The Snowflake Experiment: A Detailed Study of Nature's Art
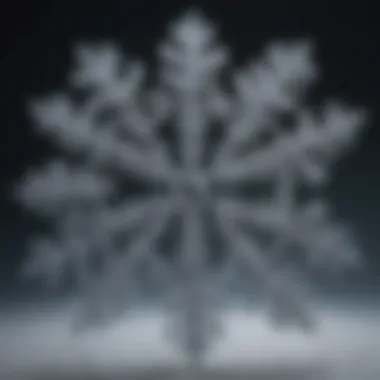
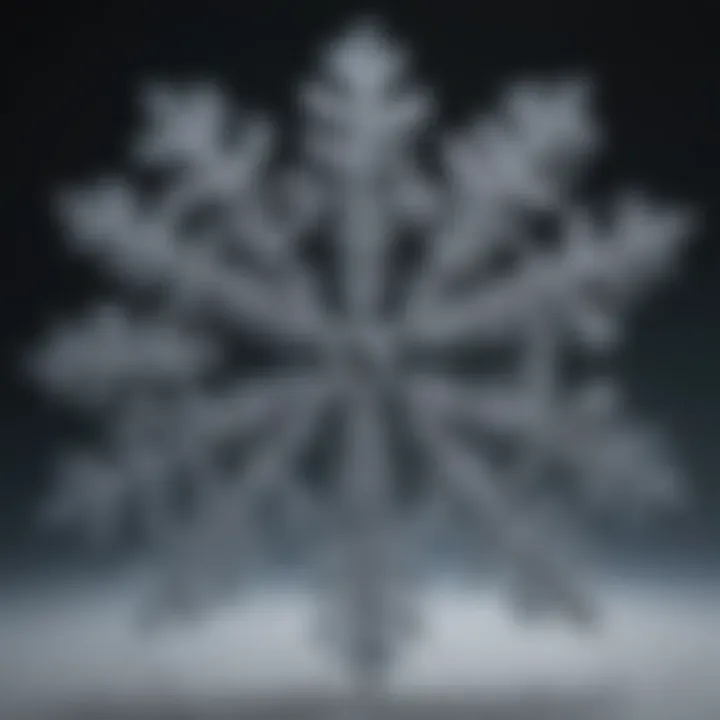
Intro
The Snowflake Experiment invites scholars to delve into the intricate world of snowflake formation and the various facets that accompany such phenomenon. Snowflakes, with their unique structures and varying morphologies, present an exceptional case for scientific inquiry. Understanding how these delicate formations develop offers insights into broader atmospheric dynamics and climate conditions. Through meticulous investigation, this article aims to bridge historical understanding with contemporary scientific methodologies.
Research Overview
Summary of Key Findings
Research into snowflake formation reveals several key findings that underscore the complexities involved. Notably, snowflakes tend to exhibit sixfold symmetry. This characteristic raises questions about molecular interactions and environmental conditions during their formation. Key observations include:
- Variation in snowflake shape is significantly affected by temperature and humidity levels.
- The presence of pollutants can alter their morphology, shedding light on anthropogenic impacts.
- Historical records indicate a rich diversity of snowflake types, emphasizing climatic variations over time.
Relevance to Current Scientific Discussions
The implications of studying snowflakes extend beyond mere curiosity. As researchers investigations into climate patterns evolve, understanding snowflake formation becomes increasingly relevant. The morphology of snowflakes serves as an indicator of atmospheric conditions at the time of their formation. Furthermore, findings from the Snowflake Experiment contribute to ongoing discussions regarding climate change. The potential for using snowflakes as proxies for historical climate conditions offers substantial value for climatologists and environmental scientists alike.
The Snowflake Experiment emphasizes the interconnectedness of snowflakes and climatic shifts, illuminating a path for further research.
Methodology
Research Design and Approach
The methodology employed in analyzing snowflakes encompasses both qualitative and quantitative approaches. Researchers often begin with a literature review to establish a theoretical foundation. This includes the examination of previous studies conducted in both laboratory and field settings.
In many cases, researchers have utilized advanced imaging techniques to capture intricate details of snowflake structures. High-resolution cameras and specialized equipment facilitate a thorough analysis of snowflake geometries.
