crucial for ensuring that the probe will hybridize effectively with its complement. For instance, sequences with high homology to the target region increase the likelihood of successful hybridization, making them a popular choice for various experimental designs.
One unique feature to note is the ability to incorporate modified nucleotides to enhance the probe's stability and binding properties. This can significantly benefit binding in environments with a high degree of sequence variability, ensuring that even closely related target sequences can be distinguished effectively. However, the complexity added by modifications can sometimes come with trade-offs, potentially affecting the probe's overall performance in certain applications.
Secondary Structure
The secondary structure of RNA probes is also a critical player in their functionality. The way the sequence folds can affect accessibility to the target RNA, dictating how efficiently they can bind. Probes that maintain a stable, predictable secondary structure tend to outperform others, particularly in hybridization techniques requiring temperature variations.
This characteristic highlights a strategic angle: optimizing secondary structure can lead to enhanced hybridization under specific experimental conditions. However, the challenge arises in balancing stability with flexibility; too rigid a structure might miss transient binding sites, while too flexible could lead to nonspecific interactions.
Stability Factors
Stability factors are paramount when discussing RNA probes, as they directly influence the probes' longevity under various conditions. Factors such as temperature, pH, and ionic strength are key determinants of stability. This can be a fine line to walk, as conditions optimal for probe stability might not always align with those ideal for target RNA stability.
One significant aspect of stability is the potential use of stabilizing agents, such as certain salts, which can stabilize the hybridization complex by shielding negatively charged phosphate groups. However, it is important to keep in mind that that the inclusion of such agents may introduce variables that can complicate the interpretation of experimental results.
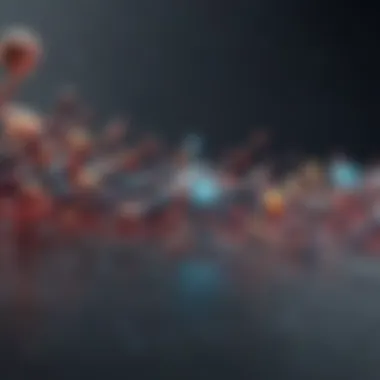
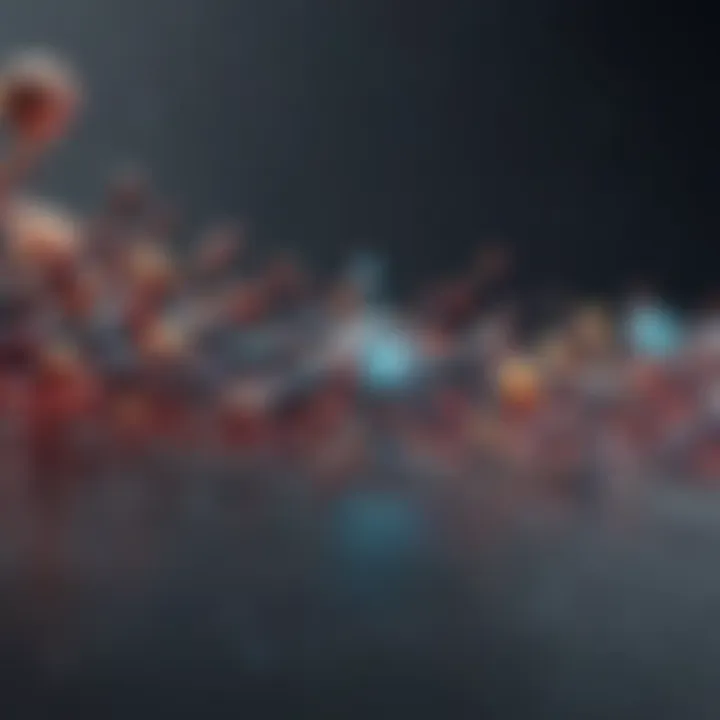
Binding Mechanisms
Hybridization Principles
Hybridization principles underlie the very essence of RNA probe technology. At their core, these principles dictate how well an RNA probe interacts with its target. The complementarity of the nucleotide sequences ensures that the probe locates its target efficiently. The ability to fine-tune conditions such as temperature and reaction composition further enhances hybridization efficiency, making this an essential area of study.
The unique feature of hybridization lies in its ability to shift based on environmental conditions—this adaptability can bring robustness to different experimental setups. However, care must be taken, as improper conditions can lead to weak or nonspecific binding, potentially skewing results.
