Understanding Locked Nucleic Acid: An In-Depth Analysis
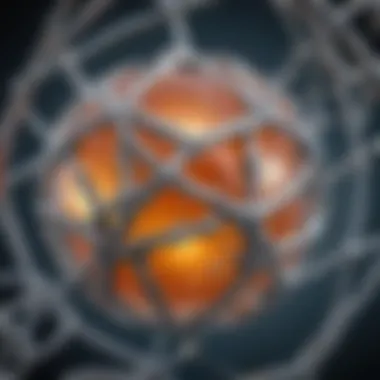
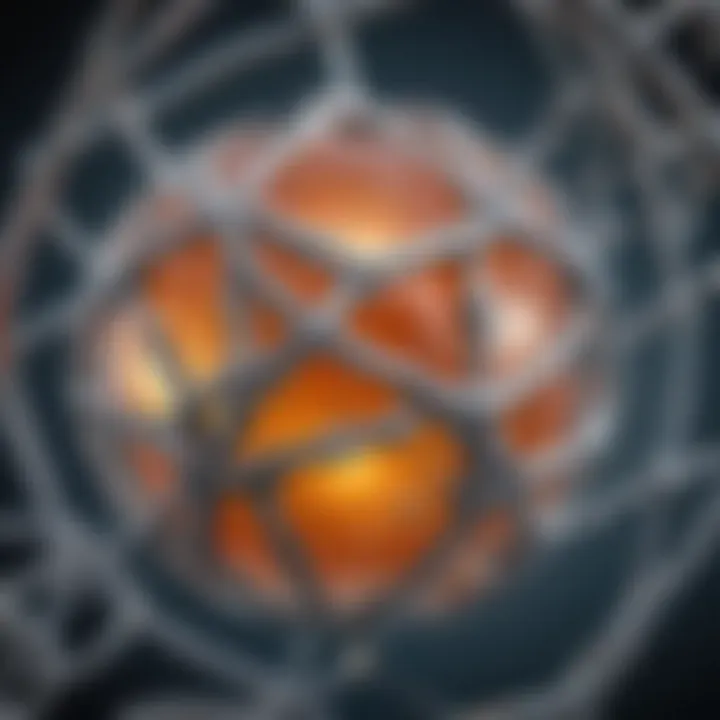
Intro
Locked Nucleic Acids (LNAs) have emerged as a pivotal innovation in molecular biology. These chemically modified RNA analogs offer unique structural advantages, which enhance their binding affinity for complementary nucleic acids. The significance of LNAs lies not only in their stability but also in their diverse applications across various scientific domains. The subsequent sections will provide a thorough exploration of LNAs, focusing on their characteristics, mechanisms, and real-world applications. This overview aims to equip students, researchers, and professionals with a deeper understanding of the integral role LNAs play in advancing modern scientific inquiry.
Research Overview
Summary of Key Findings
Recent research highlights that LNAs afford improved hybridization characteristics compared to conventional nucleic acids. They are designed to minimize target degradation and improve specificity. A growing body of literature underscores their utility in gene regulation, allowing for precise modulation of gene expression. The remarkable attribute of LNAs is their ability to function in various biological contexts, making them vital tools in both research and therapeutic applications.
Relevance to Current Scientific Discussions
LNAs are increasingly being regarded as essential components in the development of targeted genetic therapies. Their applicability spans across diagnostics, where they serve as probes for detecting specific nucleic acid sequences, to their role in enhancing the efficacy of oligonucleotide-based therapeutics. These advancements resonate with ongoing discussions on gene editing and RNA interference technologies. Understanding the mechanism and potential of LNAs can thus enrich the discourse within the scientific community, particularly in fields related to genomics and molecular medicine.
Methodology
Research Design and Approach
A systematic approach towards studying LNAs often includes a combination of biochemical assays and molecular modeling techniques. Various experimental designs enable researchers to investigate the binding properties of LNAs to their targets. This research typically involves the synthesis of LNA oligonucleotides and the analysis of their interaction with complementary DNA or RNA. The data collected aids in assessing the strength and stability of these interactions.
Data Collection and Analysis Techniques
Data collection methodologies may involve both quantitative and qualitative assessments. Techniques such as fluorescence resonance energy transfer (FRET) and gel electrophoresis are common in this field. Such methods provide insights into binding affinities and specificities. Analysis of such data often includes statistical methods to ensure reliability and reproducibility, further validating the applications of LNAs.
LNAs represent a significant advancement in molecular biology, with profound implications in gene regulation, diagnostics, and therapeutic applications.
The continuing exploration of LNAs' potential opens exciting avenues for scientific innovations, supporting their role as critical tools in contemporary research.
Prelude to Locked Nucleic Acid
The exploration of Locked Nucleic Acids (LNAs) is vital in the field of molecular biology. As a relatively new class of nucleic acid analogs, they have the potential to revolutionize various applications, including diagnostics and therapeutics. Understanding their unique structure and characteristics provides insights into their capability to enhance gene regulation, improve binding affinity, and stabilize entities against nucleases.
