Understanding Uracil Pairing in RNA Dynamics
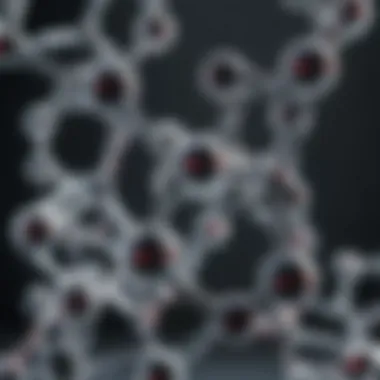
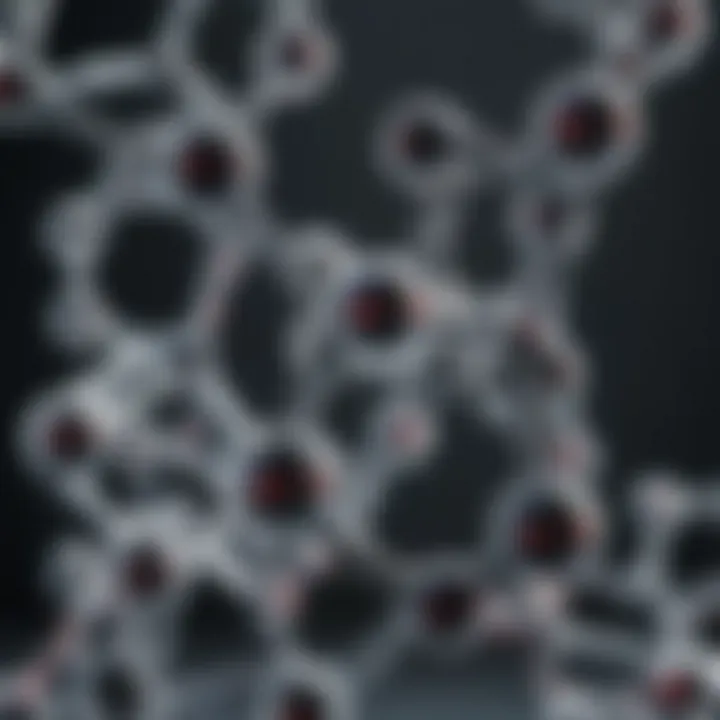
Intro
Uracil is a central component of RNA, yet its pairing mechanisms often receive less attention compared to other bases like adenine or cytosine. Understanding uracil's role is crucial for grasping RNA's structure and function. This section aims to clarify why uracil pairing warrants deeper investigation and how it influences essential biological processes, such as replication and transcription. By exploring its unique contributions, we can better appreciate the complexities of RNA dynamics.
Research Overview
Summary of Key Findings
Research on uracil pairing has shown several important findings.
- Pairing with Adenine: Uracil primarily pairs with adenine through hydrogen bonds, similar to thymine in DNA.
- Stability: The presence of uracil in RNA can impact overall stability. Studies illustrate that uracil leads to dynamic structures, unlike the more rigid DNA.
- Functional Implications: The specific pairing can influence the efficiency of ribozymes and the recognition of RNA by proteins.
These findings underline uracil's essential part not only in maintaining RNA's functionality but also in its evolution.
Relevance to Current Scientific Discussions
In light of recent discoveries, understanding uracil pairing is more relevant than ever. With RNA's importance in various biological processes, including gene expression and regulation, discussions surrounding uracil's role have gained momentum. Recent literature is pointing to potential applications in synthetic biology and therapeutics, where manipulating RNA structures can lead to innovative treatments.
Additionally, ongoing debates in molecular biology emphasize how uracil pairing differs from thymine's role in DNA. This distinction enhances our understanding of genetic fidelity and mutation rates, contributing insights into evolutionary biology.
Methodology
Research Design and Approach
Investigation into uracil pairing often employs a combination of experimental and computational methods. Researchers utilize techniques such as X-ray crystallography and NMR spectroscopy to visualize RNA structures. Complementary computational modeling helps predict behaviors and dynamics, ensuring a robust understanding of the interactions at play.
Data Collection and Analysis Techniques
Data is typically collected from various experimental setups. For instance, crystallization experiments yield structural data, while biochemical assays help assess the stability of uracil pairs under different conditions. Analysis involves comparing these results to draw conclusions about uracil's functional significance in RNA.