Temperature and Salt Effects
Temperature and salt concentration are two key factors that greatly influence RNA probe binding. Generally, higher temperatures can promote unwinding of nucleic acid structures, thereby potentially facilitating hybridization. However, they also bring risks by increasing the likelihood of destabilizing interactions. Likewise, salt concentration impacts the electrostatic interactions between the probes and target; a necessary balance must be maintained.
Raising salt concentration typically enhances stability, yet excessive levels can also hinder probe performance. Thus, optimizing these parameters in protocols must be approached with care, taking into consideration the specific objectives of the research.
Specificity Challenges
While specificity is a desirable characteristic in RNA probes, achieving high specificity can come with its own set of challenges. In environments where numerous similar sequences exist, distinguishing between a target and its close relatives can be daunting. This means that researchers have to design probes with sequences unique enough to minimize cross-hybridization, but not so unique that they fail to bind altogether.
A distinguishing feature of specificity lies in the sequence length and composition of the probes. Shorter probes may bind less specifically, as they offer less sequence coverage, while longer probes come with their own difficulty—balancing length and stability. Thus, navigating these specificity challenges is crucial for the reliable application of RNA probes across different research contexts.
Types of RNA Probes
Understanding the varied types of RNA probes is crucial in molecular biology, as each type offers distinct advantages and potential applications. This section sheds light on how different RNA probes function and their unique characteristics, making it easier to choose the appropriate tool for specific research needs. From detecting gene expression to analyzing tissue samples, the type of RNA probe selected can significantly influence the accuracy and efficiency of the results.
In Situ Hybridization Probes
Detection Methods
Detection methods for in situ hybridization probes revolve around their ability to locate specific RNA molecules within fixed tissue samples. One essential aspect of these methods is the use of labeled probes, which can be tagged with either radioactive isotopes or fluorescent dyes. Both approaches offer their own sets of benefits.
Radioactive probes, while sensitive, require stringent safety protocols and can pose handling difficulties. On the other hand, fluorescent probes are gaining preference due to their ability to provide real-time data and the convenience of imaging systems available today. They allow researchers to visualize the spatial distribution of RNA within cells easily.
A major characteristic of these detection methods is their specificity. The bound probe can give immediate feedback regarding the presence of the target RNA, which is key for confirming gene expression levels in specific regions of interest. However, it’s crucial to note that cross-reactivity can sometimes occur, impacting the results. Therefore, proper control experiments are vital for obtaining reliable data.
Applications in Tissue Analysis
The applications of in situ hybridization probes in tissue analysis are profound. These probes enable researchers to investigate the localization of RNA transcripts within complex tissues, helping to unravel biological functions in their natural context. A significant advantage here is that they maintain the spatial architecture of the tissue, allowing for detailed studies on how gene expression changes in different physiological and pathological conditions.
One unique feature of these applications is the ability to incorporate multiple probes within the same section. This opens the door for multiplexing, where researchers can analyze several RNA targets simultaneously. Such an approach enhances the efficiency of data collection but can complicate interpretation due to potential probe interference. Thus, careful experimental design is paramount to fully utilize this dual probe application effectively.
Fluorescent Probes
Role of Fluorophores
Fluorophores play a pivotal role in the functionality of fluorescent probes. These chemical compounds exhibit fluorescent properties that are crucial for detecting RNA targets. A primary advantage of using fluorophores is their sensitivity. They can amplify the signal generated from bound probes, which is essential when working with low abundance transcripts.
The distinct wavelengths emitted by different fluorophores allow researchers to tailor their studies, choosing specific colors for various RNA targets. Notably, the choice of a fluorophore can greatly influence the outcome of the analysis; some may be brighter or more stable than others, giving rise to the importance of selecting the right tool for the job.
Nevertheless, using fluorophores does come with its share of challenges. Photobleaching—where the fluorophore loses its brightness upon exposure to light—can lead to issues during lengthy experimental procedures. Understanding the behavior of chosen fluorophores under experimental conditions is critical to minimize this risk and ensure reliable results.
Signal Amplification Techniques
Signal amplification techniques are essential in enhancing the visibility of RNA probes, particularly for regions with low transcript expression levels. These techniques can include enzyme-mediated signal boosting or using branched DNA constructs which create a cascade of fluorescent signals from a single RNA molecule.