Data Collection and Analysis Techniques
Data collection for the Snowflake Experiment typically involves several key techniques:
- Field Sampling: Snowflakes are collected directly from snowy environments to ascertain their properties in situ.
- Laboratory Experiments: Controlled conditions allow scientists to manipulate temperature and humidity to observe snowflake growth under various scenarios.
For data analysis, statistical methods are employed to correlate environmental factors with snowflake morphology. This includes software modeling that visualizes the impact of different variables on snowflake development.
In summation, the Snowflake Experiment offers an avenue for profound scientific exploration and understanding. By examining snowflakes not just as beautiful phenomena but also as critical indicators of climate dynamics, researchers pave the way for a deeper comprehension of our changing world.
For further reading, one can explore detailed data and findings on platforms like Wikipedia, Britannica, Reddit, and Facebook.
Prolusion to the Snowflake Experiment
The study of snowflakes offers a unique glimpse into the complex interplay of atmospheric conditions and crystallization processes. Understanding the formation of these delicate structures not only has scientific value, but also implications for meteorology and climate research. This section is crucial as it lays the foundation for grasping the intricacies of snowflake characteristics and the methodologies used to explore them.
Defining Snowflakes
Snowflakes are ice crystals that form within clouds when water vapor freezes. Each flake begins as a tiny ice nucleus, which collects more water vapor as it descends through the atmosphere. As the temperature and humidity levels vary, the water vapor crystallizes in distinct patterns, leading to the unique forms of snowflakes. The outcome of such processes results in a wide variety of designs, from simple hexagonal plates to more complex dendritic structures. This intrinsic diversity makes snowflakes a fascinating subject of study, providing insights into the micro-physical processes within the atmosphere.
Scientific Significance
Snowflakes hold significant importance in various scientific domains. From a meteorological perspective, they serve as crucial indicators of weather patterns and changes in atmospheric conditions. By analyzing snowflake formations, researchers can better understand temperature fluctuations, humidity levels, and overall climatic trends. Furthermore, the study of snowflakes contributes to fields such as crystallography and materials science, shedding light on the mechanisms through which simple molecules can organize into intricate structures. Understanding snowflakes can also enhance predictive models of snowfall and climate change, making their study not just academic but essential for real-world applications.
"Understanding the formation and characteristics of snowflakes aids in improving predictive models for weather and climate changes."
Through this exploration, readers will gain a deeper appreciation for the delicate balance of forces that shape these identities in nature.
Historical Context of Snowflake Studies
Understanding the historical context surrounding the study of snowflakes provides invaluable insights into the development of meteorological science and the cultural significance of these unique ice crystals. The journey of snowflake research spans centuries and intertwines with advancements in science and technology, making it critical to highlight key observations and theories that paved the way for modern appreciation of snowflakes. Recognizing these historical milestones helps scientists and educators appreciate how far we have come in this field and the importance of continued inquiry.
Early Observations and Theories
Dating back to ancient times, snowflakes have captivated human curiosity. Early philosophers and naturalists attempted to explain their beauty and complexity. Many believed that snowflakes were products of magical or divine forces. The first rigorous attempts at studying these structures began in the 17th century. Naturalists like Johannes Kepler recorded detailed observations and speculations about frozen water. These early theories laid a rudimentary foundation for understanding crystallization.
As advancements in technology took root, especially with the invention of the microscope, the study of snowflakes began to deepen. The ability to observe snowflakes at a micro level shifted the perspective, moving away from mystical explanations to scientific investigation. This transformation in understanding set the stage for future research and experimental designs focused on snowflake formation and morphology.