Definition of Locked Nucleic Acid
Locked Nucleic Acid refers to a modified nucleic acid structure where a methylene bridge connects the 2'-O and the 4'-C of the ribose sugar. This modification locks the sugar in a certain conformation, reducing the flexibility typically found in RNA and DNA. This rigidity enhances the stability of the nucleotide, allowing LNAs to bind more efficiently to complementary nucleic acids. The resulting improvement in hybridization properties is a valuable aspect of LNAs, which positions them as superior tools in various molecular biology applications.
Historical Context
The inception of Locked Nucleic Acids can be traced back to early research in nucleic acid chemistry. Initially developed in the late 1990s, LNAs were designed to overcome the limitations of traditional nucleic acids in terms of stability and binding affinity. Researchers recognized the potential of these modifications and conducted extensive studies that demonstrated their unique properties. Over the years, the field has evolved, leading to an increasing number of applications in diagnostics, gene regulation, and therapeutics. Key milestones in LNA research have paved the way for their integration into modern molecular biology, prompting further advancements and innovations.
Chemical Structure of Locked Nucleic Acid
Understanding the chemical structure of Locked Nucleic Acid (LNA) is essential due to its implications for molecular biology and its applications in research. The unique structural modifications of LNA grant it distinct properties that enhance its stability, specificity, and binding affinity towards complementary nucleic acids. This section delves into the key aspects related to the chemical structure, highlighting its relevance and impact.
Structural Modifications
Locked Nucleic Acids are characterized by specific chemical modifications that set them apart from standard RNA or DNA molecules. The core feature of LNA is the presence of a methylene bridge connecting the 2'-O and 4'-C of the ribose sugar. This modification results in a locked conformation of the ribose moiety, which imposes a constraint on the sugar pucker. Consequently, this structure enhances the overall stability of the LNA strand.
These modifications lead to important properties such as:
- Increased Thermal Stability: LNAs exhibit enhanced thermal stability compared to unmodified oligonucleotides, making them reliable for various experimental conditions.
- Improved Binding Affinity: Because of their unique structure, LNAs can form stronger hybrid complexes with complementary RNA or DNA strands. This characteristic is pivotal when designing probes for hybridization assays.
- Resistance to Nuclease Degradation: The structural modifications of LNA make it more resistant to enzymatic degradation, allowing them to function effectively in biological systems.
Understanding these structural elements is crucial for researchers interested in designing oligonucleotides that leverage the benefits of LNAs in diverse biotechnological applications.
Comparative Analysis with RNA and DNA
When comparing LNA with traditional nucleic acids such as RNA and DNA, several distinctions emerge that illustrate the advantages of LNAs in nucleic acid research.
- Base Pairing: LNAs can pair with both RNA and DNA sequences, often at a higher efficiency compared to standard RNA or DNA. They exhibit greater selectivity in hybridization due to the stronger base pairing interaction.
- Hybridization Kinetics: The kinetics of hybridization for LNA/DNA or LNA/RNA complexes is typically faster than for the corresponding RNA or DNA pairs. This feature allows for effective and rapid detection in molecular diagnostics.
- Thermodynamics: The stability of LNA constructs compared to RNA or DNA affects their thermodynamic properties. LNAs possess enhanced binding energies, making them preferable in high-specificity applications where precise recognition is required.
In summary, the chemical structure of Locked Nucleic Acid plays a critical role in its functionality and effectiveness in molecular applications. The specific structural modifications and their comparative advantages over other nucleic acid forms allow LNAs to stand out as valuable tools in gene regulation, diagnostics, and therapeutic strategies.
Mechanisms of Action
The mechanisms of action of Locked Nucleic Acids (LNAs) are critical to understanding their unique role in molecular biology. LNAs, owing to their structural modifications, exhibit distinct hybridization capabilities and heightened stability compared to conventional nucleic acids. These qualities allow LNAs to effectively engage with complementary RNA and DNA sequences. In light of their importance in various applications ranging from gene regulation to diagnostics, delving into these mechanisms is essential.
Hybridization Properties
Locked Nucleic Acids possess superior hybridization properties. The locked structure results from a methylene bridge connecting the 2' oxygen and the 4' carbon of the ribose sugar, which consequently stabilizes the sugar-phosphate backbone. This stabilization leads to a higher melting temperature of LNA-DNA or LNA-RNA duplexes when compared to standard DNA or RNA duplexes. This results in more stable binding, allowing for effective target recognition.
One notable advantage of LNAs is their ability to bind tightly to complementary nucleic acids even at low concentrations. The increased affinity not only improves the sensitivity of detection assays but also facilitates the precise regulation of gene expression. As a result, LNAs have found utility in various analytical applications and have become invaluable as molecular probes, especially in the context of real-time quantitative PCR assays.
"Locked Nucleic Acids stand out due to their remarkable ability to hybridize under conditions where traditional methods fail to yield results."
The increased affinity stems from the conformational flexibility in the LNA structure, allowing it to adjust to variable binding contexts. Thus, hybridization behavior is intrinsically linked to the efficacy of LNAs in targeting specific RNA sequences, making them valuable in both research and clinical settings.
