From mRNA to Protein: Insights into Translation Mechanisms
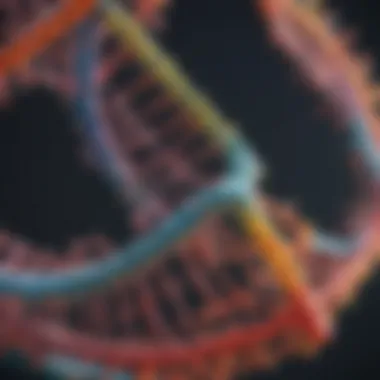
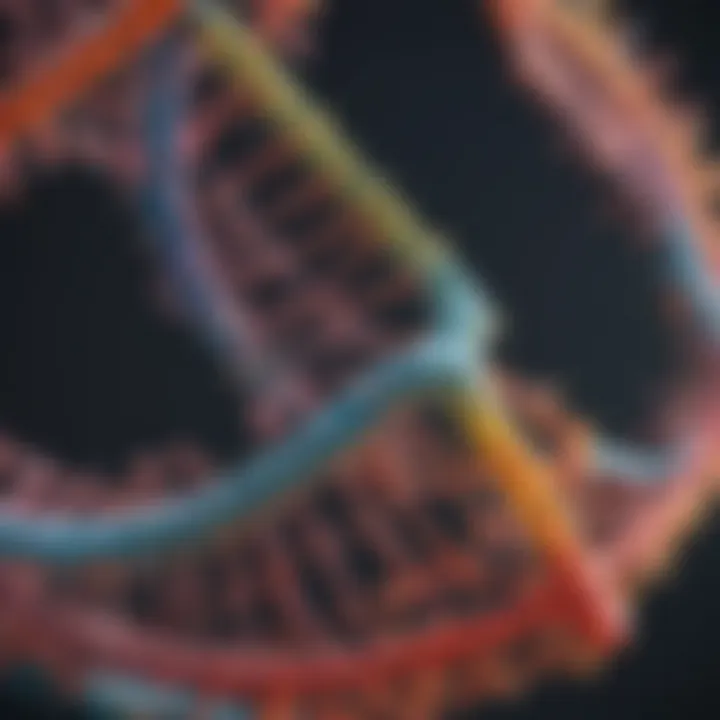
Intro
In the fascinating realm of biology, the translation process holds a pivotal position in the journey from genes to functioning proteins. At the heart of this process is messenger RNA, or mRNA, acting as the vital intermediary between DNA and proteins. When cells need to synthesize proteins, understanding how mRNA translates into a tangible product is essential.
The journey of mRNA is not just a simple read-off; it’s a structured and complex flow that involves various players—all of which perform distinct roles to ensure the accurate production of protein. The collaboration between ribosomes, transfer RNA (tRNA), and the precise stages of initiation, elongation, and termination make this process remarkable.
But why does this matter? The insights gained from studying the translation process extend far beyond academic curiosity. They pave the way for advancements in medical science, particularly with the rise of mRNA technologies in vaccines and treatments. As we navigate through the intricacies of how mRNA becomes protein, we uncover not only the mechanics involved but also the broader implications for health and disease.
This article is designed to provide a comprehensive overview, blending fundamental scientific principles with contemporary applications, while aiming to inform students, researchers, and professionals alike.
Prologue to mRNA and Protein Synthesis
The journey of mRNA to protein is a cornerstone of molecular biology, pivotal in the understanding of how genetic information is translated into functional entities. This translation process is essential for not only the development of proteins but also for regulating biological functions that sustain life itself. Comprehending mRNA's role allows researchers to uncover the nuances of gene expression, impacting fields like genetics, biotechnology, and medical science.
In the modern landscape of genetics, mRNA molecules serve as vehicles, carrying encoded instructions from DNA to ribosomes, which are the cellular machinery responsible for protein synthesis. Without mRNA, these instructions would remain locked within the nucleus, rendering much of life's complexity inoperative. Thus, understanding mRNA is not just an academic exercise; it’s a key that opens doors to innovations in treatments and therapy.
There are several aspects to consider regarding mRNA and protein synthesis:
- Basic Functionality: Knowing how mRNA facilitates the translation of genetic code into proteins offers insight into cellular processes.