Key Contributions by Scientists
Wilson Bentley
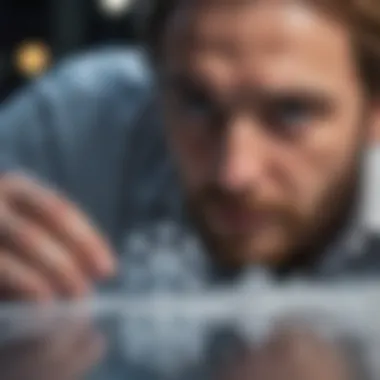
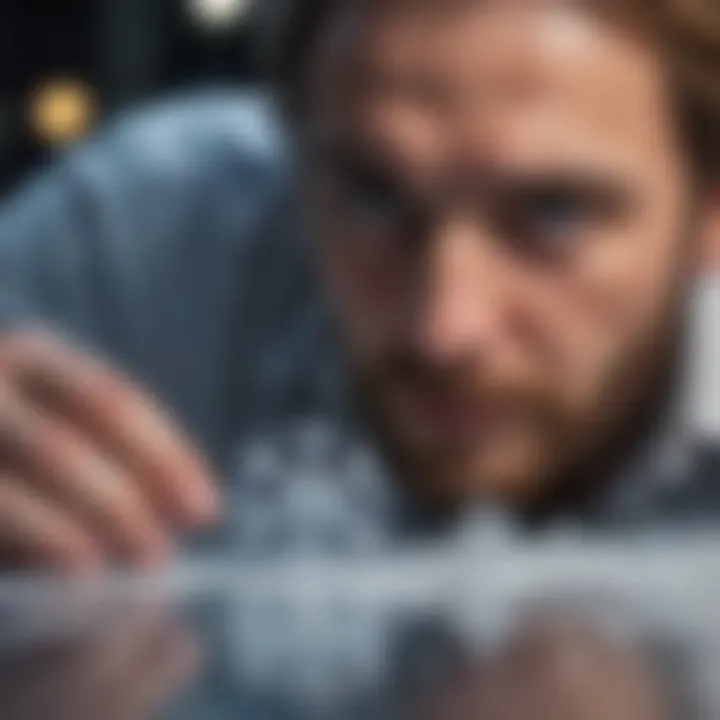
Wilson Bentley, an American farmer and photographer, significantly contributed to our understanding of snowflakes. He was the first to capture detailed photographs of snowflakes in the late 19th century, showcasing their intricate structures. Bentley's work is notable not only for the quantity of snowflakes he documented but also for his belief that no two snowflakes were alike. His meticulous collection provided irreplaceable data, and these photographs continue to be a reference in both scientific and artistic communities.
One key characteristic of Bentley's photography was its focus on showcasing the six-sided symmetry common in snowflake formation. This symmetry is essential in understanding the physics behind crystal growth and environmental factors influencing their structures. Bentley's passion for studying snowflakes fosters an appreciation for their beauty and diversity, making him a compelling figure in this article.
The Role of the Microscope
The invention of the microscope revolutionized science and had a profound impact on the study of snowflakes. It allowed scientists to observe crystals with unprecedented detail, uncovering complexities in their formations. Microscopes enabled researchers to shift from abstract theories to empirical data. Moreover, these instruments facilitated the discovery of various snowflake shapes and types, such as dendrites, plates, and columns.
A significant advantage of utilizing microscopes in snowflake research is the ability to visualize shapes and structures in real-time, enhancing the understanding of how atmospheric conditions affect crystallization. However, challenges remain, such as the limitations in capturing momentary formations during their descent through the atmosphere. Despite these challenges, the microscope remains a fundamental tool for advancing our knowledge of snowflake morphology and behavior.
The Science Behind Snowflake Formation
Understanding the science behind snowflake formation is critical for grasping the complexity of these unique natural structures. Snowflakes are not merely frozen water droplets; they are the result of intricate processes influenced by various atmospheric conditions and crystallization processes. This section delves into how each element contributes to the shape and characteristics of snowflakes, thus shedding light on their formation.
Atmospheric Conditions
Atmospheric conditions play a vital role in determining the properties of snowflakes. Three primary factors are essential: temperature, humidity, and pressure.
Temperature
Temperature serves as a fundamental aspect of snowflake formation. As the temperature decreases, the capacity of air to hold moisture lowers. This change affects not only the formation of snowflakes but also their size and structure. When the temperature hovers around -5 °C to -10 °C, the rate of crystallization is optimal, allowing for the development of intricate dendrite structures. This is why low temperatures are often seen as beneficial for producing beautiful snowflakes. However, too low temperatures can cause the snowflakes to become smaller and more compact, limiting their aesthetic variations.