Stability and Affinity
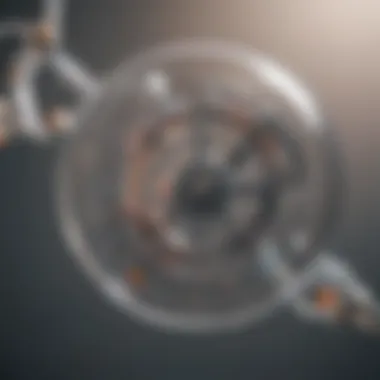
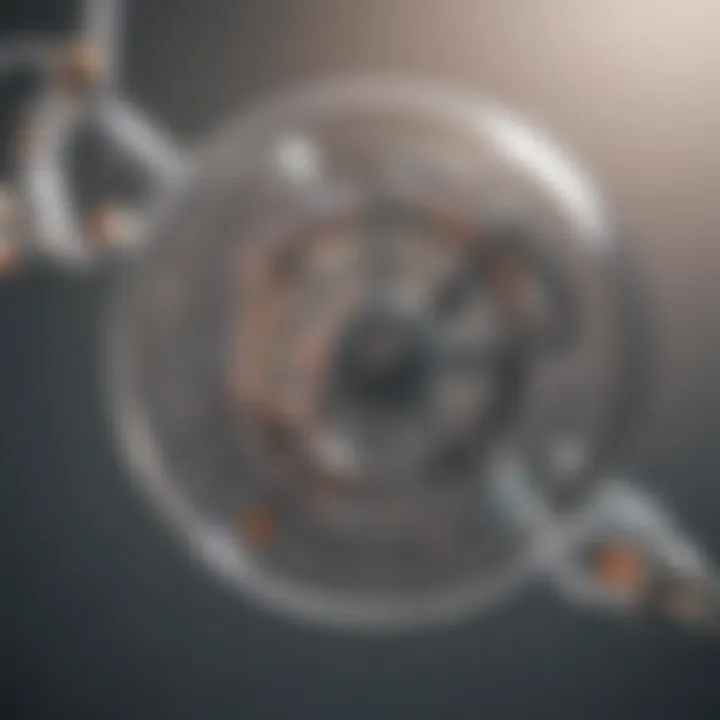
The stability and affinity of Locked Nucleic Acids play a central role in their effectiveness. LNAs, due to their unique design, present considerable resistance to nucleolytic degradation. This property is particularly salient when LNAs are utilized in therapeutic applications where prolonged activity is required. Their stability ensures that even in biological fluids where degradation rates are typically high, LNAs retain their functional integrity longer than standard oligonucleotides.
In addition to physical stability, the thermodynamic stability of LNA-modified oligonucleotides ensures that they maintain their binding properties under physiological conditions. This resilience is significant because it allows for specific interactions with target RNA or DNA without rapid dissociation, which could compromise experimental outcomes or therapeutic effectiveness.
The increased binding affinity translates into decreased dosage requirements in applications, making LNAs economically viable as well. Furthermore, their stability makes LNAs suitable for long-term storage, reducing the need for complex storage conditions often associated with traditional nucleic acid probes.
Synthesis of Locked Nucleic Acid
The synthesis of Locked Nucleic Acids (LNAs) plays a vital role in maximizing their potential in research and applications. As LNAs possess unique structural properties, the methods employed to synthesize them critically influence their functionality. Understanding these techniques is essential for researchers aiming to leverage LNAs in molecular biology. This synthesis can take various forms, each with its own advantages and constraints, making it important to choose appropriate methods for specific applications.
Chemical Synthesis Techniques
Chemical synthesis techniques have been the forefront in producing LNAs. These methods generally involve solid-phase synthesis and phosphoramidite chemistry. Solid-phase synthesis allows for the automated assembly of nucleotide sequences, facilitating the generation of complex oligonucleotides with high purity.
One significant advantage is the flexibility in designing LNAs. Researchers can introduce modifications that enhance binding affinity and stability. Moreover, the synthesis is relatively rapid and can be scaled for larger quantities, which is critical for extensive studies.
However, some challenges exist. The step-wise synthesis can lead to incomplete products or sequence errors, thus affecting the consistency of the final LNA product. Nevertheless, advances in synthesis technology continue to improve the reliability of these methods.
Enzymatic Approaches
On the other hand, enzymatic approaches for synthesizing LNAs offer a different set of benefits. These methods typically involve using enzymes, such as DNA and RNA polymerases, to facilitate the incorporation of LNA monomers into growing nucleic acid chains. Enzymatic synthesis tends to have fewer errors in nucleotide incorporation compared to chemical methods.
The benefits of enzymatic approaches are notable, especially in contexts where high fidelity is crucial. Enzymatic systems can also enable more complex reactions, leading to variations in LNA structures that may not be achievable through conventional chemical synthesis.
Despite these advantages, the enzymatic processes can be limited by the availability of suitable monomers and enzyme efficiency. In some cases, the yield may not be as high as that of chemical methods, making it crucial to balance between efficiency and accuracy based on the research needs.
The synthesis techniques for Locked Nucleic Acids not only determine their quality but also define their applicability across various scientific fields.
In summary, both chemical and enzymatic synthesis techniques play important roles in the development of LNAs, and understanding each method's strengths and limitations is paramount for researchers wanting to exploit these powerful molecules in their studies.
