Comprehensive Overview of siRNA Design Principles
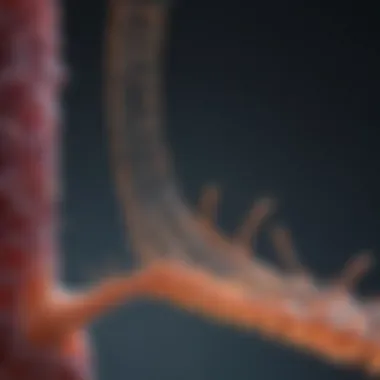
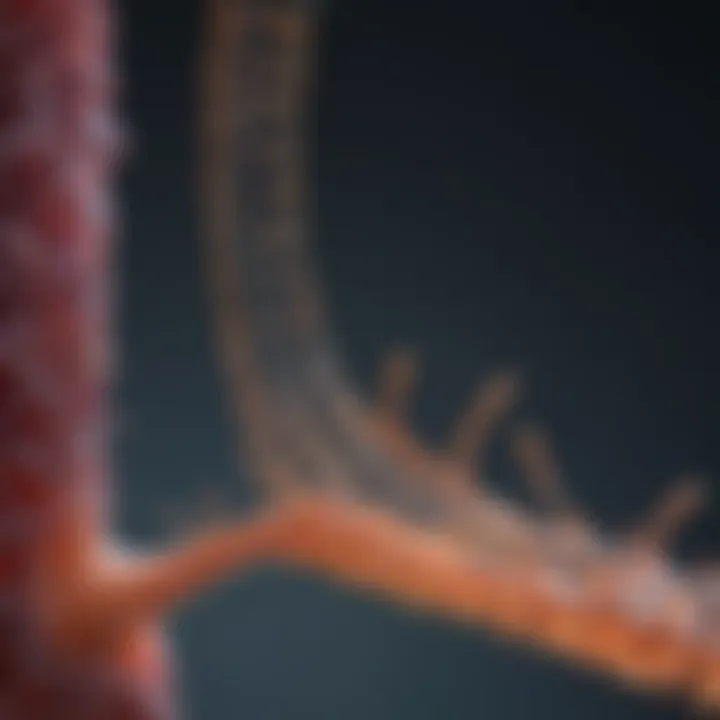
Intro
Small interfering RNA (siRNA) plays a significant role in the realm of gene silencing, a critical mechanism harnessed in various scientific and therapeutic applications. The design of siRNA is not a trivial task; it requires a nuanced understanding of its principles, structural characteristics, and the latest methodologies used in its creation. By diving into the intricacies of siRNA design, we can better grasp its vital contributions to molecular biology and medicine.
This overview aims to illuminate the underlying principles instrumental in effective siRNA design, alongside its practical applications. We will cover essential topics, from fundamental concepts to cutting-edge tools that facilitate the process of designing siRNA. Moreover, this examination will place a spotlight on their relevance to contemporary scientific dialogs, emphasizing their implications for advancements in research and treatment strategies.
Research Overview
Summary of Key Findings
The exploration of siRNA design reveals several critical factors affecting efficacy and specificity. Key findings indicate that effective target selection, optimization of structural elements, and appropriate chemical modifications are paramount to achieving desired gene silencing outcomes. Notably, the choice of RNA sequences must consider off-target effects, which can hinder therapeutic effectiveness.
Relevance to Current Scientific Discussions
In the ever-evolving landscape of molecular biology, siRNA technology remains at the forefront of discussions about genetic therapies and personalized medicine. As researchers and clinicians continue to investigate the potential of RNA interference, understanding siRNA design principles is crucial. Its relevance extends to various studies aimed at treating diseases, including cancers and genetic disorders, thereby influencing therapeutic development and strategies.
Methodology
Research Design and Approach
This article will provide a methodical approach to siRNA design, highlighting various strategies used in current research. By discussing the principles that underpin siRNA effectiveness, the article will guide the reader through existing design frameworks and methodologies employed in academic and clinical settings.
Data Collection and Analysis Techniques
Data is often collected from multiple sources, including experimental validations and computational analyses. Various algorithms and bioinformatics tools are employed to predict optimal siRNA sequences based on target mRNA characteristics. Analysis techniques may include assessing knockdown efficiency, measuring off-target effects, and verifying desired outcomes through quantitative methods.
Understanding these methodologies is integral for students, researchers, and healthcare professionals seeking to harness the potential of siRNA in their respective fields. Through comprehensive insight into siRNA design, we aim to equip the audience with the knowledge necessary for employing these techniques in research or therapeutic applications.
