Understanding Acidic Concentration: Impacts and Applications
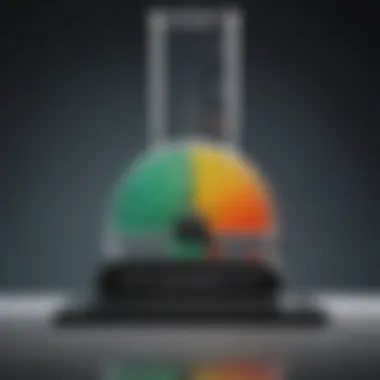
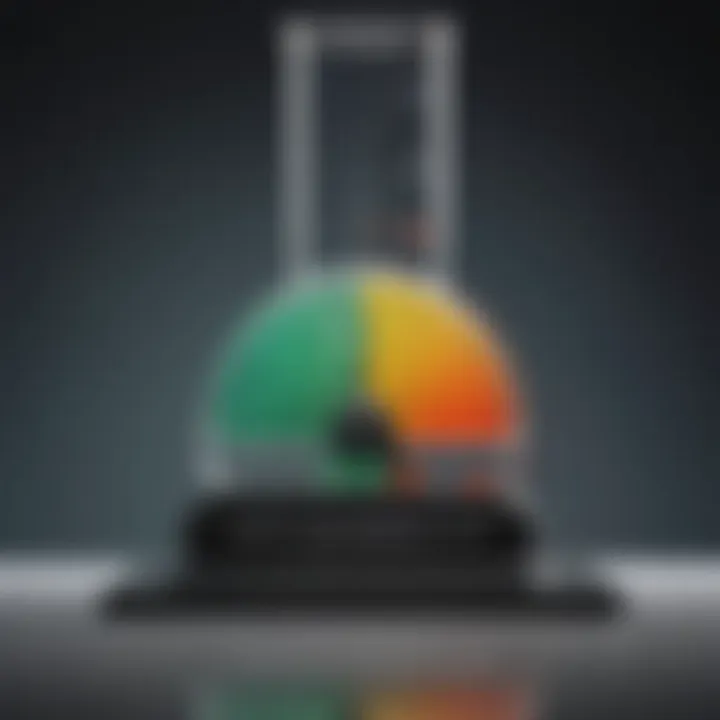
Intro
Acidic concentration is a fundamental concept that has critical implications across a range of scientific disciplines. Understanding its nature is not just about defining acidity but also about grasping how it influences chemical reactions, biological processes, and environmental stability. This article aims to offer a detailed exploration of acidic concentration, discussing its metrics, effects, and applications in various fields.
The significance of acidic concentration extends into practical realms, affecting industries such as agriculture, manufacturing, and environmental management. By comprehensively examining these aspects, we will highlight the relevance of acidity in both theoretical and applied science. This foundational knowledge is essential for students, researchers, and professionals alike, who rely on accurate communication of scientific concepts to foster innovation and address pressing global challenges.
"The understanding of acidity is not merely an academic pursuit; it affects our health, environment, and industrial practices."
As we proceed, we will dissect the research surrounding acidic concentration. This involves a deep dive into its definitions, metrics for measurement, and the varied impacts on ecosystems and living organisms. The narrative will follow with detailed assessments of how acidic conditions apply to everyday life.
In the quest to elucidate this important topic, it is crucial to remain aware of ongoing scientific discussions regarding acidity. Therefore, readers will find value in bringing this understanding into broader contexts, linking its relevance to current environmental and health issues.
The following sections will provide a research overview, elucidating key findings and methodological approaches that have shaped our understanding of acidic concentration.
Prelude to Acidic Concentration
Acidic concentration plays a fundamental role in a range of disciplines, including chemistry, biology, and environmental science. Understanding acidic concentration is not merely an academic exercise; it impacts practical applications in many aspects of daily life, from agriculture to industrial manufacturing. The article discusses why comprehending this concept is vital for both professionals and researchers. It aims to explore various dimensions of acidity: its definition, measurement, effects on biological systems, industrial applications, and its environmental implications. Each facet helps illuminate how acidic substances interact within different contexts and why they must be considered in some decision-making processes.
Definition of Acidity
Acidity refers to the capacity of a substance to donate protons (H⁺ ions) in a solution, resulting in a decrease in pH. The concept has vital implications in chemistry, particularly when discussing chemical reactions. A lower pH indicates a higher acidic concentration, which can influence the behavior of molecules and ions in a solution. Different methods exist to quantify acidity, enabling scientists and industries to apply this knowledge effectively. It is crucial to recognize various types of acids, including strong acids like hydrochloric acid and weak acids like acetic acid, each affecting ecosystems and human health differently.