Applications in Molecular Biology
Locked Nucleic Acids (LNAs) have emerged as a transformative tool in molecular biology, largely due to their ability to modulate gene expression and enhance the specificity of molecular probes. Their unique properties make them suitable for various applications, particularly in gene regulation and diagnostic processes.
LNAs are known for their high binding affinity and stability when hybridized to complementary nucleic acid sequences. These features not only amplify the effectiveness of molecular assays but also reduce the likelihood of non-specific binding. As such, LNAs represent a significant advancement in the field, allowing researchers to conduct more precise studies.
Gene Regulation
Gene regulation is a fundamental process in biology. LNAs play a pivotal role in this area by enabling targeted gene silencing through antisense mechanisms. By designing LNAs that are complementary to specific mRNA sequences, researchers can effectively reduce the expression levels of genes. This technique is particularly valuable when studying gene function or validating targets for drug development.
The process works through hybridization, where the LNA binds to the mRNA, leading to its degradation or blocking its translation. The high stability of LNAs in biological systems allows for effective and long-lasting effects in gene silencing. This quality can also minimize off-target effects compared to traditional RNA interference techniques.
Moreover, LNAs can also be utilized to enhance the efficacy of small interfering RNAs (siRNAs). By modifying siRNAs with LNA at key positions, it can improve their specificity and resistance to nuclease degradation. Such enhancements amplify the potential of gene regulation strategies in therapeutic applications.
Molecular Probes
Molecular probes are essential for the detection and analysis of nucleic acids. LNAs are increasingly used as molecular probes due to their superior binding characteristics. Their ability to form stable duplexes even with mismatched or partially complementary targets enhances their utility in diagnostic applications.
The high affinity of LNAs for target sequences allows for the development of highly sensitive assays. This is particularly useful in applications such as real-time PCR and in situ hybridization. The incorporation of LNAs into probes can lead to increased signal-to-noise ratios, enabling the detection of low-abundance targets.
Additionally, the stability of LNAs under varying conditions further improves their reliability as molecular probes. This stability allows them to perform well in harsh conditions which might denature traditional nucleic acid probes.
In summary, LNAs have solidified their place in molecular biology through their applications in gene regulation and as effective molecular probes. Their unique characteristics facilitate advancements in research and therapeutic applications.
LNAs are crucial for advancing genetic research and diagnostics. As researchers continue to explore their potential, the contributions to molecular biology promise to expand. With ongoing innovations, LNAs are likely to play an even more critical role in future scientific breakthroughs.
For further details about LNAs and their applications, consider reviewing resources on Wikipedia and Britannica.
Impact on Diagnostics
The role of Locked Nucleic Acids (LNAs) in diagnostics cannot be overstated. Their unique structural properties enhance the sensitivity and specificity of assays that are critical in detecting various conditions. As molecular biology advances, the demand for precise and reliable diagnostic tools has increased. LNAs facilitate this through their ability to bind tightly to complementary nucleic acids, making them invaluable in identifying genetic variations associated with diseases.
Diagnostic Tools Utilizing LNAs
Various tools leverage LNAs for diagnostic purposes. For example, LNA-based probes are widely used in polymerase chain reaction (PCR) applications. These probes increase the reaction's efficiency, improving multiplexing capabilities, which allows for simultaneous detection of multiple targets. Additionally, LNAs are incorporated into quantitative reverse transcription PCR (qRT-PCR), where they enhance the quantification of RNA transcripts, aiding in gene expression studies.
Key features of LNA-based diagnostic tools include:
- Improved Specificity: LNAs offer higher binding affinity leading to reduced cross-reactivity with non-target sequences.
- Increased Sensitivity: Their stable interactions with complementary strands result in improved signal detection, essential in low-abundance targets.
- Robustness: These tools show resilience against degradation in biological samples, which is a common challenge in conventional nucleic acid approaches.
The application of LNAs in diagnostic kits is prevalent. Tests like the LNA-based PCR assays have shown to improve accuracy in detecting mutations in genetic disorders like cystic fibrosis and certain cancers.
Detection of Pathogens
The ability to detect pathogens accurately is crucial for disease management. LNA technology has transformed pathogen detection, allowing for rapid and sensitive identification of various microorganisms. LNAs can target specific segments of pathogen nucleic acids, significantly decreasing the time needed for diagnosis.
LNA-based assays have been utilized effectively in detecting viral pathogens, including SARS-CoV-2. This has implications not only in clinical laboratories but also in public health initiatives aimed at controlling outbreaks. Furthermore, they can also assist in the monitoring of antibiotic resistance, which is a growing concern in infectious disease management.