The key characteristic of these amplification strategies is their capacity to increase sensitivity without compromising specificity. For researchers, amplified signals can rescue data from scenarios where traditional probes might yield weak or uninformative results. This makes signal amplification an attractive option, especially in diagnostics and clinical settings.
Despite the apparent benefits, these techniques can introduce complexity to the experimental setup. Increased sensitivity comes along with the potential for higher background noise, which necessitates a careful balancing act when designing the experiment. The trade-off between clarity of results and ease of interpretation often dictates the choice of methods used.
Microarray Probes
High-Throughput Screening
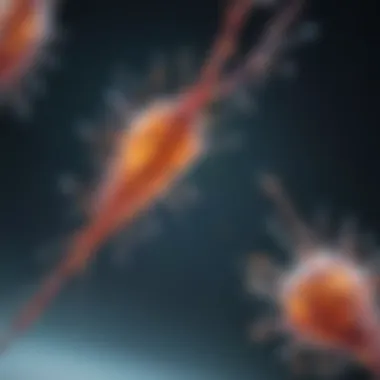
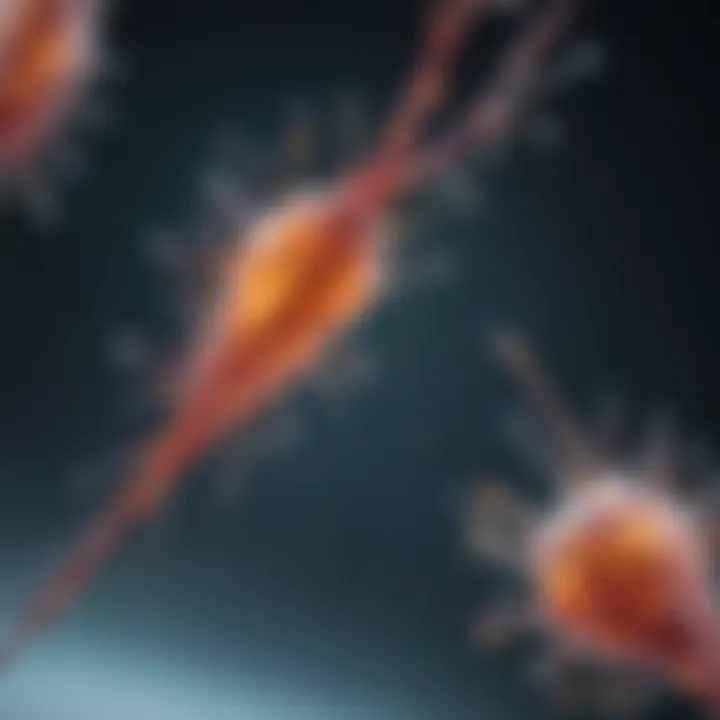
High-throughput screening enabled by microarray probes represents a cornerstone of modern genetic research. This technique allows simultaneous analysis of thousands of RNA molecules, making it an invaluable tool for comprehensive gene expression studies. The primary benefit lies in its ability to deliver vast amounts of data quickly, facilitating studies that were previously time-prohibitive.
A unique feature here is the capability to compare gene expression profiles across different conditions or time points within one experiment. This comparative nature has made microarrays instrumental in understanding diseases, pharmacogenomics, and ecological responses.
However, interpreting large datasets can sometimes resemble finding a needle in a haystack. The complexity of data generated demands sophisticated computational tools for accurate analysis and meaningful results, which can represent a barrier for some researchers without access to these resources.
Data Interpretation
The interpretation of data from microarray probes is crucial and can often make or break the success of the study. Given the wealth of information produced, it’s essential for researchers to have a solid grasp of statistical methods and bioinformatics tools. The ability to differentiate meaningful signals from background noise is a necessary skill in this context.
A key characteristic of data interpretation is its dependence on appropriate controls and normalization procedures. These practices ensure that the data reflects true biological changes and not artifacts of the experiment. Additionally, advanced algorithms are frequently employed to handle large datasets, which can either enhance understanding or, if misapplied, lead to misleading conclusions.
Despite these challenges, mastery of microarray data interpretation paves the way for discoveries in gene function and regulation, solidifying its role in molecular biology research. The ongoing evolution of data analysis tools continues to enhance this process, ensuring that high-throughput screening remains a vital resource in the field.