"Understanding uracil's role in RNA offers insights that have potential implications for both fundamental biology and applied sciences."
In sum, the methodologies employed are critical for shedding light on the complexities of uracil pairing and its implications on RNA functionality.
Preamble to RNA and Its Components
The introduction to RNA and its components serves as the foundation for understanding the role of uracil in RNA pairing. A comprehensive grasp of RNA is pivotal for appreciating how uracil functions, particularly in relation to other nucleobases. RNA, or ribonucleic acid, is essential for various biological processes, including coding, decoding, regulation, and expression of genes. It is also crucial in the synthesis of proteins, which are vital for cellular functions across all life forms.
The importance of RNA extends beyond mere structure; it influences the dynamics of cellular mechanisms. All these aspects set the stage for a deeper exploration of uracil, underscoring its significance within the broad context of nucleic acid chemistry. This exploration will elucidate the critical interactions that take place during RNA structure formation and function.
Basics of RNA Structure
RNA is primarily composed of a long chain of nucleotides, comprised of three main components: a ribose sugar, a phosphate group, and a nitrogenous base. The structure of RNA can be single-stranded or, in some cases, double-stranded. The specific configuration allows RNA to fold into complex three-dimensional shapes, essential for its function as a messenger and in catalysis.
Each of the four nucleobases—adenine, guanine, cytosine, and uracil—contributes to the unique properties of RNA. These bases interact through hydrogen bonding, forming base pairs that stabilize RNA structures. Notably, uracil replaces thymine, which is found in DNA, providing RNA a distinct identity.
Role of Nucleobases in RNA
Nucleobases play a profound role in the stability and functionality of RNA. Each base contributes to the overall molecular architecture and influences how RNA molecules bind with other biomolecules. The pairing mechanisms among nucleobases, particularly between uracil and adenine, underpin the process of transcription.
Key points regarding the role of nucleobases include:
- They determine the genetic coding and regulatory functions within cells.
- Different combinations of nucleobases lead to diverse proteins and functional RNA molecules.
- The presence of uracil modifies the interaction dynamics significantly, compared to thymine in DNA.
Understanding these bases is integral to grasping how RNA operates at various levels, from basic cellular activity to complex organismal biology.
Uracil: The Unique Nucleobase
Uracil holds a vital and distinct position within the alphabet of nucleobases that compose RNA. This unique nucleobase is a key player, contributing to the complexity and functionality of RNA structures. Its incorporation in RNA instead of thymine, which is commonly found in DNA, underscores its specialized role in cellular processes. Understanding uracil is essential for grasping RNA's diverse mechanisms in transcription, translation, and its overall biological implications. This section will delve into the particular chemical aspects of uracil and draw comparisons with thymine, emphasizing its importance in the structure and behavior of RNA.
Chemical Composition of Uracil
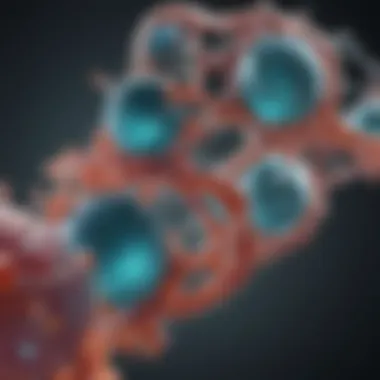
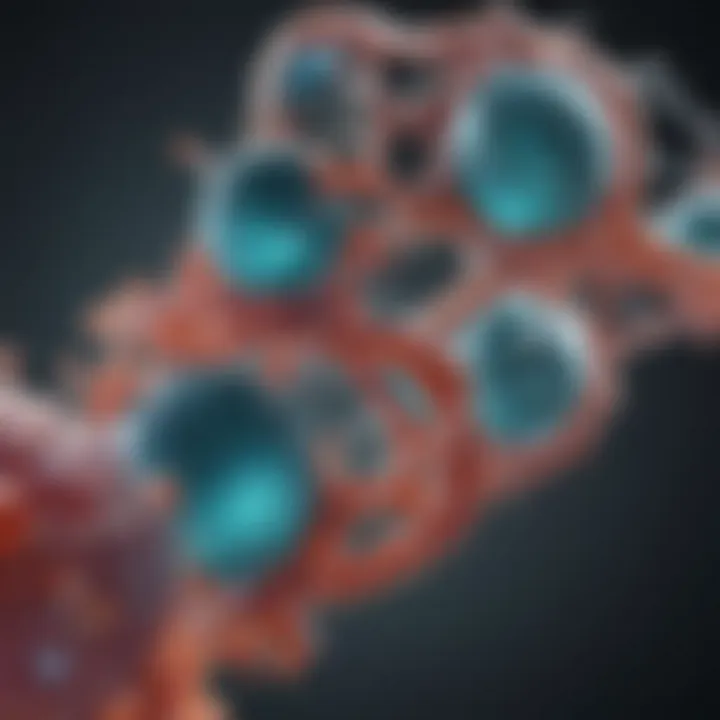