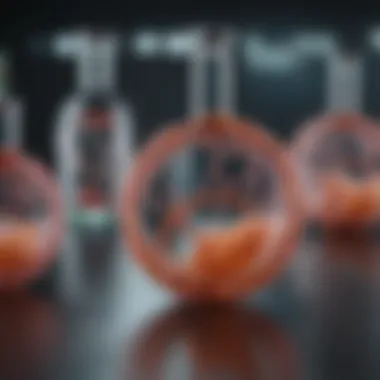
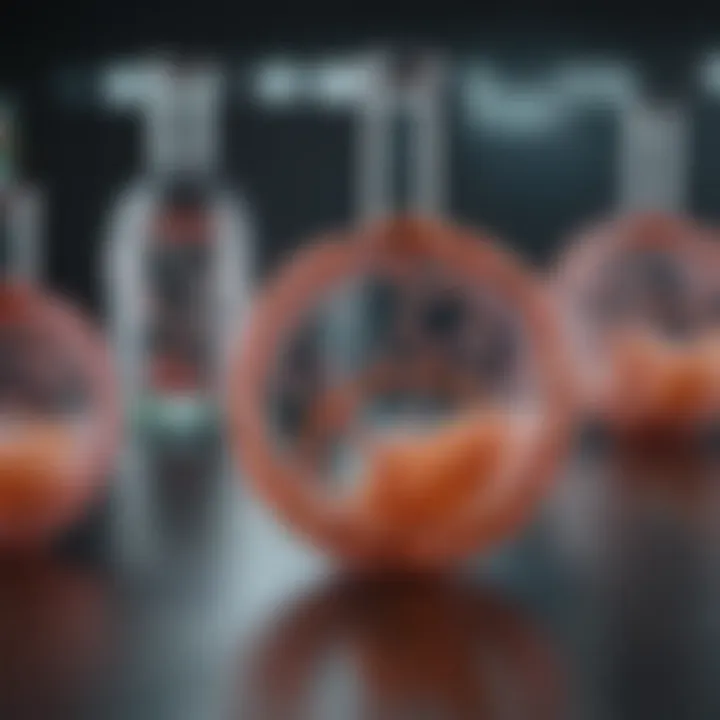
"The integration of LNA technology in diagnostic processes marks a pivotal advancement in our capacity to detect and respond to infectious diseases efficiently."
In summary, the impact of Locked Nucleic Acids on diagnostics is profound, with their ability to create precise and reliable tools that cater to the evolving requirements of modern medicine. Their usage in diagnostic tools enhances specificity and sensitivity, addressing important challenges that researchers and clinicians face daily.
Therapeutic Potential of Locked Nucleic Acid
Locked Nucleic Acids (LNAs) possess significant therapeutic potential. Their unique structure enhances their ability to bind to complementary RNA sequences with high specificity and stability. This attribute makes them valuable tools in targeted therapies, particularly in areas such as antisense therapeutics and cancer treatment. The increasing interest in LNAs stems not only from their binding properties but also from their ability to influence gene expression and modulate cellular pathways.
The importance of LNAs in therapeutic applications can be summarized into several key points:
- Increased Binding Affinity: The locked structure provides stronger and more stable binding to complementary nucleic acids, improving their efficacy in therapeutic scenarios.
- Diverse Applications: LNAs can be utilized in gene therapy, cancer treatment, and the development of molecular probes, broadening their therapeutic scope.
- Reduced Off-Target Effects: Due to their high specificity, LNAs are less likely to interact with non-target nucleic acids, minimizing potential side effects.
Overall, the therapeutic potential of LNAs offers promising avenues for advanced medical treatments, making them an essential topic in contemporary molecular biology.
Antisense Therapeutics
Antisense therapy involves the use of synthetic oligonucleotides that bind to specific mRNA molecules. The aim is to inhibit the expression of undesired genes, a process vital in treating various genetic disorders and diseases. LNAs, with their enhanced stability and affinity, serve as potent antisense agents. They can effectively block mRNA translation or promote degradation by cellular mechanisms.
Several factors contribute to the effectiveness of LNAs in antisense therapeutics:
- Thermodynamic Stability: LNAs provide better thermodynamic stability than traditional antisense oligonucleotides. This stability results in prolonged interactions with target mRNA.
- Improved Cellular Uptake: Modified properties of LNAs allow for better cellular uptake, which is critical for achieving therapeutic concentrations within target cells.
- Versatile Delivery Mechanisms: There are various delivery systems including liposomes and nanoparticles that can be adopted to enhance LNA delivery, ensuring they reach the desired sites within the body.
Research in this area has shown promising results, and LNAs are being investigated for conditions like Duchenne Muscular Dystrophy and Huntington’s disease.
Cancer Treatment Applications
The role of LNAs in cancer treatment is gaining traction as a significant field of research. Cancer cells often exhibit dysregulation of gene expression that contributes to their uncontrolled growth. By designing LNAs to selectively target oncogenes or downregulate tumor suppressor genes, researchers aim to intervene in these processes.
Key aspects to consider regarding LNAs in cancer therapies include:
- Targeted Gene Silencing: LNAs can effectively silence genes responsible for tumor growth, thereby slowing down or even reversing cancer progression.
- Combination Therapies: Utilizing LNAs in conjunction with chemotherapy or immunotherapy may enhance treatment effectiveness. By targeting specific cancer-related genes, LNAs can sensitize tumors to existing treatments.
- Personalized Medicine: LNAs provide opportunities for developing personalized therapies based on genetic profiles of tumors, resulting in more efficient and tailored treatment strategies.
In clinical trials, LNAs have shown potential in treating various types of cancer, including breast, prostate, and lung cancers. Continued innovation and research are essential to unlock their full therapeutic potential, paving the way for new treatments that could dramatically improve patient outcomes.