Humidity
Humidity is another critical factor influencing snowflake formation. High humidity creates conditions for more water vapor to condense and crystallize. In environments where humidity levels exceed 90%, it leads to the growth of larger, more complex snowflakes. This characteristic makes humidity a popular aspect of snowflake morphology discussions. Nonetheless, excessive humidity can sometimes lead to wet snow, which may affect the snowflake's delicate structure and transform it into slush. Thus, while high humidity can enhance snowflake development, it can also pose challenges to their formation.
Pressure
Pressure is less frequently discussed yet remains significant in the formation of snowflakes. Atmospheric pressure affects air density and, subsequently, the movement of water vapor. At lower pressure, snowflakes tend to form rapidly due to the greater availability of water vapor. This characteristic renders low-pressure conditions beneficial when considering the efficiency of snowflake formation. However, fluctuating pressure can lead to instability in snowflake growth, producing inconsistent results. Thus, while pressure does not often take center stage, its influence on snowflake behavior is worthy of recognition.
Crystallization Processes
Following the atmospheric conditions, the crystallization process is the actual mechanism through which snowflakes take shape. This growth can be influenced by numerous factors, including:
- Nucleation: The initial formation of ice crystals occurs here.
- Molecular Arrangement: Water molecules bond in various orientations, leading to different shapes.
- Growth Conditions: Factors like temperature variations can affect how quickly crystals grow.
These processes collectively determine the myriad forms snowflakes can take, showcasing a breathtaking array of shapes and patterns that challenge our understanding of crystallography.
Methodologies in Studying Snowflakes
The exploration of snowflake formation encompasses a variety of methodologies that contribute profoundly to our understanding of these unique structures. Each methodology offers distinct advantages and potential drawbacks, which researchers must carefully consider when designing their studies. Methodologies typically consist of both experimental designs and imaging techniques, each playing a crucial role in revealing the intricacies of snowflake formation and morphology.
Experimental Designs
Field Studies
Field studies represent an essential aspect of snowflake research. They allow scientists to observe snowflakes in their natural environment. This method provides real-time data and captures the meteorological conditions present during snowflake formation. A key characteristic of field studies is their ability to document snowflakes as they fall from the sky, offering insights into live atmospheric processes.
The unique appeal of field studies lies in their practicality and the authenticity of the data gathered. They are especially beneficial for collecting samples directly impacted by local weather conditions. However, its disadvantages include variability due to changing environmental factors, making it challenging to replicate findings across different studies. Thus, while field studies offer rich data, they can also introduce complexities that must be addressed through careful study design.
Laboratory Simulations
Laboratory simulations provide controlled environments for studying snowflake formation. In this setting, researchers can manipulate variables such as temperature, humidity, and pressure to observe how these conditions affect crystal growth. The main advantage of laboratory simulations is the precision they offer. Researchers can conduct repeatable experiments that yield consistent results.
A notable feature of laboratory simulations is the ability to create a variety of conditions and observe the resultant snowflake structures in a systematic way. However, there are limitations to this approach as well. Simulated conditions may not perfectly reflect those in nature, which can impact the applicability of findings to real-world scenarios. Nonetheless, laboratory simulations are a vital part of the snowflake research landscape, contributing valuable insights that complement field studies.
Imaging Techniques
Cryomicroscopy
Cryomicroscopy is a cutting-edge imaging technique used to examine snowflakes at extremely low temperatures. This method enables researchers to visualize snowflake structures in detail without altering their natural state. The main characteristic of cryomicroscopy is its ability to capture high-resolution images while preserving the integrity of the ice crystals.
The unique advantage of this technique lies in its non-destructive nature. Researchers can analyze snowflakes without introducing heat or pressure that could alter their formation. However, the complexity and expense of cryomicroscopy equipment can be significant drawbacks for some research teams, limiting its widespread use.
High-Resolution Photography
High-resolution photography serves as an important tool in snowflake research. This method allows scientists to capture intricate details of snowflakes, making it easier to identify and classify different types. A key feature is the ability to document changes in the crystalline structures under varying environmental conditions.