Historical Context
The study of acidity has roots that extend back to ancient civilizations. The word "acid" comes from the Latin "acidus," which means "sour." Historical texts illustrate the early understanding of sour substances, such as vinegar, which was one of the first acids identified. As scientific inquiry developed during the Enlightenment, researchers like Antoine Lavoisier played a crucial role in refining the concepts of acidity and base. The advent of modern chemistry brought about rigorous theories, such as Arrhenius and Brønsted-Lowry theories, which formulated a better understanding of acid-base interactions. This historical perspective lays the groundwork for insights into the contemporary applications and implications of acidic concentration in our world today.
Theoretical Foundations of Acidic Concentration
The theoretical foundations of acidic concentration are of fundamental importance in understanding its implications across various scientific domains. These foundations provide the necessary frameworks to define acidity, measure acidic solutions, and interpret their behavior in chemical reactions. By exploring acid-base theories and the pH scale, we can better grasp the complexities surrounding acidic concentrations. This understanding is critical for advancements in chemistry, biology, and environmental science, offering insights into both theoretical concepts and practical applications.
Acid-Base Theories
Brønsted-Lowry Theory
The Brønsted-Lowry theory represents a significant advancement in acid-base chemistry. This theory defines acids as proton donors and bases as proton acceptors. This characteristic allows for a more comprehensive understanding of acid-base reactions beyond the limitations of earlier theories. The Brønsted-Lowry theory's emphasis on proton transfer supports its application in various settings, including biological systems, where protonation states can determine molecular behavior.
One unique feature of this theory is its adaptability; it can accommodate reactions that do not involve hydroxide ions, thus expanding the concept of acidity. However, this theory may not address every scenario, particularly in non-aqueous environments. Despite these disadvantages, its ability to describe a vast range of chemical processes makes it a valuable choice in this article.
Arrhenius Theory
The Arrhenius theory is another foundational concept in acid-base chemistry, defining acids as substances that increase the concentration of hydrogen ions in solution, while bases elevate hydroxide ions. This straightforward approach is highly beneficial for its clarity and directness in typical aqueous solutions.
A distinctive element of the Arrhenius theory is its historical significance; it paved the way for subsequent theories while providing a simple framework for identifying acids and bases. However, it has limitations when considering behaviors of substances in non-aqueous solutions or in reactions where water is not present. Despite its shortcomings, the Arrhenius theory remains relevant for understanding basic acid-base interactions.
Lewis Acids and Bases
The concept of Lewis acids and bases broadens the scope of acid-base theory further. Here, Lewis acids are electron pair acceptors, while Lewis bases are electron pair donors. This perspective is advantageous as it incorporates molecular and ionic interactions into a unified definition of acids and bases.
A key characteristic of the Lewis theory is its inclusivity; it captures reactions that involve covalent bonds and complex ions, expanding the traditional dialogues about acidity. However, some may find the theory abstract; it requires a clear understanding of electron behavior. Nevertheless, it contributes significantly to discussions in organometallic chemistry and catalysis, solidifying its relevance in diverse contexts.
pH Scale and Measurement
Defining pH
The pH scale is essential in quantifying the acidity or basicity of a solution. It specifically measures the concentration of hydrogen ions, presenting a logarithmic scale that ranges from 0 to 14, with lower values indicating acidic conditions. Defining pH this way simplifies discussions around acidic concentration, enabling quick assessments of chemical environments.
A key characteristic of pH is its broad applicability across various fields, from environmental science to biology, stressing the importance of understanding acidic solutions. However, users must note that pH does not capture the complete picture of a solution's nature, especially in cases involving buffered solutions or complex mixtures.
Calculating pH
Calculating pH involves logarithmic equations that link hydrogen ion concentration to pH values. This method often proves practical in laboratory settings due to its straightforward calculations. The versatility of pH calculations enhances their relevance across disciplines, allowing researchers to evaluate acidity quickly and effectively.
A notable unique feature of calculating pH is its ability to provide precise values that inform experimental decisions. However, practitioners must be cautious of dilution effects and temperature variances that can influence readings. Understanding these aspects ensures accurate assessments.