Limitations and Challenges
This section addresses the inherent limitations and challenges associated with Locked Nucleic Acids (LNAs). While LNAs have significantly impacted molecular biology, understanding their limitations also helps refine their applications and enhance their effectiveness. Awareness of these challenges is crucial for students, researchers, and practitioners in the field, as it shapes future research directions and refinement strategies.
Stability Issues in Biological Systems
Stability in biological systems is a major concern when utilizing Locked Nucleic Acids. The biological environment is inherently dynamic, and nucleic acids are prone to degradation by enzymes such as nucleases. LNAs, despite their enhanced stability compared to conventional RNA, can still face challenges in vivo.
Factors contributing to their instability include:
- Enzymatic Attack: LNAs can be recognized by specific nucleases, which may lead to unintended degradation, reducing their efficacy in experimental and therapeutic settings.
- Cellular Conditions: The intracellular environment is complex, with varying pH and temperature levels that can impact LNA stability.
- Chemical Modifications: Some modifications intended to enhance binding affinity could affect stability, leading to a compromise between binding efficiency and resilience in biological systems.
Understanding these stability issues allows researchers to develop strategies to mitigate degradation risks, such as adopting protective chemical modifications or targeting specific cellular compartments.
Delivery Mechanisms
Another significant challenge in the application of Locked Nucleic Acids is effective delivery mechanisms. For LNAs to exert their therapeutic or functional roles, they must efficiently reach the target cells or tissues.
The key considerations for improving delivery mechanisms include:
- Cell Membrane Penetration: LNAs are polar molecules, which can hinder their ability to cross cellular membranes. Lipid-based delivery systems or conjugation with cell-penetrating peptides have been explored to enhance cellular uptake.
- Targeted Delivery: The development of targeted delivery systems is vital. Utilizing ligands that specifically bind to receptors on target cells can increase the localization of LNAs to specific tissues, improving their therapeutic potential.
- Formulation Factors: The formulation of LNA therapeutics must consider factors such as stability during storage, compatibility with delivery vehicles, and release kinetics once delivered to the target site.
Recent Innovations in Locked Nucleic Acid Research
Recent innovations in Locked Nucleic Acid (LNA) research mark significant milestones that enhance both our understanding and application of these versatile molecules. As scientific inquiry evolves, the exploration of LNAs has opened new frontiers in molecular biology, diagnostics, and therapeutics. Innovations have improved the efficacy and specificity of LNAs, magnifying their potential impact. They possess unique structural characteristics that confer remarkable stability and an increased binding affinity when compared to traditional nucleic acids.
Emerging research focuses on refining the synthesis of LNAs, exploring novel delivery systems, and integrating LNAs with other advanced technologies. These developments not only improve the functionality of existing applications but also expand the framework for new uses. For instance, innovations in chemical synthesis techniques are enabling the production of LNAs with tailored properties, enhancing their role in precision medicine and targeted therapies.
Moreover, a deeper understanding of LNA interactions at the molecular level is paving the way for more sophisticated applications in molecular diagnostics. The ability to design LNA-based probes for specific target sequences has implications for the early detection of diseases. As we navigate innovations in LNA research, key considerations such as production costs, scalability, and regulatory hurdles demand attention, ensuring sustainable progress in this field.
Emerging Techniques
Emerging techniques in Locked Nucleic Acid research illustrate the innovative spirit driving the field forward. Recent advancements in synthesis methods provide greater control over LNA characteristics, enabling the design of molecules that meet specific needs. For example, advancements in solid-phase synthesis techniques allow for the rapid and efficient production of LNA oligomers. This not only accelerates research but also enhances the reproducibility of results, which is critical in scientific studies.
Researchers are also exploring novel strategies for enhancing LNA stability. Techniques such as the incorporation of modified nucleotides can improve resistance to enzymatic degradation. This feature is crucial for applications in vivo, where the biomolecules are exposed to various biological processes.
In addition, the integration of LNAs with various chemistries, like click chemistry, allows for the attachment of functional groups that can facilitate cellular uptake. The combination of these emerging techniques helps to overcome the limitations of previous generations of nucleic acid analogs and sets the stage for greater utility in clinical applications.
Novel Applications
The novel applications of Locked Nucleic Acids reflect their growing significance in several scientific domains. One promising area is personalized medicine, where LNAs are being developed as tools for targeted gene editing and regulation. By employing LNAs, researchers can selectively silence or activate gene expression, thereby developing tailored therapies for complex diseases.
In diagnostics, LNAs are being utilized in the development of advanced molecular probes. These probes can detect low-abundance target sequences in various sample types, making them invaluable for early disease detection. Their high specificity reduces the likelihood of false positives, which is crucial in clinical settings.