- Regulatory Mechanisms: The ways in which mRNA is modified and regulated can greatly influence health and disease.
- Medical Implications: With the rise of mRNA technologies, such as vaccines, understanding this process has never been more relevant.
The following sections of this article will delve into the genetic code, the role of mRNA, and the intricate machinery that executes translation. By grasping the basic principles of mRNA's functionality, one can appreciate its central role in biology and the potential it holds for future medical advancements.
The Translation Machinery
Understanding the translation machinery is crucial for grasping how proteins are synthesized from mRNA. The translation machinery encompasses the cellular components that work together seamlessly to facilitate this complex process. At the heart of this machinery are ribosomes and transfer RNA (tRNA), both of which play vital roles in interpreting the genetic instructions and constructing proteins accordingly.
Ribosomes are often likened to factories where the actual assembly of proteins occurs, reading the mRNA and assembling amino acids in the correct order. Meanwhile, tRNA serves as an important go-between, bringing specific amino acids to the ribosome as dictated by the mRNA sequence. This collaboration is critical; without them functioning properly, the translation process would come to a grinding halt, leading to inefficient protein production.
The efficiency of translation hinges on several factors, including the integrity of the mRNA, the availability of tRNA, and the ribosomes' functionality. Any disruption in these components can significantly affect protein synthesis, potentially leading to dysfunctional proteins or cell malfunction. Thus, a deep understanding of the translation machinery not only impacts basic biology but also has far-reaching implications in fields such as genetics, molecular biology, and medicine.
Ribosomes: The Site of Protein Synthesis
Ribosomes can be thought of as the workhorses of the cell, responsible for synthesizing proteins by reading the sequences presented by mRNA. Found either floating freely in the cytoplasm or attached to the endoplasmic reticulum, these small but mighty structures are composed of ribosomal RNA (rRNA) and proteins, making them complex entities.
At their core, ribosomes consist of two subunits: the large subunit and the small subunit.
- Small Subunit: This part binds to the mRNA strand and is responsible for decoding its sequence. It ensures that the ribosome correctly matches the tRNA carrying the corresponding amino acid to the codons on the mRNA.
- Large Subunit: This component catalyzes the peptide bond formation between amino acids, enabling the polypeptide chain to grow and eventually fold into a functional protein.
The synergy between these subunits is essential for accurate translation. The ribosome essentially acts as a sophisticated reading machine that interprets the genetic code gleaned from the mRNA.
"Ribosomes showcase a unique blend of simplicity and complexity, translating the language of mRNA into the universal language of proteins."
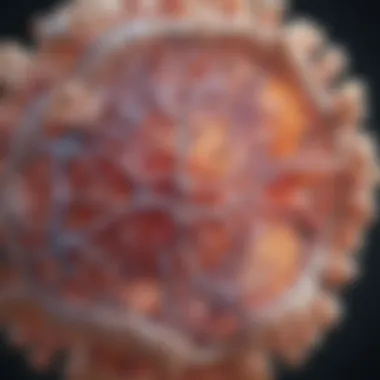
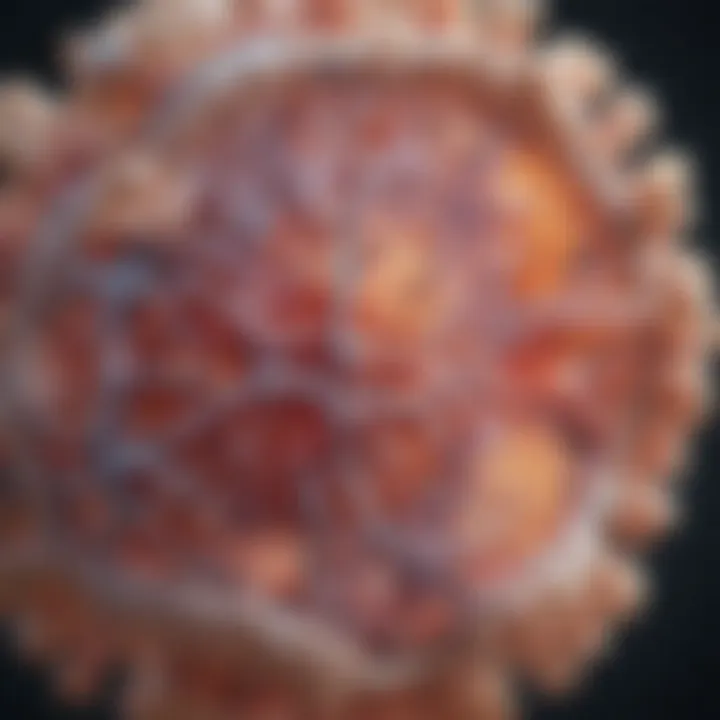
tRNA: Adaptor Molecules
tRNA, or transfer RNA, serves as the vital link between mRNA's codons and the corresponding amino acids. Imagine tRNA as delivery vehicles, where each one is tailored to carry a specific type of amino acid. Each tRNA molecule has an anticodon region that matches with a specific codon on the mRNA strand, ensuring that the right amino acid is transported to the ribosome.