Uracil is classified as a pyrimidine nucleobase. Its chemical formula is C4N2O2. The structure consists of a six-membered ring containing two nitrogen atoms and four carbon atoms. Additionally, it has two oxygen atoms, which play a critical role in the ability of uracil to participate in hydrogen bonding. This bonding capability is central to its function in pairing with adenine in RNA. The absence of a methyl group in uracil, which is present in thymine, affects not just its pairing actions but also its stability when integrated into RNA chains.
Comparison with Thymine
While uracil and thymine may seem similar, they are distinctly different. Thymine has a methyl group at the 5-position, which uracil lacks. This addition in thymine contributes to DNA's stability and structural integrity.
In terms of pairing, uracil undergoes hydrogen bonding with adenine during RNA formation. The absence of the methyl group in uracil results in different bonding energies and affinities. To illustrate these differences:
- Stability: Thymine pairs with adenine using two hydrogen bonds; uracil also pairs using two bonds, but the interactions can be less stable.
- Occurrence: Thymine is typically found in DNA, whereas uracil is a hallmark of RNA.
- Biological Role: While both nucleobases are involved in controlling genetic information, uracil's presence in RNA reflects its role in quickly producing proteins, allowing for more dynamic functions within cells.
Understanding the differences between uracil and thymine helps illustrate why RNA is suitable for its roles, especially in processes like transcription and translation. This distinction in structure plays a crucial role in the unique characteristics of RNA.
Pairing Mechanisms in RNA
Understanding the pairing mechanisms in RNA is essential, as it contributes to the overall stability and functionality of RNA molecules. These mechanisms dictate how nucleobases interact, impacting processes such as transcription and translation. The efficient pairing of nucleobases ensures that RNA can perform its role in protein synthesis and gene regulation. In the context of uracil, its unique interaction with adenine forms the basis of vital RNA structures.
The Watson-Crick Model
The Watson-Crick model describes how nucleobases interact through specific hydrogen bonding patterns. This model underpins the basic structure of nucleic acids, showcasing the complementary nature of base pairing. For uracil, it pairs specifically with adenine through two hydrogen bonds. This interaction is not just functional; it stabilizes the RNA structure as a whole.
In addition, the Watson-Crick pairing enhances the fidelity of RNA replication and transcription. This ensures accurate messaging from DNA to RNA with minimal errors. The nuances of this model highlight the importance of base pairing in maintaining genetic integrity, which is central to the process of life itself.
"The Watson-Crick model illustrates the elegance and precision of nucleobase interactions, which are critical for biological processes."
Uracil and Adenine Pairing
Uracil's pairing with adenine is fundamental in RNA biochemistry. This specific pairing facilitates rapid chain elongation during RNA synthesis. The two hydrogen bonds formed in this interaction are relatively weak, allowing for transient stability, which suits the dynamic nature of RNA function.
Compared to thymine, uracil's ability to participate in similar bonding patterns with adenine gives RNA a distinct functional flexibility. This characteristic is important in various cellular processes, especially those involving RNA viruses, which exploit this flexibility for rapid replication.
During the translation process, the pairing of uracil with adenine ensures that genetic information is conveyed correctly during protein synthesis. The difference in pairing dynamics can influence the rates of transcription and the subsequent translation phases, thus affecting overall protein production.