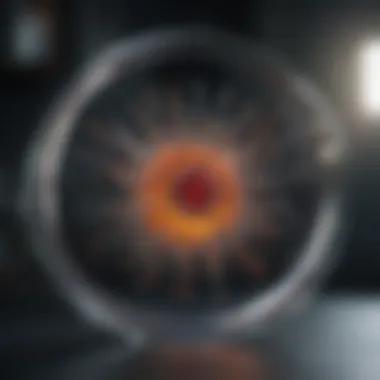
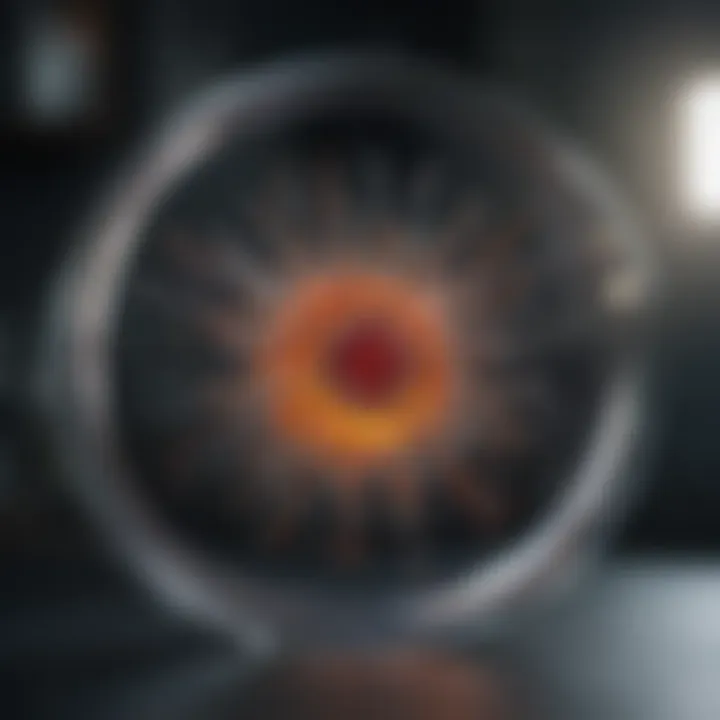
Additionally, there is growing interest in using LNAs in the realm of bioconjugation. By attaching LNAs to nanoparticles, researchers can create highly sensitive diagnostic assays. This method leverages the unique binding properties of LNAs, allowing for enhanced sensitivity and specificity in detecting pathogens or biomarkers.
As the landscape of Locked Nucleic Acid research continues to expand, it is essential to monitor these innovations closely. Understanding the newest techniques and applications will provide deeper insight into how LNAs will shape the future of molecular biology and medicine.
Comparison with Other Nucleic Acid Analogs
Locked Nucleic Acids (LNAs) hold a notable position within the landscape of nucleic acid analogs. Their capacity to enhance hybridization and stability of nucleic acid structures positions them as a crucial tool in molecular biology. Understanding how LNAs compare with other nucleic acid analogs like Peptide Nucleic Acids (PNAs) and Morpholino Oligomers helps in grasping their unique advantages and limitations.
Peptide Nucleic Acid (PNA)
Peptide Nucleic Acids are synthetic polymers that closely mimic DNA or RNA. Unlike normal nucleic acids, PNAs have a backbone made of peptide-like bonds. This structural difference grants PNAs remarkable binding affinity for complementary nucleic acids. They enable stronger hybridization due to their neutral charge and resistance to nucleases, making them promising for various applications.
PNAs offer several advantages:
- High Affinity: They bind effectively to DNA and RNA, often better than DNA probes.
- Stability: PNAs are stable in biological fluids, which is a significant factor in therapeutic settings.
However, there are limitations. PNAs do not readily cross cellular membranes, restricting their application in vivo. Therefore, while they are effective hybridization tools, their utility can be limited in real biological contexts compared to LNAs, which have a higher cellular uptake due to their modified structure.
Morpholino Oligomers
Morpholinos are another class of nucleic acid analogs, characterized by a morpholine ring in their backbone. This structure sets them apart from typical nucleic acids, allowing them to enhance stability against nucleases and increasing the overall lifespan of the molecules in biological systems.
The benefits of Morpholino Oligomers include:
- Resistance to Nucleases: This makes Morpholinos long-lasting in the body.
- Targeted Gene Silencing: They can effectively block specific mRNA from translating into proteins, which is beneficial in gene regulation studies.
Nonetheless, Morpholino Oligomers also face challenges similar to PNAs. They require specific delivery methods to enter cells effectively. In contrast, LNAs have been developed to have higher affinity for their target sequences, often silencing genes more efficiently and demonstrating significant therapeutic potential.
In summary, while PNAs and Morpholino Oligomers offer unique strengths, their limitations in delivery and cellular uptake position LNAs as powerful alternatives in modern research and therapies. Understanding these comparisons enhances the overall insight into the advancements in the field of nucleic acids.
Future Directions in Research
Research on Locked Nucleic Acids (LNAs) is key to appreciating their role in modern molecular biology. As scientists investigate the complex interplay between LNAs and biological systems, potential applications continue to expand. This section will explore how LNAs are anticipated to shape the future of genetic and therapeutic research.
Potential for Increased Applications
The versatility of locked nucleic acids holds promise for a broad array of applications. One significant area is in gene therapy. LNAs enhance the stability of oligonucleotides, allowing for more effective targeting of genes implicated in diseases. This could facilitate tailored treatments for genetic disorders, potentially transforming how we approach these conditions.
In diagnostics, LNAs are being leveraged to create more sensitive assays. Their high binding affinity can improve detection rates of low-abundance nucleic acids. Such advancements might lead to earlier diagnoses and more personalized medicine.