Prologue to siRNA Technology
The exploration of small interfering RNA (siRNA) technology emerges as a vital area of study in molecular biology. siRNA plays a pivotal role in the process of RNA interference, a mechanism that governs gene silencing. Understanding siRNA technology is crucial, especially for researchers focused on genetics, therapeutics, and biomedical innovations. This section aims to offer clarity on the basics of siRNA and how its mechanisms can be utilized in various contexts.
Definition and Mechanism of siRNA
SiRNA is a class of double-stranded RNA molecules, typically 20-25 base pairs in length. These molecules can silence specific genes through a process known as RNA interference. The mechanism begins with the introduction of siRNA into the cell, which leads to the unwinding of the double strands. Subsequently, the guide strand binds to its complementary mRNA target, prompting the RNA-induced silencing complex (RISC) to degrade the mRNA. This degradation prevents the translation of the targeted gene into a protein, effectively silencing its expression.
Key Features of siRNA Mechanism:
- Specificity: siRNA can be designed to target precise mRNA sequences, making it a powerful tool for knocking down specific genes.
- Dual Strands: The two strands of siRNA work together to enhance stability and efficacy in gene silencing.
- Natural Process: The mechanism mimics a natural defense pathway, allowing for potential applications in combating viral infections and regulating gene expression.
Understanding these features is crucial, as they lay the groundwork for applying siRNA in various fields, including therapeutic development and functional genomics.
Historical Context and Development
The discovery of RNA interference paved the way for siRNA technology. The journey can be traced back to early studies in the late 1990s. The initial identification of small RNA molecules that influenced gene expression offered exciting possibilities for gene regulation. The pivotal work by Andrew Fire and Craig Mello in 1998 demonstrated that introducing double-stranded RNA into nematodes could induce the silencing of specific genes. This groundbreaking discovery laid the foundations for modern siRNA research.
As research progressed, the understanding of siRNA design and application expanded significantly. The advent of synthetic siRNA accelerated the pace of discovery, allowing researchers to create specific siRNA constructs tailored for their experiments. This approach not only validated the RNA interference pathway but also established siRNA as a crucial tool in genetic research and therapy.
"The development of siRNA technology revolutionized the field of genetics, providing researchers with the means to explore gene function and regulation in unprecedented ways."
From its initial discovery to current applications in gene therapy, siRNA technology has continued to evolve. Researchers now utilize a variety of design strategies and computational tools to optimize siRNA design for diverse applications, signaling a transformative era in molecular biology and therapeutics.
Fundamental Principles of siRNA Design
Understanding the fundamental principles of siRNA design is crucial for maximizing the efficacy of RNA interference technology. The effectiveness of siRNA in gene silencing is heavily dependent on several key considerations: target gene selection, sequence characteristics, and structural aspects. Each of these principles plays a significant role in determining how well the siRNA will function in various biological contexts. An informed design process can lead to more successful outcomes in both research and clinical applications.
Target Gene Selection
Choosing the right target gene is the first and possibly the most critical step in siRNA design. The success of silencing a specific gene depends on its accessibility and expression levels within the chosen system. Target genes should ideally have a high degree of sequence uniqueness to avoid off-target effects. Researchers often employ various bioinformatics tools to assess potential target sites within the gene sequence. It is advisable to focus on exonic regions since these areas are less likely to vary among different individuals, thus increasing the likelihood of uniform gene silencing across a population. Moreover, consideration of the biological consequences of silencing a gene is important. The target should not only be relevant to the study but also essential enough to yield observable effects when silenced.