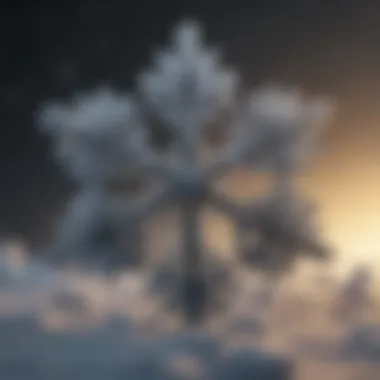
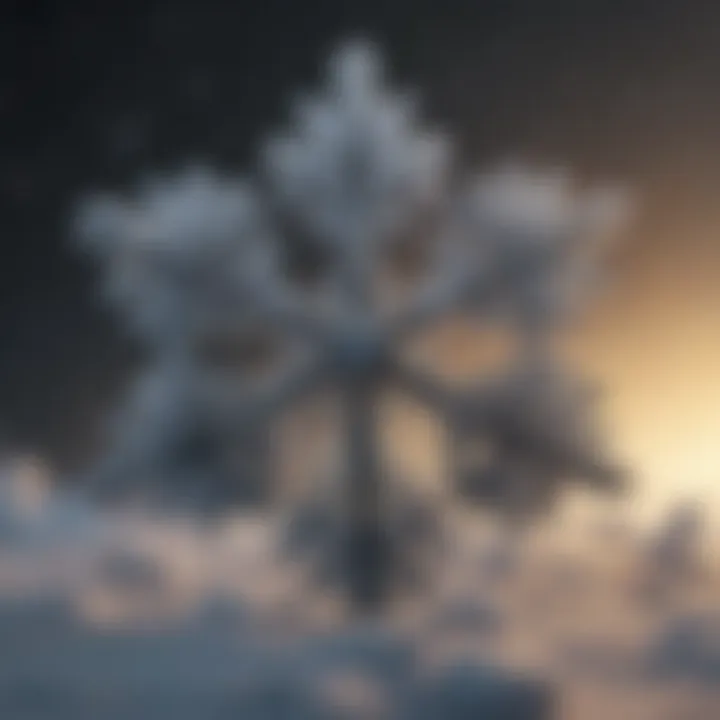
The appeal of high-resolution photography lies in its accessibility and simplicity compared to more advanced techniques. It can be performed with relatively standard photographic equipment and does not require intricate setups. However, photographs may not capture internal structures as effectively as other methods, which can lead to partial or distorted observations. Despite this limitation, high-resolution photography remains a valuable method for studying and understanding snowflakes.
Morphological Characteristics of Snowflakes
Understanding the morphological characteristics of snowflakes is essential to appreciate their complexity and significance in both meteorology and climate science. Each snowflake possesses a unique structure that reflects the environmental conditions during its formation. Investigating these characteristics allows researchers to draw connections between snowflake morphology and climatic variables. Additionally, categorizing snowflakes provides insight into broader meteorological phenomena. Thus, studying the morphological features of snowflakes goes beyond aesthetics; it is integral to understanding atmospheric processes.
Classification of Snowflake Types
Snowflakes can be classified into distinct types based on their morphology. This classification encompasses various shapes, including dendrites, plates, and columns. Each type exhibits unique characteristics that facilitate their identification and study.
Dendrites
Dendritic snowflakes are perhaps the most iconic shape. They feature intricate branching patterns that resemble tree branches, which contribute to their aesthetic appeal. Dendrites form through a process known as agglomeration, where water vapor crystallizes onto existing ice lattices. This branching structure creates a larger surface area, enhancing light reflection and giving dendrites their captivating appearance.
The key characteristic of dendrites is their complex structure, which also indicates the effectiveness of particular atmospheric conditions, such as high humidity and low temperature. Their formation typically occurs under ideal conditions, making them a popular subject in snowflake studies. However, their delicate structure can be a disadvantage. Dendrites may easily disintegrate when subjected to wind or temperature fluctuations, complicating data collection during research.
Plates
Plate snowflakes are characterized by their flat, symmetrical structures. These shapes often resemble hexagonal discs and result from specific humidity and temperature conditions during their formation. Plate snowflakes thrive when temperatures hover around -2 to -5 degrees Celsius and humidity is moderate.
The principal advantage of plates is their stability. They tend to maintain their structure better than dendrites under varying environmental conditions. This makes them advantageous for researchers aiming to capture detailed morphological data without risk of distortion. However, their relatively simple structure may offer less information regarding the atmospheric conditions compared to more complex types. Thus, they serve as a fundamental type to establish baseline data in studies.