Indeed, understanding this specific pairing highlights uracil's vital role in biochemistry. It shows why uracil is considered an integral part of RNA's diverse functionalities within biological systems.
The Biochemical Significance of Uracil Pairing
Uracil plays a critical role in the structure and function of RNA. Its pairing mechanisms directly impact RNA stability and folding, key elements in biological processes. This section will explore the biochemical significance of uracil pairing in more detail, addressing specific elements, benefits, and considerations regarding this essential nucleobase. Understanding these aspects sheds light on the intricate dynamics of RNA and the implications for genetic information transmission.
Stability of RNA Structures
The stability of RNA structures largely hinges on the interactions between nucleobases. Uracil, in its pairing with adenine, contributes to the overall stability of RNA molecules. The hydrogen bonds formed when uracil pairs with adenine are crucial. These interactions allow RNA to maintain its three-dimensional configuration, which is vital for proper functioning.
Additionally, uracil's unique chemical composition (having a carbonyl group instead of a methyl group found in thymine) allows for distinct interactions with other molecules. This difference affects the molecular dynamics, influencing how RNA can fold and participate in various biological pathways. A stable RNA structure is fundamental for proper cellular activities, such as protein synthesis and gene regulation.
"The stability provided by uracil pairing is not just about maintaining structure; it ensures that RNA can effectively fulfill its roles in cellular mechanisms."
Some benefits of stability include:
- Reduced degradation: Stable RNA is less susceptible to enzymatic digestion, which is essential for maintaining genetic information.
- Functional efficiency: RNA with stable structures is more effective in its roles, whether in encoding proteins or regulating gene expression.
In summary, uracil's role in promoting stability is essential for the reliability of RNA in biological systems.
Impact on RNA Folding
Uracil's pairing with adenine also plays a significant part in RNA folding. RNA is characterized by its ability to form complex three-dimensional shapes. The pairing mechanism involving uracil influences how RNA strands fold and align. Proper folding is necessary for RNA to function correctly, allowing it to engage in catalysis or as a blueprint for protein synthesis.
Furthermore, the presence of uracil influences the secondary structures of RNA. These structures, such as loops and bulges, are essential for the recognition and binding of proteins, which are critical for many cellular processes. Without appropriate folding, RNA molecules may lose their functionality, leading to disruptions in cellular activities.
Factors that affect RNA folding include:
- Environmental conditions: Factors like temperature and ion concentration can impact how RNA molecules fold and stabilize.
- Sequence context: The surrounding nucleobases also influence the structural outcomes of RNA folding involving uracil.
Through understanding uracil's impact on RNA folding, researchers can gain insights into functional RNA dynamics, which is crucial for advancements in biomedical sciences and genetic engineering.
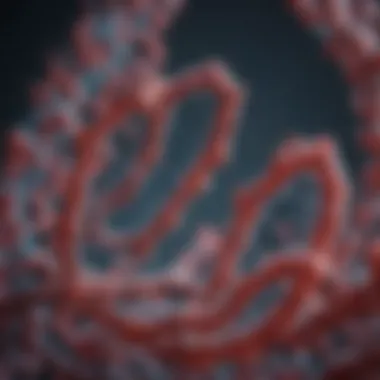
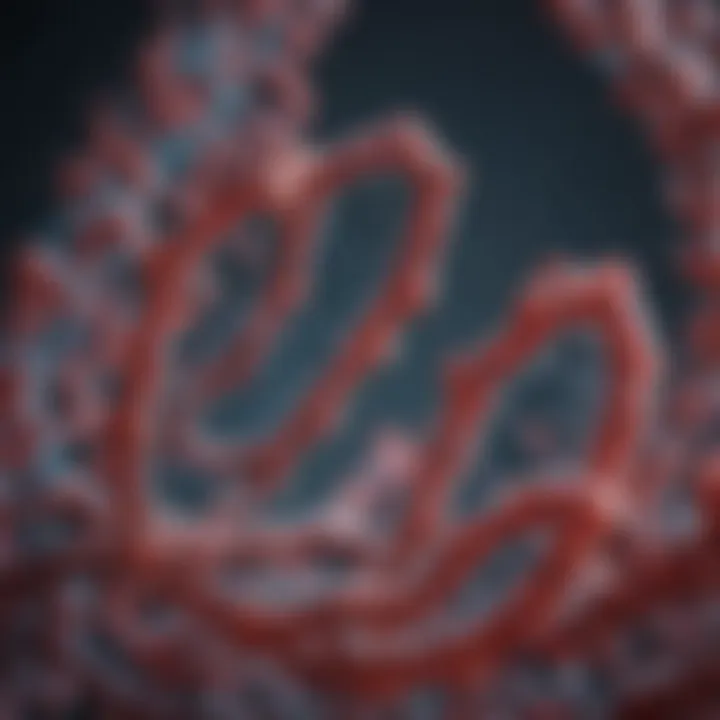
Uracil's Role in Transcription and Translation
Uracil plays a crucial role in both transcription and translation processes of RNA. Understanding this role provides insight into how RNA operates at a molecular level, influencing everything from gene expression to protein synthesis. Uracil pairs with adenine during transcription, which is essential for the formation of RNA molecules that serve various functions within living organisms. This section will delve into the specific aspects of uracil's involvement in transcription and translation, focusing on the significance of these processes in the broader context of molecular biology.