Sequence Characteristics
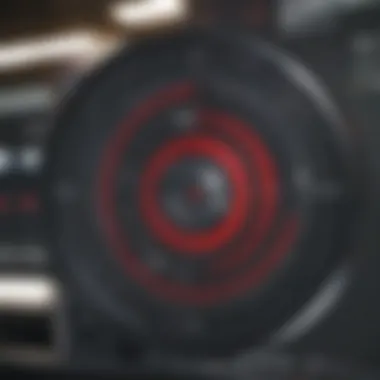
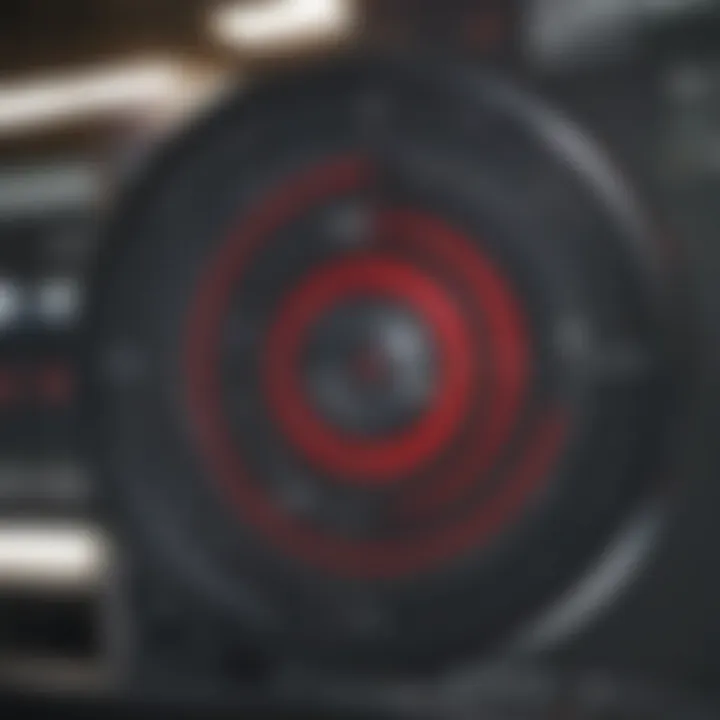
The sequence characteristics of siRNA are pivotal to its binding efficacy and overall functionality. The design primarily revolves around a 21-nt double-stranded RNA format that generally exhibits a symmetric configuration. Sequences with a high GC content tend to offer better stability and thermodynamic properties compared to those with low GC content. When evaluating sequence design, it is crucial to ensure that the siRNA is complementary to the target mRNA. This allows the RNA-induced silencing complex (RISC) to effectively bind and initiate the cleavage of the mRNA strand. Additionally, researchers must avoid sequences that might form secondary structures or that contain repetitive regions, which can inhibit RISC binding and activity.
Structural Considerations
The structural integrity of siRNA also plays a vital role in its functionality. Properly designed siRNA molecules must exhibit certain structural features to enable effective interaction with the RISC. One fundamental aspect is the formation of a 5' phosphate and a 3' hydroxyl group that are necessary for siRNA stability and activity. Furthermore, the presence of a two-nucleotide overhang on the 3' end of each strand enhances binding affinity. Optimal structural characteristics can also mitigate degradation by cellular nucleases, thus prolonging the half-life of siRNA within the target cells. Researchers often employ chemical modifications, such as locked nucleic acids or phosphorothioates, to improve the resistance of siRNA to nuclease degradation and increase overall efficacy.
Effective siRNA design requires careful consideration of target selection, sequence, and structural integrity to achieve desired silencing outcomes.
By understanding these fundamental principles, researchers can better design siRNAs that are not only effective in silencing targeted genes but also minimize off-target effects and enhance therapeutic potential.
Chemical Modifications in siRNA
The role of chemical modifications in small interfering RNA (siRNA) design is crucial for enhancing the functionality and effectiveness of therapeutic applications. Such modifications serve to improve the stability of siRNA molecules, increase their efficacy in gene silencing, and reduce off-target effects. This section will delve into the introduction of these modifications, their implications on stability and efficacy, and the common types that researchers often employ in clinical and laboratory settings.
Foreword to Modifications
Chemical modifications refer to deliberate changes made to the siRNA structure. These alterations can involve the backbone, sugar, or even the RNA bases. Modifications can diversify the properties of siRNAs, offering specific advantages like enhanced stability in biological environments and improved cellular uptake. For instance, by altering the ribose sugar or replacing certain nucleotides, researchers can ensure that the siRNA is less prone to degradation by nucleases, enzymes that break down RNA molecules.
A common approach is phosphorothioate modification where one non-bridging oxygen atom in the phosphate backbone is replaced by sulfur. This method has been widely used to extend the half-life of siRNAs in biological systems, allowing them to remain effective longer.
Effects on Stability and Efficacy
The effects of chemical modifications on the stability and efficacy of siRNA are significant. Increased stability can lead to improved silencing activity by ensuring that a larger fraction of the siRNA survives longer in the cellular environment. This longevity permits prolonged interaction with the target mRNA, enhancing the overall gene silencing capability.
Moreover, optimal modifications have been shown to positively influence the binding affinity of siRNA to the RNA-induced silencing complex (RISC). This complex is critical for the mechanism of action of siRNA as it carries out the silencing process. If siRNA binds more effectively, the resultant gene knockdown is more pronounced and specific. On the contrary, poorly designed siRNAs or those lacking appropriate modifications might encounter rapid degradation, leading to ineffective gene silencing. Thus, the consideration of modifications is fundamental when designing siRNAs for therapeutic or experimental purposes.