Limitations of pH Measurements
While pH measurements are vital for assessing acidity, they have certain limitations. Factors such as temperature changes, ionic strength, and the presence of interfering substances can skew results. Recognizing these limitations is crucial for scientific environments seeking reliable data.
The key characteristic of these limitations lies in their ability to affect accuracy in sensitive contexts. Thus, researchers must often complement pH measurements with additional analysis methods to gain clearer insights into solution behavior. Despite these challenges, pH remains an indispensable tool in the study of acidic concentration.
Measuring Acidic Concentration
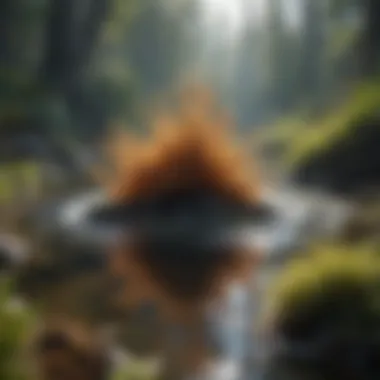
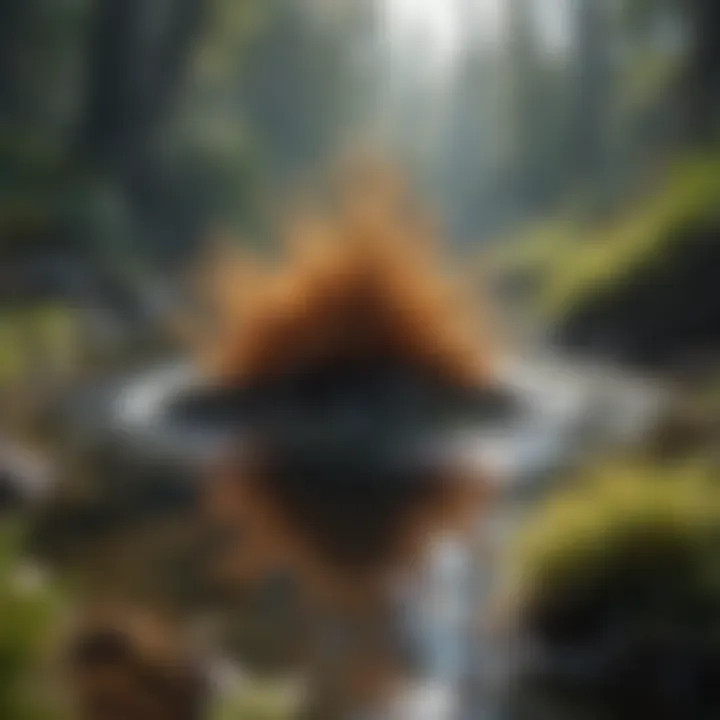
Measuring acidic concentration is a central aspect of understanding how acids interact within various systems. Accurately assessing acidity levels is crucial in numerous fields, including biology, chemistry, and environmental science. This measurement can influence experimental outcomes, industrial applications, and agricultural practices. Therefore, a solid grasp of measurement techniques can significantly impact decisions and strategies across various scientific and practical domains.
Titration Methods
Acid-Base Titration Techniques
Acid-base titration techniques are among the most commonly employed methods for measuring acidity. This involves the careful addition of a titrant to an analyte until a specific reaction endpoint is reached. One notable feature of these techniques is their ability to provide precise measurements of acidic concentration, which is critical in both laboratory and industrial settings. The practicality and ease of use make acid-base titration very popular in educational contexts as well.
With titrations, the concentration of an unknown acid can be determined by comparing it with a solution of known strength. This is particularly useful in scenarios where accurate acidity levels are required for product formulation or research activities. However, one limitation is that successful titration depends on the selection of the right indicators, as some reactions may not produce visible color changes that are easy to analyze.
Indicators Used in Titration
Indicators are chemical compounds that change color at specific pH levels, serving as a visual cue during titrations. These substances are essential for identifying the endpoint of the titration. The characteristic colour change provides immediate feedback to the experimenter, making indicators a beneficial choice in measuring acidity levels. Common indicators include phenolphthalein and bromothymol blue, each offering unique ranges of pH sensitivity.
The use of indicators streamlines the titration process, making it less reliant on equipment. However, the selection of an appropriate indicator is key, as the color change must align effectively with the pH of the solution. For instance, if the endpoint occurs outside of an indicator's effective range, results may be inaccurate.