Transcription Process Overview
Transcription is the first step in gene expression, during which a segment of DNA is copied into RNA. This process occurs in three main stages: initiation, elongation, and termination.
- Initiation
- Elongation
- Termination
- The RNA polymerase enzyme binds to a specific region of the DNA, known as the promoter.
- Transcription factors assist in this binding, ensuring the correct gene is expressed.
- The DNA strands unwind, exposing the nucleotide sequence to be transcribed.
- RNA polymerase moves along the DNA template strand, synthesizing the RNA strand by adding nucleotides.
- In this phase, uracil is incorporated instead of thymine, pairing with adenine from the DNA.
- This creates a complementary RNA strand, essential for accurate transcription.
- The process concludes when RNA polymerase encounters a termination signal in the DNA.
- The newly formed RNA molecule, now a primary transcript, detaches from the DNA.
- This RNA will undergo further processing to become mature and functional.
Uracil's incorporation during elongation is a key factor that distinguishes RNA from DNA. Its ability to base pair smoothly with adenine supports the fidelity of transcription, ensuring that genetic information is accurately relayed from DNA to RNA.
Function in Protein Synthesis
Once transcription is completed, the RNA undergoes translation. This is the process where messenger RNA (mRNA) is decoded to synthesize proteins. Uracil's influence continues through this phase.
- Role of mRNA: mRNA, which carries the code from the DNA, contains uracil. The ribosome reads this mRNA sequence in sets of three bases, known as codons. Each codon corresponds to specific amino acids, which are the building blocks of proteins.
- tRNA and Uracil: Transfer RNA (tRNA) molecules have anticodons that are complementary to the mRNA codons. During translation, tRNA molecules deliver the appropriate amino acids to the ribosome. Uracil's pairing capabilities enhance the interactions between tRNA and mRNA, fostering precise amino acid incorporation.
"Transcription and translation are fundamental processes that shape the molecular landscape of life, and uracil plays an essential role in both."
In summary, uracil's functional importance in the transcription process assists in producing accurate RNA molecules, while its presence in translation facilitates proper protein synthesis. Understanding these processes gives valuable insights into molecular biology and highlights the vital contribution that uracil makes to RNA dynamics.
Uracil's Connection to RNA Viruses
Uracil plays a significant role in the realm of RNA viruses. Understanding its interactions and functionalities can provide insight into viral behavior. This section examines how uracil contributes to the lifecycle of RNA viruses, focusing on two main areas: its role in enhancing viral replication and the structural implications it has within viral genomes.
Accelerating Viral Replication
One of the key functions of uracil in RNA viruses is its ability to facilitate rapid viral replication. The incorporation of uracil allows for the efficiency and speed of the replication process. RNA viruses depend heavily on their ability to quickly reproduce to spread effectively within host organisms.
- Increased Mutation Rate: The paired interactions of uracil with adenine can increase the mutation rate during viral replication. This can create diverse viral populations, which is essential for adaptation to changing environments.