Common Types of Modifications
Several common types of modifications are recognized in siRNA design. These include but are not limited to:
- 2'-O-Methylation: This modification adds a methyl group to the 2' position of the ribose sugar. It is known to enhance resistance against nuclease degradation and improve binding affinity to target mRNA.
- Locked Nucleic Acids (LNA): LNAs are modified RNA nucleotides that have a methylene bridge connecting the 2' and 4' positions of the ribose. They can increase binding affinity and enhance stability, making them particularly useful in specific applications.
- Phosphorothioate: As mentioned before, this modification replaces an oxygen in the phosphate backbone with sulfur. This leads to greater resistance to degradation by nucleases.
- Amino modifications: These involve the addition of various functional groups to enhance the overall uptake of siRNA by cells.
Using these specific modifications in siRNA design can lead to highly effective gene silencing outcomes, thereby expanding the applications of siRNA technology in both research and clinical settings.
In summary, exploring chemical modifications in siRNA design is fundamental to enhancing their therapeutic potential. The right combination of modifications can optimize the delivery, stability, and efficacy of siRNAs, making them powerful tools in gene therapy and molecular biology.
Design Tools and Algorithms for siRNA
Design tools and algorithms play a critical role in the effective creation of small interfering RNA (siRNA) sequences. Given the complexity of gene expression and RNA interactions, specific software has emerged to streamline the siRNA design process. These tools help researchers optimize their sequences, ensuring high silencing efficacy while minimizing off-target effects. A systematic approach to siRNA design increases the reliability of experimental outcomes, which is vital for both academia and clinical applications.
Overview of Design Algorithms
Many different algorithms are utilized in siRNA design, each employing distinct methodologies for predicting the effectiveness of siRNA sequences. These algorithms generally evaluate factors such as sequence uniqueness, thermodynamic stability, and target accessibility. Some of the well-known algorithms include the Ambion siRNA Design Tool, Dharmacon's siRNA Design Tool, and the RNAi Consortium's design algorithm.
Most algorithms prioritize the identification of a unique target site within the mRNA sequence. This is accomplished through scoring systems that analyze sequence patterns for optimal targeting. For example, algorithms often incorporate considerations based on known targets that have succeeded in previous studies.
Comparative Analysis of Popular Tools
A comparative analysis reveals variances in the effectiveness and user-friendliness of the most popular siRNA design tools:
- Ambion siRNA Design Tool: This tool is known for its comprehensive algorithm that calculates the siRNA’s potential effectiveness based on a variety of parameters, including GC content and the presence of specific nucleotides. It also provides users with information about potential off-target sequences.
- Dharmacon siRNA Design Tool: Dharmacon offers a robust interface that allows users to input specific target sequences and generates multiple siRNA candidates for analysis. Its strengths include extensive databases and normalization factors that assess the likelihood of success.
- The RNAi Consortium Tool: This resource provides a systematic design platform that integrates community feedback and empirically-tested data. It is especially valuable for researchers looking for validated siRNA sequences.
Although performance across these tools can vary based on the specific target genes, users should consider multiple factors such as ease of use, access to historical data, and available support when selecting a design tool.
User-Friendly Platforms for siRNA Design
An increasing number of user-friendly platforms now exist to facilitate siRNA design for both novice and advanced users. Many platforms offer intuitive interfaces that simplify the complex task of inputting data and interpreting results. This accessibility is essential for enhancing productivity and driving research forward.
Several notable platforms include:
- GenePharma: This platform is renowned for its ease of navigation and comprehensive database, allowing users to find effective siRNA sequences efficiently.
- Sigma-Aldrich: Offers an advanced siRNA design tool with an extensively researched database and user-specific recommendations that enhance the likelihood of successful gene silencing.
- siRNA.org: A freely accessible online resource that aggregates various design tools and databases, making it easy for users to generate siRNA candidates rapidly.