Methods of RNA Probe Utilization
In the landscape of molecular biology, effectively employing RNA probes is instrumental in unraveling the complexities of gene expression. The importance of this topic lies in its direct implications for research methodologies and data interpretation. From pinpointing gene activity to understanding the minutiae of pathological conditions, these probes serve as essential tools. Delving into the various methodologies involved, one can appreciate how nuances in probe design, experimental procedures, and specific applications collectively enhance the potential of RNA probes.
Probe Design Considerations
Target Selection
When it comes to design considerations, effective target selection holds a notable position. The choice of the target sequence is paramount, as it directly influences the specificity and sensitivity of the probe. A well-chosen target means higher chances of accurately indicating where a gene is active or inactive. One significant characteristic of optimal target selection is the aim for a unique region, ideally less likely to have homology with unintended sequences. This singular approach not only minimizes background noise but also maximizes the clarity of results. However, selecting a target isn’t without challenges; as many genes share similar sequences, misinterpretation can easily flip the script, leading researchers astray.
Length and Composition
The parameters of length and composition of RNA probes add yet another layer of complexity. Generally, a longer probe can enhance specificity due to extended sequence complementarity. On the flip side, the longer the probe, the more intricate the synthesis and stability can become. A characteristic of a well-composed probe is its GC content; a balanced composition can improve binding stability while also addressing the risk of nonspecific binding. However, overemphasizing length for specificity can inadvertently introduce performance variability in different environmental conditions. In summary, there is an art in determining the right length and composition, as both factors significantly influence the ultimate efficacy of the probe.
Experimental Protocols
Preparation of Samples
Once the probes are designed, proper sample preparation becomes indispensable for any successful experiment. This step involves isolating RNA from cells or tissues in a manner that maintains both integrity and bioactivity. Typically, the process begins with the lysis of cells, followed by extraction procedures using kits designed for high yield and quality. A key characteristic of sample preparation is to maintain clean handling techniques to prevent contamination, which can severely compromise results. Unique features of this part of the methodology could include monitoring RNA integrity via gel electrophoresis, allowing researchers to visualize degradation or purity issues. Though often thought of as an inconvenient step, a precise preparation process lays the essential groundwork for accurate experimental outcomes.
Probe Application Techniques
After thorough preparation, the application of the RNA probe to the sample is a crucial step in the overall experimental workflow. Various techniques can be employed, ranging from in situ hybridization to solution hybridization, each with its unique set of parameters and potential. A primary characteristic of effective probe application is control over environmental conditions—temperature and ionic strength play pivotal roles in achieving proper hybridization. One intriguing feature is the advent of automation in probe application. While manual techniques suit many labs, automation increases throughput and minimizes human error. However, one must also be aware that automation often demands a higher initial investment and could introduce a learning curve for laboratory personnel. All said, selecting the right application technique is vital as it determines not just the ease of experiment, but also influences data quality.
Understanding the intricacies of RNA probe utilization is imperative not just for innovating in the field but also for cementing the reliability of results in research endeavors.
Thus, the methods of RNA probe utilization encapsulate the multi-faceted steps required to ensure effectiveness. Each method adds layers of complexity but also opportunities for more profound discoveries in molecular biology.
Applications in Research
The applications of RNA probes are vast and multifaceted, which makes them integral to modern molecular biology. They serve as powerful tools for studying gene expression and detecting pathogens. By utilizing various techniques, researchers can draw meaningful insights into biological processes and the health of ecosystems or individuals. This exploration provides a clear understanding of why RNA probes are pivotal in both research and clinical settings.
Gene Expression Analysis
Gene expression analysis enables scientists to understand which genes are active under specific conditions. This information is crucial for unraveling the complexities of cellular functions and can also be applied to therapeutic development.
Quantitative Techniques
Quantitative techniques with RNA probes, such as reverse transcription quantitative PCR (RT-qPCR), allow researchers to measure gene expression levels precisely. The key characteristic of this approach is its ability to provide numerical data, enabling comparisons across different samples.
One major benefit of using quantitative techniques is their sensitivity; they can detect low abundance transcripts, which could be critical in understanding diseases like cancer. However, one unique feature worth noting is that these techniques often require careful standardization, as small variations can greatly affect results, making reproducibility sometimes challenging.
Qualitative Assessment
On the other hand, qualitative assessment through RNA probes, such as in situ hybridization, allows researchers to visualize gene expression within the context of tissue architecture. This approach highlights the spatial distribution of RNA, providing insights that quantitative methods might overlook.