Spectrophotometric Analysis
Spectrophotometric analysis is another valuable technique for measuring acidic concentration. This method involves assessing the absorbance of light by a solution to determine its acidity. The strength of the light absorption correlates with the concentration of acids present in the solution. This method is noted for its rapid results and high level of precision, making it ideal for standard laboratory analyses.
The technology works based on the Beer-Lambert Law, which relates absorbance to the concentration of the absorbing species. However, the technique requires careful calibration and a proper understanding of the light wavelengths that ions absorb. Skillful management of these factors is essential to obtaining reliable data.
Impacts of Acidic Concentration in Biological Systems
Understanding acidic concentration is essential in exploring its numerous impacts on biological systems. Acidic compounds play significant roles in both human physiology and broader ecosystems. This section will focus on how acidity influences human metabolic processes and cellular functions, as well as its effects in ecosystems, particularly concerning aquatic life and soil health.
Acidity in Human Physiology
Effects on Metabolism
Acidic concentration directly influences metabolic processes in the human body. Metabolism refers to the chemical reactions that maintain life, transforming food into energy. An important aspect of metabolism affected by acidity is enzymatic activity. Enzymes, which are proteins, rely on specific pH levels to function optimally. For example, the enzyme pepsin, involved in protein digestion, operates effectively in highly acidic conditions found in the stomach.
The key characteristic of this relationship is that optimal acidity enhances metabolic efficiency. When pH levels deviate from ideal ranges, metabolic rates can slow, leading to fatigue or other health issues. It is crucial in this article to emphasize that maintaining a balanced acid concentration within metabolic pathways is beneficial for overall health.
Unique features of this topic include the role of lactic acid produced during intense exercise. While it can lead to muscle fatigue, managing its concentration may also signal the body to adapt and improve metabolic performance over time. An important consideration is that prolonged deviations in acidity can indicate metabolic disorders, impacting wellbeing adversely.
Impact on Cellular Function
Cellular function is fundamentally tied to the pH of the surrounding environment. Many cellular processes, including signaling pathways and structural integrity, depend on an appropriate acidic concentration. For instance, acidic conditions within lysosomes, cellular organelles that digest waste materials, help maintain normal function and cellular health.
A notable feature of this relation is that incorrect acidity in the cellular environment can disrupt normal function, leading to conditions such as acidosis. This condition can impair cardiovascular function, leading to fatigue and decreased exercise capacity. It is important to recognize that a balanced acidic concentration keeps cellular processes functioning optimally, sustaining health.
From this perspective, the advantages of understanding how acid concentration affects cellular function encompass better management of health at the cellular level, potentially mitigating various diseases. The unique aspect is the responsiveness of cells to changes in pH. This responsiveness can guide targeted therapies aimed at restoring balance in conditions where acidity is impaired.
Acidity in Ecosystems
Effects on Aquatic Life
In ecosystems, acidity, particularly in water bodies, plays a critical role in shaping aquatic life. Acidic water can significantly impact the biodiversity, behavior, and health of aquatic organisms. For example, lower pH levels can harm fish populations, as many species are sensitive to changes in acidity. The relationship here is clear: as water becomes more acidic, some species may struggle to survive, leading to a decline in populations and biodiversity.
The unique characteristic of this discussion lies in the delicate balance of pH and its influence on nutrient availability in water. Acidity can alter the solubility of metals and minerals, impacting the overall health of aquatic environments. Understanding these dynamics is critical in conserving aquatic species and ecosystems.
However, one must also consider the adaptability of some aquatic organisms to acidic conditions. This adaptability can lead to shifts in species composition, which may have long-term ecosystem implications. It suggests that ecosystems might adjust to changes in acidity, though such changes often come with risks to less adaptable species.
Soil Health and Fertility
Acidity also profoundly affects soil health and fertility. The pH of soil influences nutrient availability to plants. At optimal acidity levels, essential nutrients such as nitrogen, phosphorus, and potassium are more accessible to roots, supporting healthy crop growth. Therefore, managing soil pH is crucial for agriculture and ecosystem sustainability.
One important characteristic of soil health affected by acidity is the activity of microorganisms. Beneficial bacteria that support plant health often thrive in specific pH levels. When soil becomes too acidic, it can inhibit these organisms, leading to reduced soil fertility and plant growth. This underscores the importance of maintaining balanced acidity, thus promoting robust ecosystems.