Columns
Columnar snowflakes are less common than dendrites and plates. They are characterized by their elongated, cylindrical shape. Columns often emerge when formation occurs under low temperatures and vary in length and thickness. Their structure provides a clear indication of the specific environmental conditions under which they form.
The key characteristic of columns is their robustness. This unique shape provides considerable stability, allowing them to endure harsher weather conditions. Columns give researchers insights into microclimatic variations. However, their architectural simplicity limits the richness of data they can provide for atmospheric assessments. The presence of columns can indicate certain conditions, but they do not have the same complexity as dendritic shapes for study.
Mathematical Models of Snowflake Shapes
Mathematical models serve an essential role in studying the shapes of snowflakes. These models help quantify the relationships between environmental influences and the resulting morphology of snowflakes. By applying mathematical equations, researchers can predict snowflake types based on specific atmospheric parameters such as temperature and humidity.
Additionally, mathematical modeling allows for simulations of snowflake formation, offering a deeper understanding of their growth patterns and crystallization processes. Through these insights, scientists may develop better predictive capabilities in meteorological studies and better comprehend climate dynamics.
Impact of Climate on Snowflake Formation
The interaction between climate and snowflake formation is a crucial aspect of understanding how weather patterns and atmospheric conditions manifest. Climate influences not only the availability of moisture but also the temperature range at which snowflakes develop. In turn, snowflakes themselves can provide valuable information about these climatic conditions. Understanding this interplay offers insight into larger environmental changes and their implications.
Climate Change and Its Effects
Climate change poses significant risks to snowflake formation. Rising global temperatures can lead to more rain instead of snow, particularly in regions that traditionally experience heavy snowfall. This shift from solid to liquid precipitation alters the dynamics of winter weather, impacting ecosystems and water resources. For instance, warmer winters may decrease the number of snowy days, consequently reducing the conditions necessary for snowflake creation.
In addition, increased atmospheric moisture can influence the size and density of snowflakes. Changes in humidity levels play a central role in determining how snowflakes are formed. As the Earth's atmosphere warms, it can hold more moisture, leading to heavier and wetter snow. This can affect snow composition, potentially making it less stable and impacting areas reliant on snow for water supply.
Snowflakes as Climate Indicators
Snowflakes serve not only as a fascinating subject of study but also as indicators of climate conditions. Their unique formation can reflect ambient temperature, humidity, and other atmospheric variables. For example, the presence of intricate dendritic snowflakes might suggest colder temperatures and particular humidity levels during their formation, while simpler shapes could indicate different atmospheric conditions.
The shape and structure of snowflakes can serve as proxies for analyzing historical climate patterns. By studying snowflake characteristics over time, scientists can assess how climate changes have affected snowfall over the years. Observations collected from snowflakes aid researchers in tracking variations in weather patterns and provide a clearer picture of the ongoing changes in our climate.
"Snowflakes are not just beautiful; they are essential indicators of climate change. Their study can reveal much about our changing world."
Applications of Snowflake Research
The study of snowflakes has far-reaching implications beyond their aesthetic appeal. Understanding their formation and structure plays a crucial role in various applied fields, particularly in meteorology, climate science, and environmental research. The unique qualities of snowflakes can offer insights into weather patterns and climate dynamics. This section explores the vital applications of snowflake research, emphasizing their relevance in today's scientific inquiry.
Understanding Weather Patterns
Snowflakes are remarkable indicators of atmospheric conditions. Each snowflake reflects the specific temperature, humidity, and other variables present during its formation. By analyzing snowflake characteristics, researchers can gain insights into prevailing weather patterns. For instance, fluctuations in snowflake morphology can signify shifts in weather systems.
An extensive understanding of snowflakes allows meteorologists to:
- Identify types of precipitation, such as snow vs. rain.
- Forecast weather events more accurately.
- Monitor changes in climate over time.
The variability of snowflakes offers a tangible record of atmospheric changes. By systematically collecting and analyzing snowflakes during different seasons, scientists can create a database that assists in predicting future weather trends.
"Snowflakes serve as a window into the atmospheric processes that govern our climate."