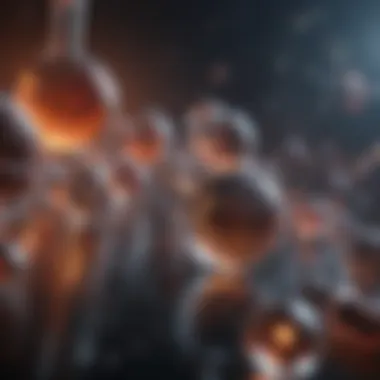
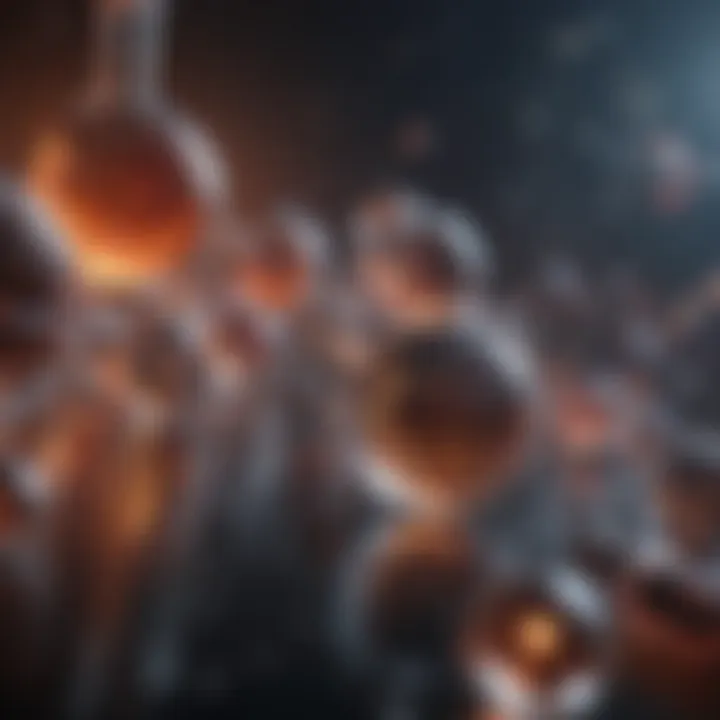
Integrating these platforms into research workflows enables researchers to engage in more productive discussions and decisions about the best siRNA sequences to test in their experiments.
It is important to remember that the choice of design tools can substantially affect the outcomes of siRNA experiments.
Experimental Validation of siRNA Design
Validating the design of small interfering RNA (siRNA) is a critical process that ensures the efficacy and reliability of gene silencing. Experimental validation provides the necessary empirical evidence to confirm that designed siRNAs will effectively reduce the expression of target genes. This step is essential in both research applications and potential clinical implementations where unintended off-target effects must be minimized. The need for rigorous validation stems from the complexities involved in RNA interference mechanisms, wherein factors such as the sequence, structure, and cellular environment can influence the performance of siRNAs.
By validating siRNA designs, researchers can identify optimal sequences that achieve significant knockdown of gene expression while limiting any adverse effects. This process often includes various testing approaches that assess effectiveness and specificity of siRNA molecules.
In Vitro Testing Approaches
In vitro testing is a cornerstone of siRNA experimental validation, providing an initial platform to assess the functionality of siRNAs before moving to complex in vivo systems. This phase typically involves several techniques such as:
- Cell Culture: Culturing appropriate cell lines that express the target gene allows researchers to evaluate the knockdown efficiency of the siRNA.
- Quantitative PCR (qPCR): This technique measures the mRNA levels post-transfection, offering quantitative insight into the levels of gene silencing achieved by the siRNA.
- Western Blotting: A method for protein assessment, helping in confirming that reductions in mRNA levels correlate with diminished protein expression.
- Reporter Assays: Introducing luciferase or GFP reporters provides a visual confirmation of successful gene silencing, often yielding quantitative data as well.
These approaches facilitate a controlled environment to precisely analyze the behaviour of siRNAs, enabling the identification of the most effective candidates for downstream applications.
In Vivo Application and Challenges
Transitioning from in vitro to in vivo evaluation introduces new layers of complexity due to the dynamic nature of living organisms. Testing siRNAs in living models is important for understanding the systemic effects, delivery mechanisms, and overall therapeutic efficacy. However, this step poses various challenges:
- Delivery Mechanisms: Effective delivery of siRNA to target tissues remains a primary hurdle. Many strategies, including lipid nanoparticles and viral vectors, are explored to ensure adequate bioavailability and cellular uptake.
- Off-Target Effects: It is vital to ensure that siRNAs do not inadvertently silence unintended genes, which could lead to toxic side effects. Thorough screening is necessary to identify and mitigate these risks.
- Efficacy Across Models: Results from animal models may not always directly translate to human responses, necessitating more tailored approaches regarding species and genetic background.
- Regulatory Hurdles: The pathway from experimental validation to clinical application involves numerous regulatory checkpoints. Understanding these regulations is crucial for successful translation into therapies.