Additionally, while acidity can pose challenges, it also presents opportunities for soil amendment practices. Farmers can manage soil pH through the application of lime or sulfur, fostering a more suitable environment for crops. Nevertheless, one must consider the environmental impacts of modifying soil acidity, including potential runoff issues, which can affect local water bodies.
Industrial Applications of Acidic Concentration
Acidic concentration plays a vital role in various industrial processes. The significance of this topic lies in its multifaceted applications, which directly impact chemical manufacturing and the food and beverage industry. Understanding the procedures, benefits, and potential hazards related to acidic concentration enables businesses to optimize their operations and ensure compliance with safety standards.
Chemical Manufacturing
Role in Synthesis
In chemical manufacturing, acidic concentration is essential for numerous synthesis reactions. Acids serve as catalysts, helping to speed up chemical reactions that produce a range of products, from plastics to pharmaceuticals. The ability to adjust the acidic concentration can significantly influence the efficiency of these synthesis processes.
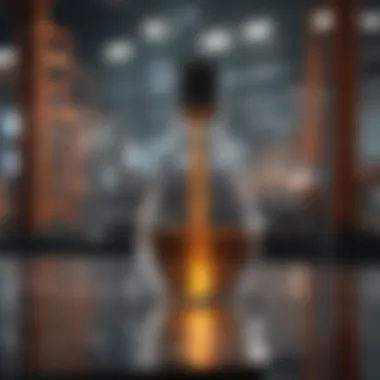
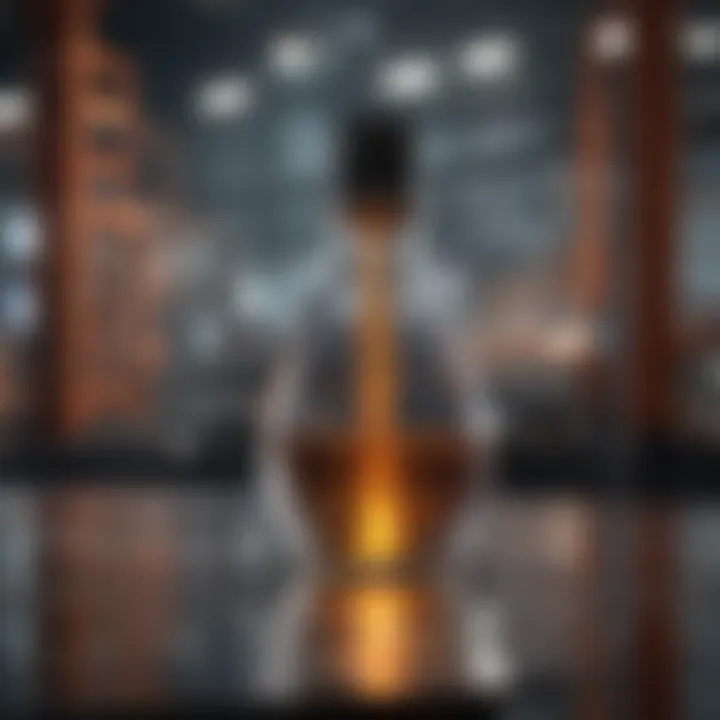
One key characteristic of using acids in synthesis is the versatility they bring. Many reactions depend heavily on precise acidic environments to achieve optimal yields. This benefits manufacturers by allowing them to fine-tune reactions, achieving a better product quality and reducing waste. However, it is crucial to control the acidic levels carefully, as excessive acidity can lead to unwanted byproducts or hazardous conditions.
Safety and Regulations
Safety and regulations surrounding acidic concentration in manufacturing are of utmost importance. The handling of acids is inherently dangerous and requires strict adherence to safety protocols. Industry regulations can include guidelines on storage, usage, and disposal of acidic substances to minimize risks associated with chemical burns and environmental damage.
A significant focus on safety is the training provided to employees. Understanding the risks and safety measures can lead to a safer work environment. Compliance with such regulations not only protects workers but also minimizes legal liabilities and environmental impacts. Non-compliance can lead to severe consequences, as regulatory bodies impose hefty fines and other penalties for breaches.
Food and Beverage Industry
Preservation Methods
In the food and beverage industry, acidic concentration is crucial for preservation methods. Acidic environments can effectively inhibit the growth of spoilage microorganisms, prolonging the shelf life of various products. For example, using acetic acid in pickling enhances preservation by lowering pH level, creating an unfavorable environment for pathogenic bacteria.