To elaborate, the structure of tRNA is designed to facilitate this role effectively:
- Anticodon: A set of three nucleotides that base pairs with the complementary codon on the mRNA, ensuring the correct amino acid is added to the growing chain.
- Amino Acid Attachment Site: Located on the opposite end of the anticodon, this site is where the specific amino acid is linked. This atomically precise matching is what enables the ribosome to construct proteins accurately.
Thanks to this system, each tRNA is uniquely associated with one specific amino acid, allowing for the accurate reading of the mRNA code and the proper assembly of amino acids into a polypeptide chain. It’s this adaptability of tRNA that makes the translation process both flexible and highly accurate, ensuring that proteins are synthesized correctly to perform their many diverse functions in the cell.
Stages of Translation
Understanding the stages of translation is like getting the blueprint for a complex building. It's through these stages—initiation, elongation, and termination—that mRNA coding is turned into functional proteins. Each stage plays a vital role, ensuring that the final product is not just a random assembly but a precise construction of amino acids that form the polypeptide chain.
Translation is not just a casual process; it’s dynamic and highly regulated. Any hiccup here can lead to disastrous outcomes, such as malfunctioning proteins which could cause a host of diseases. Knowing the stages helps in grasping medical advances that utilize this fundamental process, especially in developing therapies like mRNA vaccines. The insights gained can help us optimize protein synthesis in both research and medical contexts, making it a crucial area of study.
Initiation: Starting the Translation
In the initiation phase, a complex assembly gets to work, ensuring that the mRNA is ready to be translated. This is where the Formation of the Initiation Complex kicks into gear. It lays the groundwork for the whole translation process.
Formation of the Initiation Complex
The formation of the initiation complex is where it all begins. This complex is made up of the ribosome, the mRNA strand, and several proteins and factors crucial for the process. This stage ensures that everything binds correctly, setting the stage for smooth sailing ahead. The key characteristic here is precision; the ribosome must attach to the start codon on the mRNA with absolute accuracy.
What’s interesting is that this stage is like a well-rehearsed theater performance; it needs all players to be in sync. If anything goes awry—like using the wrong initiation factors—the complex may not form properly, leading to inefficiencies or errors.
Advantages: A properly formed initiation complex allows for efficient translation continuation. This makes it beneficial for our understanding as we can draw connections to various cellular mechanisms affecting health and disease.
Role of Initiator tRNA
Now let’s shine a spotlight on the role of initiator tRNA. This tRNA carries a methionine amino acid and is central to kicking off the whole translation process. It’s unique in serving as the first tRNA molecule to bind to the mRNA. Its job is not just to show up; it actively recognizes the start codon, ensuring the ribosome knows where the protein-making begins.
The critical element here is equivalent to having the right key to unlock the door. If the initiator tRNA doesn't pair with the right codon, translation can’t properly kick off. Advantages: This specialized molecule allows the process to start on the correct foot, further enhancing the accuracy of protein synthesis.
Elongation: Synthesizing the Polypeptide Chain
Once initiation is successfully completed, the spotlight shifts to elongation, where the actual synthesis of the polypeptide chain takes place. Here, we see a remarkable interplay of ribosomal movement and peptide bond formation.
Ribosomal Movement
Ribosomal movement is crucial during elongation. As tRNA molecules, each bearing specific amino acids, bind to the mRNA, the ribosome travels along the mRNA strand. This movement is a well-oiled machine, almost like a conveyer belt that adds amino acids to the growing chain with each step.