Experimental validation is not merely a checkpoint; it assures that the siRNAs are both specific and efficacious in their intended roles.
Applications of siRNA in Research
The applications of small interfering RNA (siRNA) in research are crucial for understanding gene function and regulation. siRNA technology facilitates the targeted silencing of specific genes, enabling researchers to investigate gene roles in various biological processes. This approach's significance lies in its ability to provide insights into gene expression and pathway mechanisms, ultimately advancing our comprehension of molecular biology and genetics.
Functional Genomics and Gene Knockdown
In functional genomics, siRNA plays a pivotal role by allowing researchers to knock down genes systematically. By designing siRNAs that target specific mRNA transcripts, scientists can effectively reduce or eliminate the expression of these genes. This silencing can be applied to study knockdown effects across a wide array of cellular contexts. Researchers can explore cellular responses, validate gene functions, and identify gene interactions. Various cell types, including human, animal, and plant systems, are amenable to these experiments, making siRNA a versatile tool in functional genomics.
The advantages of gene knockdown with siRNA include:
- Precision: siRNAs specifically target chosen mRNAs, reducing off-target effects often associated with other methods.
- Efficiency: siRNA can induce rapid and efficient silencing compared to other gene editing tools.
- Accessibility: siRNAs can be easily synthesized and transfected into cells, allowing straightforward experimental setups.
However, it is important to consider that not all siRNAs will effectively knock down their intended targets. The design process must be meticulously executed, considering factors such as sequence specificity, the efficiency of delivery methods, and potential immunogenicity to ensure successful gene knockdown in the chosen system.
Pathway Analysis using siRNA Technology
siRNA also provides significant advantages in pathway analysis. By silencing genes within defined pathways, researchers can ascertain the functional role of specific components in broader biological processes. This method helps to elucidate complex cellular signaling networks and interactions important for cell fate, growth, and response to stimuli.
Through targeted siRNA assays, scientists can:
- Map Pathway Interactions: By knocking down key genes, researchers can discover novel interactions and contributions within signaling pathways.
- Identify Essential Pathway Components: Determining which genes are necessary for pathway functionality aids in understanding diseases associated with those pathways.
- Investigate Drug Targets: siRNA can help validate potential therapeutic targets in critical pathways, providing insights into drug development and optimization strategies.
The capacity of siRNA to dissect pathways amplifies its utility in biomedical research, facilitating innovative approaches to uncover molecular mechanisms.
Clinical Implications of siRNA Design
The clinical implications of small interfering RNA (siRNA) design are profound, impacting various aspects of therapeutic development and delivery. Given its potential to silence specific genes, siRNA holds promise for treating conditions that have been difficult to manage using traditional methods. The design of siRNA plays a central role in realizing this promise, as it directly influences the efficiency and specificity of gene silencing.
One of the most significant benefits of siRNA in clinical applications is its ability to target and downregulate disease-causing genes. This method provides a basis for innovative treatments, particularly for genetically driven disorders and cancers. By specifically targeting pathogenic gene expression, siRNA can allow for more precise control of physiological pathways compared to conventional drugs, reducing the risk of off-target effects.
Additionally, siRNA can be designed to enhance the therapeutic repertoire. For instance, it can be used to modulate pathways involved in inflammation, cancer development, or viral infections. These applications make siRNA a versatile tool in the clinical setting. However, successful implementation requires careful design and consideration of several factors, which leads us to the therapeutic applications.
Therapeutic Applications in Disease Treatment
Therapeutic applications of siRNA are steadily gaining traction in clinical research. Various conditions, including cancers, neurodegenerative diseases, and viral infections, are being targeted using siRNA technology.