The primary benefit here is the extended shelf life of products, which reduces wastage and increases profitability. However, one drawback can be the alteration of flavor and texture, which requires careful balancing of acidity to retain the desirable qualities of the food.
Flavor Enhancement
Flavor enhancement is another aspect where acidic concentration shines in the food and beverage sector. Many beverages and foods utilize acids like citric or lactic acid to achieve a characteristic tartness that enhances overall taste. This is particularly evident in products like sodas, salad dressings, and dairy products.
The critical feature of flavor enhancement through acidity is the way it can elevate the overall tasting experience. Acidity can mask sweetness or promote freshness, making a product more appealing to consumers. However, it is vital to monitor the levels of acidity; excessive use can lead to overpowering flavors that detract from the intended culinary experience.
"Understanding the role of acidic concentration in industrial applications is not only about enhancing processes but also about ensuring safety and meeting regulatory standards."
Acidic Concentration in Agriculture
Acidic concentration plays a critical role in agriculture, profoundly influencing soil health and crop productivity. Understanding how acidity affects various aspects of farming is essential for both sustainable practices and enhancing crop yield. The management of soil pH is a key factor in ensuring optimal nutrient uptake by plants. Furthermore, the application of fertilizers and understanding their relationship with soil acidity contributes to achieving desired agricultural outcomes.
Soil pH Management
Effects on Crop Yield
The effects on crop yield are significant and multifaceted. pH levels influence plant growth directly and indirectly. Different crops have varying pH requirements; therefore, maintaining appropriate soil acidity is fundamental. For example, many vegetables thrive in slightly acidic soils, which promotes availability of vital nutrients. When pH is low, specifically below 5.5, the soil may become too acidic, resulting in nutrient deficiencies that can hinder growth, leading to lower yields. Crops affected by poor acidic conditions often exhibit stunted growth or reduced fruiting, which ultimately compromises the overall harvest.
Nutrient Availability
Optimal nutrient availability is closely linked to the soil's pH. In acidic soils, certain nutrients become less available, while others can become toxic to plants. For instance, phosphorus is essential for plant development, but it becomes less soluble in highly acidic soils. As a result, plants may struggle to acquire necessary nutrients such as copper and zinc, leading to lower agricultural output. Understanding this relationship assists farmers in making informed decisions regarding soil amendments, enhancing their ability to improve crop productivity.
Fertilizer Applications
Types of Fertilizers
The types of fertilizers available in the market are diverse and need careful consideration. Acidic fertilizers, such as ammonium sulfate or urea, can lower soil pH further. However, in certain contexts, they can contribute positively by providing necessary nitrogen. Utilizing these fertilizers effectively demands knowledge of one's soil condition. Farmers must balance acidity with crop requirements to ensure soil remains suitable for cultivation. Selecting the right type is crucial for improving soil structure and ensuring nutrient delivery to plants.
Environmental Impacts
The environmental impacts of fertilizer applications cannot be overlooked. Excessive use of fertilizers, particularly nitrogen-rich ones, may lead to soil acidification over time. This runoff can contaminate nearby water sources, creating problems like algal blooms, which affect aquatic ecosystems. Moreover, acidic soils contribute to erosion and loss of biodiversity. It is critical to strike a balance where agricultural practices remain productive while minimizing environmental damage, promoting sustainability in farming operations.
"Understanding how acidic concentration affects agriculture is key to enhancing food security and environmental health."
Environmental Implications of Acidic Concentration
Acidic concentration has significant environmental implications that cannot be ignored. Understanding these effects is crucial for both scientific research and real-world applications. Acidic compounds can alter natural systems, impacting water sources, soil quality, and biodiversity. The study of these implications informs policy choices and public awareness, highlighting the need for effective environmental management.
Acid Rain Effects
Impact on Water Bodies
Acid rain is a major consequence of acidic concentration, arising from pollutants such as sulfur dioxide and nitrogen oxides. When these compounds mix with atmospheric moisture, they create sulfuric and nitric acid, which then fall as precipitation. This aspect of acid rain leads to drastic changes in water chemistry.
The impact on water bodies is profound. It can lead to reduced pH levels in lakes and rivers, affecting fish populations and other aquatic organisms.
Key characteristics of this impact include:
- Decreased Biodiversity: Many species cannot thrive in more acidic conditions.
- Metal Leaching: Higher acidity can cause harmful metals to dissolve into water, which affects drinking water quality.