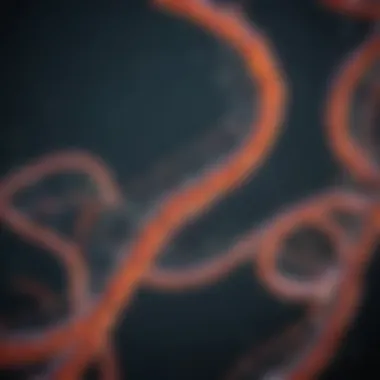
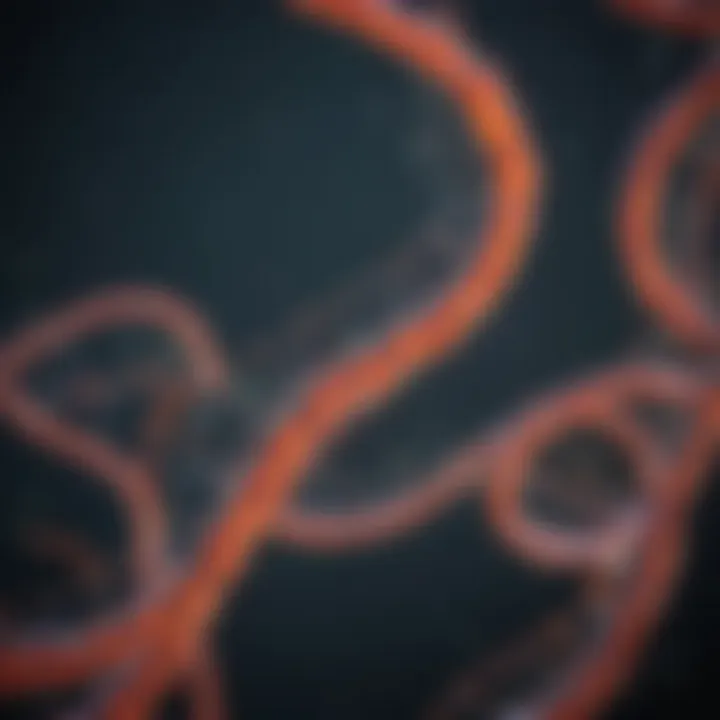
What sets qualitative assessments apart is their ability to give a more comprehensive picture of gene activity in specific cell types. A benefit of this method is its intuitive appeal; researchers can see exactly where specific RNA is located in a sample. The trade-off may involve potential subjective interpretation of results, leading to variability in conclusions drawn by different researchers.
Detection of Pathogens
The role of RNA probes in pathogen detection is another vital application, particularly in clinical diagnostics. They are instrumental in identifying viral and bacterial infections in a timely manner. For instance, RNA probes can help detect the RNA of viruses like influenza or the novel coronavirus with great precision and speed.
RNA Probes in Clinical Diagnostics
In clinical diagnostics, RNA probes have completely transformed the landscape. They facilitate the identification of pathogens at the molecular level, making it easier to diagnose diseases based on the presence of specific RNA sequences. A distinctive characteristic of using RNA probes in this context is the rapid turnaround time for test results, which is critical in managing infectious diseases effectively. However, the unique feature of needing specialized equipment for testing can be a limitation in developing regions with fewer resources.
Environmental Monitoring
Environmental monitoring utilizes RNA probes to assess the health of ecosystems, focusing on detecting and quantifying pathogens within environmental samples. This is particularly relevant in the context of emerging infectious diseases and monitoring biodiversity.
The key strength of environmental monitoring lies in its capability to analyze complex samples, offering insights into the presence of contaminants in water or soil. An added advantage is the potential for early detection of disease outbreaks or ecological disturbances. Despite their benefits, the complexity of sample preparation and the need for substantial secondary validation can pose challenges in this application.
Innovations and Future Directions
The field of molecular biology is continuously evolving, particularly in the realm of RNA probes. The significance of innovations and future directions cannot be overstated. These elements not only reflect current advancements but also pave the way for enhanced capabilities in genetic research and diagnostics. As society strives to understand complex biological systems, addressing the need for more precise, efficient, and ethical methodologies becomes paramount. Advancements may lead to breakthroughs that revolutionize our comprehension of cellular mechanisms and disease paths.