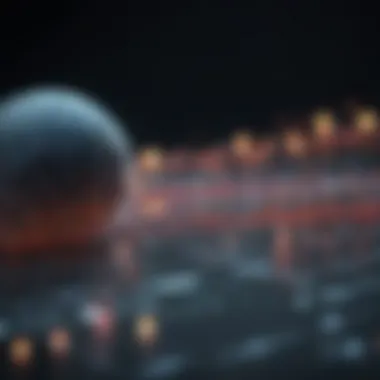
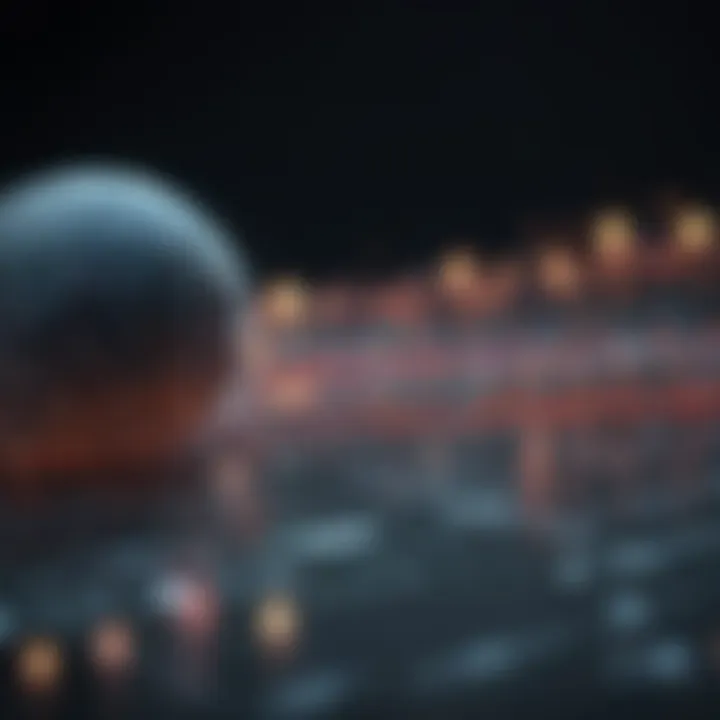
- Cancer Treatment: siRNA can target oncogenes and tumor suppressor genes, effectively reducing tumor cell proliferation. The ability to silence specific genes has opened avenues for developing gene therapies that could complement existing treatments, such as chemotherapy and radiotherapy.
- Viral Infections: Conditions caused by viral pathogens, such as hepatitis and HIV/AIDS, can also be effectively managed using siRNA. For example, siRNA can inhibit viral replication by targeting viral genes, leading to reduced disease progression.
- Genetic Disorders: In conditions caused by genetic mutations, designing siRNA to target the mutant allele could restore normal gene function, offering hope for patients with rare disorders.
Moreover, the integration of siRNA technologies into therapeutic frameworks has the potential to personalize medicine further. By tailoring siRNA to individual genetic profiles, treatments can be designed for optimal efficacy.
Challenges in Clinical Implementation
Despite the potential of siRNA as a therapeutic agent, several challenges impede clinical implementation. One primary concern is the delivery method. siRNA molecules are inherently unstable and can be degraded by nucleases in the bloodstream. Effective delivery systems must be developed to protect siRNA and facilitate its uptake by target cells.
- Delivery Vehicles: Current strategies are exploring various delivery vehicles such as lipid nanoparticles, viral vectors, and polymeric carriers to enhance stability and cell targeting. Each of these has its advantages and limitations, creating a need for tailored approaches depending on the application.
- Immunogenicity and Side Effects: siRNA can provoke an immune response, leading to undesired side effects. Avoiding such responses while ensuring that the therapeutic dose has a meaningful effect remains a significant hurdle for researchers.
- Regulatory Hurdles: Regulatory frameworks for siRNA therapeutics are still evolving. Navigating these regulations while ensuring that the designed compounds meet safety and efficacy standards requires substantial diligence and expertise.
"As siRNA technology progresses, addressing these challenges will be essential for translating laboratory success into clinical practice."
Ethical Considerations in siRNA Applications
The exploration of small interfering RNA (siRNA) technology brings with it a host of ethical considerations. This is critical in ensuring that the applications of siRNA design remain responsibly guided by moral and societal standards. Ethical concerns emerge primarily from the potential for genetic manipulation and the far-reaching consequences it may entail. Understanding these issues is essential, especially as siRNA technology drives advancements in gene silencing and therapeutic applications.
Concerns in Genetic Manipulation
The use of siRNA creates the possibility for unexpected genetic alterations. One primary concern is the off-target effects, where the designed siRNA might silence unintended genes. Such incidents can lead to harmful outcomes, risking both the integrity of the genome and the well-being of individuals involved in research or treatment.
Additionally, the potential for germline modifications heightens ethical stakes. If siRNA is used to edit genes in sperm or eggs, the changes would be passed down to future generations. This raises questions about consent, unforeseen health implications, and societal impact. People often debate who should have the authority to make such alterations and determine the future of human genetics.
Regulatory Framework and Compliance
A robust regulatory framework is crucial for addressing ethical concerns linked with siRNA applications. Governments and international organizations must set clear guidelines to manage, monitor, and enforce best practices in genetic manipulation. Current regulations may vary significantly across regions, underscoring the need for a standardized approach.