- Replication Enzymes: Uracil's presence is crucial for some viral replication enzymes, such as RNA-dependent RNA polymerases. These enzymes are responsible for synthesizing new viral RNA strands. The unique properties of uracil enhance the enzyme's efficiency, leading to faster replication times.
"Uracil allows RNA viruses to adapt rapidly, making them formidable pathogens."
- Influence of Host Factors: The interactions between uracil and host cellular factors can potentially enhance replication rates. Understanding these interactions opens avenues for targeted therapies that can disrupt viral replication by interfering with uracil's efficacy.
Structural Implications in Viral Genomes
The inclusion of uracil in the viral genome has far-reaching structural implications. It influences the stability and structure of RNA molecules, affecting how viruses package their genetic material.
- Stability of RNA Structures: Uracil contributes to the overall stability of the RNA structure. This stability ensures that the genetic material is preserved until it can successfully enter a new host cell.
- Modifications in RNA Folding: The presence of uracil can also alter the folding patterns of RNA, which is critical for its function. Variations in folding can lead to changes in how the virus interacts with host cell machinery.
- Structural Variability: The flexibility introduced by uracil allows for greater variability in how RNA viruses can configure their genomes. This structural adaptability is beneficial for evasion of the host immune response.
Comparative Analysis of Nucleobases
The examination of nucleobases provides invaluable insights into the fundamental mechanisms underpinning RNA functionality. Uracil, as a unique nucleobase, plays a crucial role in the overall landscape of RNA structures and interactions. A detailed comparative analysis ensures not only understanding of each nucleobase's individual characteristics but also their collective contributions to RNA's diverse biological roles. This understanding has implications for both theoretical research and practical applications in genetic engineering and therapeutics.
Uracil vs. Other Nucleobases
Uracil stands apart from other nucleobases, such as adenine, guanine, and cytosine, primarily due to its chemical structure and pairing behavior. Unlike thymine, which is found in DNA, uracil does not possess a methyl group at the carbon-5 position. This subtle difference can significantly alter base pairing properties and RNA stability.
- Chemical Differences: Uracil's lack of methylation results in a lower molecular weight. This impacts the hydrogen bonding capabilities, allowing uracil to pair easily with adenine through two hydrogen bonds, similar to thymine's pairing with adenine in DNA.
- Stability and Flexibility: Pairing with adenine provides RNA flexibility, which is vital for functions such as catalysis and structural roles. While thymine offers a more stable interaction due to its additional methyl group, uracil's pairing with adenine contributes to RNA's secondary and tertiary structures.
- Evolutionary Perspective: Evolutionarily, the presence of uracil in RNA may reflect a primordial form of life. The transition from RNA to DNA could explain why uracil is retained in RNA, highlighting its functional importance even as life evolved.
Uracil's interaction with other nucleobases can influence RNA behavior significantly. Its unique structure and properties result in distinct consequences for RNA folding, stability, and biological interactions.
Influences on RNA Diversity and Functionality
The diversity of RNA structures and functions can largely be attributed to the variations in nucleobases, particularly uracil. The ability of uracil to pair with adenine facilitates numerous RNA functionalities, including the formation of ribozymes and regulatory RNA elements.