This consequence is significant to the article as it illuminates the direct link between acid concentration and the health of aquatic ecosystems. Its unique feature lies in the ripple effect on food webs and the overall health of the ecosystem, presenting both advantages in understanding ecological balances and disadvantages in requiring remediation efforts.
Ecosystem Damage
Another dimension of acidic concentration involves ecosystem damage. It encompasses various habitats beyond just aquatic environments. Acid rain can lead to soil degradation, where nutrient availability is disrupted due to leaching of essential nutrients like calcium and magnesium.
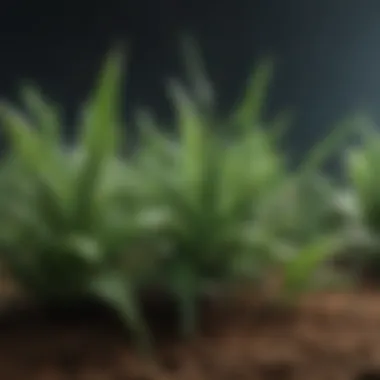
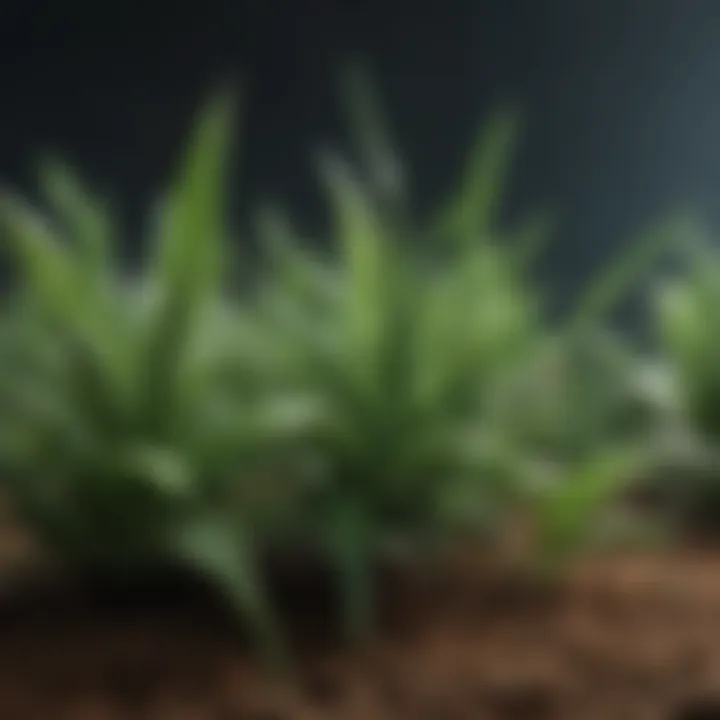
This aspect highlights how ecosystems are interconnected. Key characteristics of this damage include:
- Forest Decline: Trees weaken, making them more susceptible to pests and diseases.
- Altered Plant Growth: Changes in pH affect plant health and productivity.
Ecosystem damage is crucial for this article as it connects to broader environmental health. The unique feature of this damage is its long-term consequences, which may not be immediately observable but lead to significant biodiversity loss. Therefore, understanding this aspect helps in the evaluation of ecosystems' resilience and the need for conservation measures.
Mitigation Strategies
In response to the challenges posed by acidic concentration, various mitigation strategies can be adopted. They focus on reducing acidity's detrimental effects on the environment while promoting healthier systems.
Policy Approaches
A key component of addressing acidic concentration is developing policy approaches that regulate emissions of harmful substances. These policies can lead to significant improvements in air and water quality.
Highlighting policies include:
- Emission Standards: Set limits on pollutants released from industrial sources.
- Incentives for Clean Technologies: Encourage investments in environmentally friendly practices.
The importance of policy approaches is their capacity to create systemic changes. The unique feature here is the collaborative engagement of various stakeholders, including industries, governments, and civil organizations, which fosters shared responsibility. Their impact may not be instant, but they are crucial for long-term sustainability.
Technological Innovations
The role of technological innovations in combating acidic concentration is vital. New technologies help in detecting, measuring, and mitigating acid presence. For instance, advancements in sensor technology allow for real-time monitoring of pH levels in various environments.
Examples of significant innovations include:
- Bioremediation: Utilizing microorganisms to restore acidic soils.
- Waste Treatment Technologies: Methods to neutralize acidic effluents before release.
Technological innovations are beneficial as they provide practical solutions to environmental challenges. Their unique feature lies in their adaptability and scalability, making them accessible to various sectors from smaller farms to large manufacturing plants. Overall, these innovations enhance the potential for effective environmental management and restoration efforts.