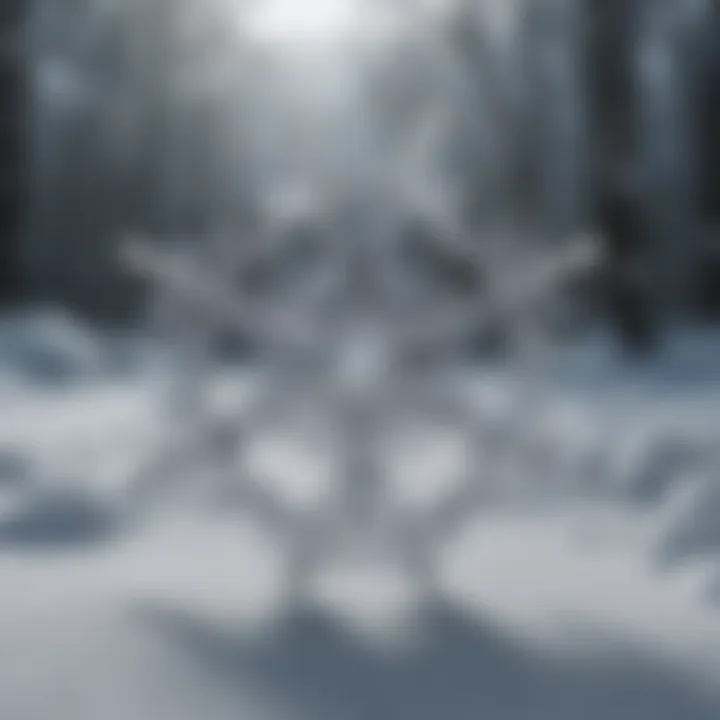
Insights into Meteorology
Severe weather systems often exhibit unique snowflake formations. In storm conditions, the size and shape of snowflakes can change rapidly. Understanding these patterns can improve models used in weather prediction. Moreover, studying snowflakes can enhance our comprehension of cloud microphysics, such as how cloud particles interact and form precipitation.
Furthermore, insights gained from snowflake research contribute to:
- Improved models for understanding atmospheric dynamics.
- Better predictive capabilities for severe weather events.
- Enhanced climate models to assess the impact of climate change on precipitation patterns.
The implications of snowflake research stretch into diverse areas such as hydrology and agriculture. It opens avenues for studying how changes in snowfall patterns influence water resources and crop yield. As climate events become more extreme, the relevance of understanding snowflake formation continues to grow.
Challenges in Snowflake Research
Researching snowflakes presents unique challenges that are often overlooked in discussions about atmospheric sciences. These challenges can significantly impact the quality and reliability of the data collected, which in turn shapes our understanding of snowflake formation and its implications for climate science. The variability inherent in snowflake samples draws attention to the precision required in data collection processes.
Variability in Data Collection
Snowflakes are influenced by myriad factors as they form in the atmosphere, leading to substantial variabilitiy in their characteristics. This variability poses difficulties in establishing consistent and replicable data across different research studies. For instance, minute changes in temperature and humidity can result in drastically different snowflake structures.
Factors influencing this variability include:
- Atmospheric conditions at different altitudes
- Microphysical processes occurring during snowflake growth
- External influences like wind patterns and pollution
Researchers encounter obstacles not only in capturing snowflakes but also in classifying and analyzing them accurately. This inconsistency affects the reliability of the findings, making it imperative for studies to adopt meticulous methodologies to ensure reproducibility and accuracy.
Limitations of Current Techniques
Despite advancements in technology, several limitations still hinder effective snowflake research. Current imaging techniques, while sophisticated, often fall short in capturing the intricate details of snowflakes in their natural setting. Some of the common limitations include:
- Insufficient resolution in standard photographic techniques
- Difficulty in maintaining the integrity of snowflakes during transport to laboratories
- Inadequate tools to measure ambient conditions during collection
The limitations not only complicate the data acquisition process but also necessitate continued innovation in research techniques. Solutions must focus on improving imaging capabilities and developing methods that allow for better real-time observation of snowflake characteristics without risking their physical structure.
"Understanding and overcoming the challenges of snowflake research is critical for enhancing our knowledge of climatic impacts and atmospheric science."