Emerging Technologies
CRISPR Integration
A major leap in research methodology is the integration of CRISPR technology with RNA probes. This powerful system, which enables targeted genetic editing, has qualities that make it a game changer in the field. The core advantage lies in its precision, allowing researchers to edit specific sequences of RNA with remarkable accuracy. CRISPR, or Clustered Regularly Interspaced Short Palindromic Repeats, empowers scientists to understand gene functionalities, manipulate gene expression, and even address genetic disorders at their root. This technology’s adaptability to various applications, from therapeutic development to functional genomics studies, underscores its value.
However, there are nuances to consider. While CRISPR is a popular choice, its implementation can introduce complexities that require careful navigation, particularly in design and specificity. Challenges in achieving perfectly tailored probes can result in off-target effects, which must be meticulously evaluated to mitigate potential complications in experimental outcomes.
Nanotechnology in Probing
Exploring the integration of nanotechnology into RNA probing brings forth another layer of potential. The hallmark of nanotechnology in this context is its ability to enhance sensitivity and specificity in detection methods. Utilizing nanoscale materials facilitates the development of highly sensitive probes that can detect low-abundance RNA sequences effectively. This represents a significant benefit, especially in cancer research where early-stage detection is crucial.
Nevertheless, inherent challenges exist within nanotechnology as well. While the unique properties of nanoparticles can amplify signaling, interactions between the probes and biological environments may yield unpredictable outcomes. Therefore, understanding these interactions and mitigating risks becomes integral to ensuring the reliability of probing outcomes.
Potential Challenges
Design Limitations
Despite the vast promise of RNA probes, design limitations present a considerable hurdle. Designing effective probes demands meticulous consideration of factors such as sequence specificity, length, and stability. One critical point is that if a probe is too short, it may not bind effectively to its target, whereas overly long probes may lead to hybridization with unintended targets, thereby reducing the accuracy of results.
Addressing these design limitations requires a delicate balance. Researchers must remain cognizant of the relationship between probe design and experimental reliability. Striking this balance is pivotal in ensuring that results reflect true biological phenomena rather than artefacts of probing sources.
Ethical Considerations
As research thrusts forward, ethical considerations remain a pressing concern in the development and application of RNA probes. Genetic research often treads into sensitive domains, and the implications of manipulating RNA, particularly in human cells, warrant careful scrutiny. Questions surrounding consent, potential misuse of technologies, and the ramifications of genetic editing are paramount.
A pivotal aspect of ethical considerations hinges on transparency and community engagement. The scientific community must strive for open dialogue regarding the capabilities and limits of RNA probe technologies. This dialogue aids in establishing trust among stakeholders, ensuring advancements are socially responsible and benefit society at large.
"The evolution of RNA probe technology must be matched by a commitment to ethical stewardship and public awareness."
By addressing these challenges proactively, the research community can harness the full potential of RNA probes while preserving the integrity of the scientific endeavor.
Closure
The conclusion serves as the culmination of our deep dive into RNA probes, neatly tying together the various threads explored throughout this article. Understanding RNA probes not only enhances our grasp of molecular biology but also reinforces their significance in contemporary scientific research. These tools have become essential in elucidating gene expression, diagnosing diseases, and developing potential therapies.
Summary of Findings
Through our exploration, we uncovered several pivotal aspects of RNA probes that stand out:
- Diverse Applications: RNA probes are versatile instruments employed in various research settings, from basic science to clinical diagnostics.
- Technological Advances: Innovations such as CRISPR integration and nanotechnology continually refine the functionalities and efficiencies of RNA probes, broadening their scope.
- Methodological Rigor: Attention to detail in probe design and application protocols is crucial for obtaining reliable results, underscoring the need for expertise in utilizing these tools effectively.
As a result of these findings, it is clear that RNA probes represent a bridge between theory and practice in molecular biology, driving forward discoveries that can have profound implications for health and disease.
Impact on Future Research
Looking ahead, the implications of RNA probes in research seem boundless. The continual refinement of probe design, coupled with emerging technologies, is set to fuel future discoveries. Noteworthy points include:
- Enhanced Precision: Advances in nanotechnology can lead toward more specific probes that target unique RNA sequences with high fidelity, minimizing off-target effects in experimental setups.
- Integration with Diagnostic Platforms: The collaboration between RNA probing and genomics may establish earlier and more accurate detection methods for diseases, significantly impacting patient outcomes.
- Ethical Challenges: As we progress into areas such as gene editing and personalized medicine, ethical considerations linked to RNA probe use demand ongoing discourse within the scientific community.
"RNA probes are not just tools; they are keys that unlock the vast potential of gene research and application in improving human health."