Future Directions in Acidic Concentration Research
Research in acidic concentration is evolving. It plays a key role in numerous scientific areas. Innovations in technology and interdisciplinary studies are shaping future explorations. Understanding how acidic concentration affects biology, ecology, and industry is vital. This focus will allow for more accurate measurements, enhanced applications, and mitigation of adverse effects.
Emerging Technologies
Nanotechnology Applications
Nanotechnology has a significant impact on our understanding of acidic concentration. Its ability to manipulate materials at the molecular level presents numerous opportunities. In the context of acidic concentration, nanomaterials can be engineered to detect and neutralize acids effectively. This precision is crucial in fields such as environmental science, where monitoring pH levels in real-time is necessary.
The key characteristic of nanotechnology is its scalability. It can be tailored for specific tasks, enhancing both efficiency and effectiveness. For example, nanosensors can provide immediate feedback on acidic levels in water. This responsiveness is beneficial for both industrial and environmental applications. However, the unique feature of nanotech applications can have associated risks. Concerns about toxicity and environmental impact must be addressed to ensure safety.
Biotechnology Advancements
Biotechnology also contributes significantly to the understanding of acidic concentration. It utilizes biological systems to create solutions that address acidity-related challenges. One major aspect is the development of microbial systems to treat acid mine drainage or agriculture settings with low pH. Microbes can be engineered to thrive in acidic conditions, helping restore balance in ecosystems.
The key characteristic of biotechnology is its adaptability. Approaches can be tailored to specific environments and objectives. For example, genetically modified organisms can enhance crop resilience to acidic soils, improving agricultural productivity. However, some unique features of biotechnology raise ethical concerns and stability questions, making careful consideration essential.
Interdisciplinary Approaches
Integration of Chemistry and Biology
Integration of chemistry and biology is crucial for innovative research. By combining these disciplines, researchers can develop more comprehensive models of acidity in various contexts. Studying how organisms respond to acidity allows for a more nuanced understanding of ecological impacts and biological functions. This integration creates opportunities for improved acid management strategies across industries.
The key characteristic of this approach is its potential for holistic understanding. It enables teams to analyze how chemical interactions affect biological systems. This integrated research can lead to better regulatory frameworks for acid emissions and pollution, which is beneficial for public health and environment.
Collaborative Research Initiatives
Collaborative research initiatives are vital for advancing knowledge on acidic concentration. By pooling resources and expertise from different fields, researchers can tackle more complex problems. These initiatives encourage sharing of data and techniques, thus accelerating discovery. An example can be the partnership between universities and industries focused on developing acidic resilient crops.
The key characteristic of collaborative efforts is diversity in thought. Researchers with varied backgrounds bring fresh perspectives and innovative solutions. Such initiatives can lead to breakthroughs that may not occur in isolated environments. However, the unique feature of collaboration can also lead to challenges in communication and alignment on project goals, requiring strong leadership and clear objectives.
Culmination
In this article, the significance of acidic concentration has been thoroughly explored. Understanding the impacts and applications of acidic concentration is crucial for multiple fields, including chemistry, biology, and environmental science. The various aspects discussed highlight how acidity affects both ecosystems and human activities. By comprehending acidic concentration, we can appreciate its role in industrial processes, agriculture, and the environment.
Summary of Key Points
- Acidic concentration influences biological systems, affecting metabolism and cellular function.
- It plays a pivotal role in defining water quality and soil health.
- Different industrial applications rely on acidity for production and preservation methods.
- Effective measurement techniques such as titration and spectrophotometry offer insights into acidity levels.
- Environmental concerns, such as acid rain, necessitate ongoing study and mitigation strategies to protect ecosystems.
Understanding these points affirms the fundamental nature of acidity in influencing life forms and habitats.
The Importance of Continued Research
Continued research on acidic concentration is necessary due to its complex implications. The evolving scientific landscape brings forth new technologies and interdisciplinary approaches. For instance, advances in nanotechnology and biotechnology present innovative ways to manage acidity in various settings. Additionally, collaborative research initiatives can yield insights that enhance our understanding of the interplay between acidity and living systems.
It is important to not only keep knowledge current but also address emerging challenges related to acidic concentrations in the environment. Continuous exploration will lead to improved strategies for monitoring and managing acidity, ultimately contributing to better environmental and health outcomes.