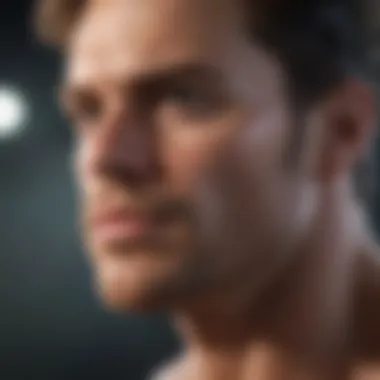
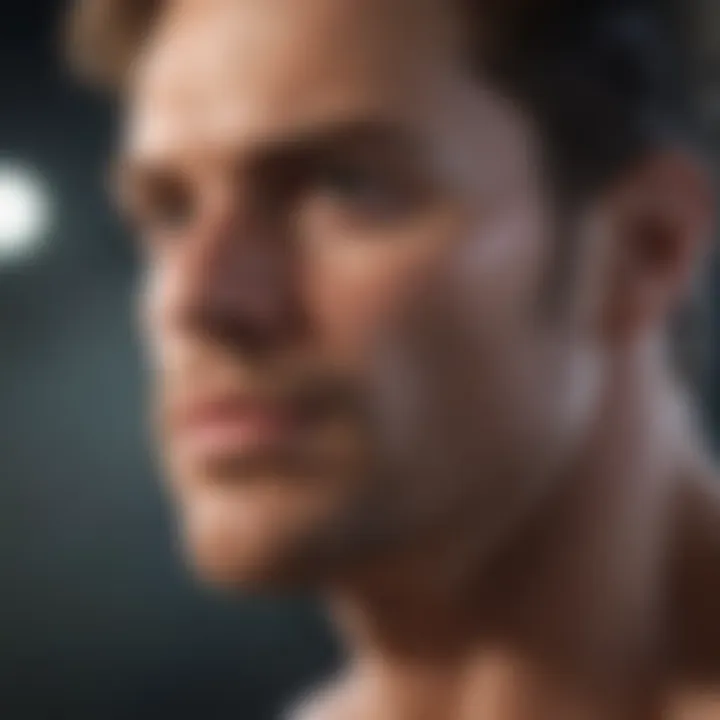
- Ribozymes: These RNA molecules can catalyze biochemical reactions, largely because of the specific interactions provided by uracil. The ability to pair with adenine can create active sites essential for enzymatic functions.
- Regulatory Roles: RNA molecules often engage in complex regulatory networks. Uracil’s pairing ability contributes to its prevalence in various regulatory RNAs, including microRNAs. This regulatory functionality is crucial in gene expression modulation and cellular responses.
- Structural Variability: The presence of uracil allows RNA to adopt various conformations, enhancing the structural complexity inherent in ribonucleic acid functions. This variability supports a wide range of biological activities, including catalysis, signaling, and genetic information storage.
"Understanding how uracil interacts and compares with other nucleobases is essential for grasping the intricate dynamics of RNA biology."
In summary, uracil's role in showcasing the differences and similarities between nucleobases enhances our grasp of RNA's versatility. By exploring these comparative aspects, researchers can design novel strategies for interventions in genetic disorders and therapeutic applications.
Research Advances Involving Uracil
Recent advancements in the study of uracil have shed light on its critical functionalities in RNA. As a pivotal nucleobase with unique pairing characteristics, uracil plays a significant role in various biochemical processes. Understanding the dynamics of uracil, especially its interaction with adenine, is essential for enhancing our grasp of RNA's role in transcription and replication. This section will discuss some key advances in research, highlighting impactful findings and innovations.
New Techniques for RNA Analysis
Innovative techniques have emerged to study RNA with a focus on uracil's properties. Techniques such as high-throughput sequencing and single-molecule imaging are opening new horizons.
- High-Throughput Sequencing: This method allows for the rapid sequencing of RNA, providing comprehensive insight into the presence and distribution of uracil in different RNA strands. Researchers can now examine how uracil's pairing with adenine influences the overall stability of RNA molecules.
- Single-Molecule Imaging: This technique enables the observation of individual RNA molecules in real time. By monitoring the interactions of uracil with adenine and other nucleobases, scientists gain a deeper understanding of RNA dynamics and the conformational changes that occur during transcription and translation.
These advancements facilitate dissecting complex RNA behaviors with a focus on the role uracil plays in various biological contexts.
Implications for Genetic Engineering
Research into uracil also bears significant implications for genetic engineering. As scientists refine their understanding of uracil's pairing behavior, new avenues open for manipulating RNA sequences to achieve desired outcomes.
- CRISPR Technology: The intersection of CRISPR-Cas systems and uracil research has emerged as a promising field. The ability to design RNA guides with specific uracil placements can enhance targeting accuracy in gene editing.
- RNA-based Drugs: Understanding uracil dynamics informs the development of RNA-based therapeutics. By tailoring RNA molecules that interact specifically through uracil-adenine pairing, researchers can create drugs with improved efficacy and specificity in treating diseases.
By leveraging insights from recent studies, genetic engineering benefits tremendously, pushing the boundaries of what is achievable in genomic and therapeutic innovations.
Uracil's role in RNA extends beyond mere structure; its study opens doors to transformative techniques in genetic manipulation, enhancing our grasp on molecular biology.