In summary, while snowflake research holds significant potential for insights into climate patterns and weather systems, the challenges related to data variability and limitations in current research techniques demand ongoing attention. Researchers must remain vigilant and adaptive to overcome these hurdles, ensuring that the study of snowflakes continues to evolve and inform our understanding of a changing climate.
Future Directions in Snowflake Research
The field of snowflake research is more relevant than ever as climate change continues to manifest in various ways. New directions in this study are crucial for improving our understanding of snowflake formation and behavior under changing environmental conditions. The significance of exploring future directions in snowflake research can be attributed to several factors, including technological advancements, interdisciplinary collaborations, and the growing need for accurate climate models.
Emerging Technologies
Technological progress has revolutionized the way researchers study snowflakes. Innovations in imaging technology, such as cryogenic electron microscopy, allow scientists to visualize snowflake structures in unprecedented detail. These advanced imaging methods provide high-resolution data, illuminating the complex microstructural features of snowflakes. This not only enhances classification systems but also improves our comprehension of how changes in atmospheric conditions affect snowflake development.
Moreover, machine learning is beginning to play a pivotal role in snowflake research. By utilizing algorithms trained on vast datasets, researchers can analyze patterns within snowflake formations more efficiently. This approach can enhance predictive models related to snowfall, helping meteorologists and climate scientists respond to changing weather patterns effectively.
Interdisciplinary Approaches
The future of snowflake research also lies in collaboration across various scientific fields. Traditionally seen as a niche within meteorology and atmospheric sciences, snowflake research benefits from interdisciplinary approaches. For instance, the integration of physics, chemistry, and environmental science deepens the investigation of how snowflakes can serve as indicators of climate shifts.
Aspects such as the chemical composition of snowflakes reveal information about the atmosphere, including pollution levels and seasonal changes. This examination can foster discussions among environmental scientists, chemists, and meteorologists, leading to more holistic approaches in understanding climate change.
"Interdisciplinary collaboration can shed light on the multifaceted nature of snowflakes, linking atmospheric studies with environmental science and physics."
Additionally, educational institutions and research organizations are encouraged to collaborate on snowflake research projects, thus promoting knowledge sharing and encouraging innovative experimental designs. This synergy will enhance the quality and depth of research, ultimately benefiting our understanding of snowflakes in a rapidly changing world.
The End
The examination of snowflakes through The Snowflake Experiment yields significant insights into both natural phenomena and scientific methodologies. This article has presented numerous aspects related to snowflake formation, characteristics, and implications of understanding their behavior, specifically in relation to climate and meteorology. The conclusion summarizes the importance of these findings and suggests pathways for future investigation, validating the relevance of ongoing research in this field.
Summary of Findings
Throughout this exploration, we dissected various components of what defines and influences snowflakes. Key findings include:
- Formation Conditions: The atmospheric conditions such as temperature, humidity, and pressure were identified as crucial factors in the crystallization processes that lead to the unique structures of each snowflake.
- Scientific Techniques: The methodologies employed, especially the advanced imaging techniques like cryomicroscopy, revealed the morphological traits and variety among snowflakes, highlighting the importance of technological innovation in scientific studies.
- Impact of Climate: Our discussion on climate change illustrated how shifts in environmental factors can lead to alterations in snowflake formation, making them crucial indicators of climate health.
These findings collectively underscore how snowflake research not only enhances our understanding of meteorological phenomena but also opens the door to broader discussions about climate change and ecological sustainability.
The Importance of Continued Research
As highlighted so far, the investigations into snowflakes extend beyond aesthetic fascination. Continuing this research is vital for several reasons:
- Climate Indicators: With climate change being a pressing global issue, snowflakes serve as both an interesting study and a barometer for ecological shifts. Monitoring their changes can provide valuable data regarding climate trends.
- Interdisciplinary Applications: Insights from snowflake studies intersect various disciplines, including physics, environmental science, and art. This intersection could lead to innovative solutions to environmental problems, fostering cooperation among diverse fields.
- Technological Advancements: Innovations in research methodologies, like those examined in this article, continually improve our approach to studying snowflakes and other natural phenomena, making this a dynamic field of study.
In summary, the conclusion emphasizes the cumulative knowledge gained about snowflakes and advocates for sustained and enhanced research efforts. The intricate details of these frozen crystals not only reflect nature's artistry but also offer vital clues about our planet's changing climate.