Key components of an effective framework include:
- Assessment of Risks: Identifying and evaluating the risks associated with siRNA technology.
- Public Engagement: Involving the public in discussions about genetic research promotes transparency and builds trust.
- Ethics Review Boards: Establishing independent boards to review research proposals ensures that ethical guidelines are adhered to.
Compliance with these regulations ensures that scientists and practitioners honor ethical standards, protecting individual rights and societal values while pushing scientific boundaries.
Future Directions in siRNA Research
The landscape of siRNA research is constantly evolving, presenting various potentials and challenges that researchers must navigate. Understanding future directions in siRNA research is essential, as it can guide strategic decisions for enhancing therapeutic applications and advancing scientific knowledge. The potential outlined in this section is pivotal not only for academics but also for industry professionals focusing on genetic therapies and molecular biology.
Innovations in siRNA Design Methodologies
Innovations in siRNA design methodologies continue to emerge, driven by technological advancements and the increasing need for precise gene targeting. Recent studies showcase the development of novel algorithms that optimize siRNA sequence selection. These algorithms utilize machine learning to predict off-target effects more effectively, which can significantly improve the specificity and efficacy of siRNA treatments. Current methodologies often incorporate metrics that assess the thermodynamic properties of siRNA duplexes, which can enhance their stability and performance in cellular environments.
New approaches such as microfluidic systems for high-throughput screening of siRNA libraries allow researchers to evaluate multiple sequences simultaneously. This accelerates the identification of the most effective siRNAs for particular target genes. Moreover, integrating CRISPR technology with siRNA has attracted attention for its potential to enhance gene silencing effects. These advancements signify a trend towards more sophisticated and efficient siRNA design methodologies that are aligned with the ongoing push for targeted therapeutic solutions.
Potential for Personalized Medicine
The integration of siRNA technology into personalized medicine represents a significant step toward tailoring treatments to individual patient profiles. The ability to design siRNA therapeutics based on a patient’s specific genetic makeup opens avenues for targeted therapies in various diseases, including cancer and genetic disorders. Personalized siRNA treatments could yield higher efficacy rates and fewer side effects, addressing a critical challenge in traditional medicine.
Furthermore, advancements in genomic sequencing technologies enable the construction of siRNA molecules targeted to unique mutations present in a patient’s genome. This customization offers potential avenues in oncology, where personalized siRNA can silence oncogenes specific to an individual’s tumor profile. The ongoing research in this domain is crucial as it encapsulates a shift towards precision medicine, where therapy is not just one-size-fits-all but rather tailored to maximize the outcome for each patient.
"The integration of siRNA technology into personalized medicine signifies a shift towards treatment modalities that respect patient individuality."
The End
The conclusion of this article underscores the significance of small interfering RNA (siRNA) design within the realm of gene silencing technology. As we have dissected throughout, the siRNA design process is not merely a technical endeavor but an intersection of biological understanding, experimental validation, and advanced computational tools. Each phase, from target gene selection to modifying chemical structures, plays a critical role in determining the effectiveness and efficiency of siRNA in various applications.
Summary of Key Points
In summary, the core elements of siRNA design discussed in this article include:
- The mechanism of siRNA and its role in RNA interference.
- The historical development that has shaped current methodologies.
- Critical considerations when selecting targets for siRNA, ensuring specificity and minimizing off-target effects.
- The importance of structural attributes that can enhance stability and efficacy.
- Detailed exploration of the common chemical modifications like 2'-O-methyl modifications that improve performance.
- A survey of existing design tools and algorithms, highlighting their strengths and practical uses.
- The necessity of rigorous experimental validation both in vitro and in vivo to confirm the anticipated outcomes.
The section also explored the implications of siRNA in functional genomics, therapeutic approaches, and the notable challenges faced during clinical implementation.
Final Thoughts on siRNA Design
In contemplating the future of siRNA design, it becomes evident that as technology advances, so too does the potential for more refined and targeted therapeutic strategies. The push towards personalized medicine is pivotal, ensuring that approaches cater not only to genetic variations but also to specific disease mechanisms.
As researchers and practitioners continue to innovate, the landscape of siRNA will evolve, leading to enhanced efficacy and broader applications. A collaborative effort involving researchers, regulatory bodies, and clinicians will be essential in navigating the complexities of ethical considerations and regulatory frameworks.
The journey of siRNA design illustrates the confluence of knowledge and technology, enabling breakthroughs in gene silencing that have the potential to transform healthcare and research.