Additionally, LNAs can further impact RNA interference technologies. Their ability to inhibit gene expression in a precise manner allows researchers to explore new routes for downregulating unwanted protein production. This has implications for areas like cancer treatment and viral infections.
Integration with Other Technologies
As research progresses, the integration of LNAs with emerging technologies seems inevitable. One avenue is their combination with CRISPR technologies. By merging the specificity of LNAs with CRISPR’s gene-editing capabilities, it may be possible to enhance gene-targeting accuracy. This collaboration might yield new approaches to address genetic diseases more effectively.
Moreover, advances in nanotechnology could provide innovative delivery methods for LNA-based treatments. Encapsulating LNAs in nanoparticles could protect them from degradation, ensuring they reach their target in biological systems. This strategy may enhance both the efficacy and safety profiles of LNA therapeutics.
LNAs also have potential in biomarker discovery when paired with high-throughput sequencing technologies. The robust nature of LNAs could allow for more reliable identification of biomarkers associated with diseases, leading to improved understanding and diagnostics.
Ethical Considerations
The exploration and application of Locked Nucleic Acids (LNAs) bring forth several ethical considerations that merit careful examination. As molecular biology advances, the manipulation of genetic material raises questions about ethical practices in research, potential consequences, and societal impacts. Understanding these considerations not only enhances our grasp of LNAs but also frames their use within a responsible scientific context.
Ethics in Genetic Research
In genetic research, ethical concerns revolve around issues such as consent, the potential for genetic discrimination, and the long-term effects of genetic modifications. Researchers utilizing LNAs must prioritize transparency and obtain informed consent from participants when human subjects are involved. This necessity is particularly critical when the work affects genetic information that could impact individuals' lives. Genetic discrimination based on findings related to LNAs could lead to unjust treatment in areas like employment or insurance. Ethical guidelines must be in place to prevent misuse.
Moreover, there is a continuous debate about the extent of genetic editing and what implications it holds for future generations. The introduction of LNAs might offer exciting possibilities in gene regulation and therapy, yet they also put into question the morality of modifying genetic codes. Researchers need to engage in discussions around these ramifications both within the research community and with the public.
Impact on Society and Regulations
The impact of LNAs on society can be profound, necessitating a framework of regulations to safeguard the ethical use of this technology. Governments and institutions must establish regulatory standards that guide research, ensuring that innovations in LNAs are not only scientifically valid but also socially responsible. Proper regulations can mitigate risks associated with genetic engineering, such as unintended consequences that may arise from altering genetic material.
A collaborative approach involving scientists, ethicists, regulators, and the public is essential to navigate the complexities involved. Policies must adapt to technological advancements in genetic research, keeping pace with developments in LNAs and their applications.
"Ethics is a process of reasoning to determine the best possible outcomes for individuals and society. In genetic research, this means ensuring that new technologies benefit humanity without compromising ethical standards."
In the pursuit of scientific knowledge, it is crucial to anchor practices in ethical principles, balancing innovation with respect for individual rights and societal implications.
Culmination
The conclusion of this article serves as a pivotal point for understanding the implications and potential of Locked Nucleic Acids (LNAs). It emphasizes the significant roles LNAs play in molecular biology and their broader applications in diagnostics and therapeutics. By integrating insights gathered from various sections, this conclusion synthesizes the key elements presented throughout the article.
Summary of Key Points
- Definition and Structure: LNAs are characterized by their unique locked structure, which enhances their stability and affinity for complementary nucleic acids. This structural feature allows for more robust hybridization compared to standard DNA and RNA.
- Mechanisms of Action: LNAs exhibit exceptional hybridization properties, contributing to their increased stability in biological environments. This characteristic is crucial for their effectiveness in gene regulation and as molecular probes.
- Applications: LNAs have found a place in various applications, from gene regulation to diagnostics. Their unique properties make them valuable tools in therapeutic strategies, particularly in the field of cancer treatment.
- Limitations and Challenges: Despite their advantages, LNAs face certain hurdles, such as stability issues in biological systems and challenges related to delivery mechanisms.
- Recent Innovations: Ongoing research continues to uncover emerging techniques and novel applications for LNAs, pointing toward an exciting future in molecular biology.
Implications for Future Research
The future of Locked Nucleic Acids research presents numerous opportunities. Firstly, as research expands, there may be potential for increasing the applications of LNAs in diverse fields like synthetic biology, where they could be employed to create highly specific genetic circuits. Secondly, integrating LNAs with other technologies, such as CRISPR, may enhance gene-editing efforts, leading to more precise interventions in genetic disorders.
Moreover, as diagnostic tools evolve, LNAs could play an integral role in developing more sensitive and specific detection methods for pathogens. The unique properties of LNAs suggest they could lead to breakthroughs in understanding complex biological processes, ultimately fostering advancements in treatment methodologies.
As the landscape of molecular biology progresses, the ongoing exploration of Locked Nucleic Acids will likely yield significant contributions, emphasizing their role as versatile agents in scientific research. It is essential for students, researchers, and professionals to stay abreast of these developments to fully grasp the potential of LNAs and their implications for the future of biotechnology.