Future Directions in RNA Research
The realm of RNA research continues to expand rapidly, driven by advances in molecular biology and a deeper understanding of genetic mechanisms. Such growth is essential, particularly in considering how uracil functions within RNA. Future directions in this field hold the potential to usher in significant breakthroughs, particularly in therapeutic applications and innovative technologies.
Potential Therapeutic Applications
Uracil's role in RNA biology makes it a focal point for therapeutic exploration. One promising area is the development of RNA-based treatments for various diseases, including cancer and viral infections. By manipulating uracil interactions, scientists can engineer RNA molecules that target specific pathways. This targeted approach may enhance drug efficacy while reducing side effects associated with traditional treatments.
- Research is ongoing about modifying uracil to improve its stability and effectiveness in therapeutic settings.
- Potential applications include the design of ribozymes and small interfering RNAs that rely on uracil's pairing capacity.
- There is also a focus on the role of uracil in gene expression regulation, which may lead to novel drugs that can silence or activate genes associated with pathologies.
In the context of genetic disorders, there is an increasing interest in how uracil modifications can impact mRNA stability, binding to ribosomes, and subsequent protein synthesis. This understanding might pave the way for personalized medicine strategies, allowing for a more tailored approach in treating genetic defects.
Explorations of RNA-based Technologies
The technological advancements inspired by RNA dynamics, particularly those involving uracil, have vast implications. Future research might delve into new RNA-based technologies with applications across various fields. Some areas under exploration include:
- RNA Nanotechnology: Researchers are investigating how uracil can contribute to the formation of RNA nanoparticles for drug delivery systems. These nanoparticles can transport therapeutic molecules directly to targeted cells, maximizing efficacy.
- RNA Vaccines: The success of mRNA vaccines during the COVID-19 pandemic has stimulated interest in further RNA technology. Innovations surrounding uracil stability and modification could lead to more effective vaccines against other pathogens.
- Synthetic Biology: The field aims to design new biological parts, devices, and systems using synthetic RNA. Understanding uracil’s properties may significantly enhance synthesis methods and overall efficacy of engineered biological systems.
The integration of uracil-focused research within modern technologies not only reveals its biological significance but also highlights its potential in creating solutions for some of the most pressing health issues today.
As researchers continue to probe the intricacies of uracil pairing and its implications in RNA structure and function, the future of RNA research promises to be both exciting and transformative.
End: The Importance of Uracil in RNA Biology
The concluding section of this article focuses on the significance of uracil in RNA biology. Understanding uracil's role does not merely contribute to a deeper comprehension of nucleobase pairing but also illuminates its broader implications in cellular processes.
Uracil stands out among nucleobases for its unique pairing properties and its role in transcription and translation. Its pairing with adenine exemplifies the intricate mechanisms driving genetic expression. This pairing is important, as it contributes to the stability and functionality of RNA molecules.
Recaps of Key Insights
In summary, several critical insights about uracil emerge from this exploration:
- Chemical Characteristics: Uracil's structure is similar to thymine but lacks a methyl group. This difference affects how uracil interacts in RNA versus thymine in DNA.
- Pairing Mechanisms: The Watson-Crick base pairing principles apply to uracil, emphasizing its compatibility with adenine. This is essential for correct RNA functioning.
- Biochemical Relevance: Uracil plays a vital role in the stability of RNA molecules, impacting RNA folding and its interaction with proteins.
- Transcription and Translation: As a key player in transcription, uracil helps facilitate the process that ultimately leads to protein synthesis, underscoring its necessity in life-sustaining functions.
The Broader Impact of Understanding Uracil
Understanding uracil's role extends beyond basic biochemistry. Its implications are profound in various scientific fields:
- Viral Research: Insights into uracil can provide critical knowledge in the study of RNA viruses, potentially guiding therapeutic strategies to combat viral infections.
- Genetic Engineering: Advances in RNA manipulation may leverage uracil's properties for enhancements in genetic engineering, possibly leading to innovations in gene therapy.
- Educational Value: For students and researchers, grasping the complex interactions of uracil enriches their understanding of molecular biology, important for advancements in related disciplines.
"A thorough understanding of uracil empowers researchers to innovate beyond conventional boundaries in genetics and virology."