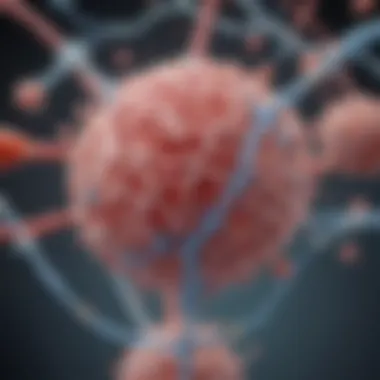
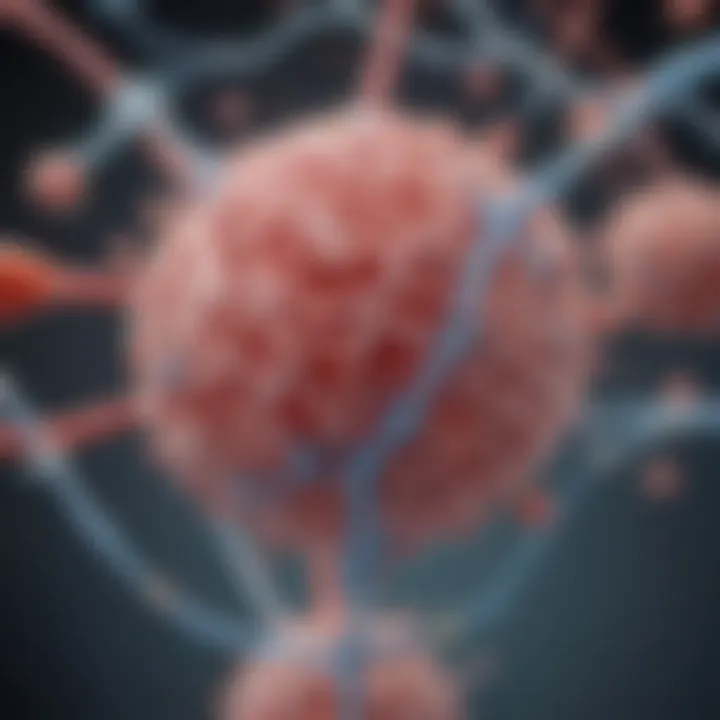
A standout feature of this process is that the ribosome shifts one codon at a time. That gradual progression is beneficial; it allows for careful monitoring of tRNA interactions, ensuring that only the correct amino acids are linked together. This carefully coordinated movement ensures that proteins are formed accurately, allowing cells to maintain functionality.
Peptide Bond Formation
Peptide bond formation is the heart of elongation. Each time a ribosome shifts, it catalyzes the formation of a peptide bond between the amino acids supplied by tRNA. This stage is what ultimately links each amino acid together to form a polypeptide chain.
What’s fascinating here is the efficiency of this process. Ribosomes can link amino acids at an astonishing speed, enabling rapid protein synthesis, which is essential for cell function. However, if any errors occur during this phase, it could drastically affect the resultant protein's functionality, leading to misfolded or dysfunctional proteins. This brings in importance of proofreading mechanisms that keep the process in check.
Termination: Concluding the Translation
The last stage, termination, is where the translation process concludes, and it's just as important as the previous stages. This is where everything wraps up, involving stop codons and release factors.
Stop Codons
Stop codons act as the signals for termination, instructing the ribosome to stop translating once a full polypeptide chain has been synthesized. This signal occurs at the end of the mRNA sequence and can be recognized by the ribosomal apparatus. A noteworthy feature of stop codons is that they do not have corresponding tRNA. Instead, they prompt the assembly of other factors that mediate the release of the completed protein.
Advantages: Understanding stop codons sheds light on how proteins are synthesized accurately. Their action signifies the end of a translation, much like period in a sentence completes a thought.
Release Factors
Release factors are proteins that recognize stop codons and facilitate the disassembly of the ribosome and mRNA once the protein is fully formed. These factors play a critical role; without them, proteins would remain attached to ribosomes, resulting in a backlog.
Their unique feature is this crucial recognition and facilitation role, which ensures proteins can be appropriately released and made available for cellular functions. However, if there are issues with release factors, it could mean a serious delay in protein turnover, negatively impacting both cellular health and organismal physiology.
In summary, the stages of translation are not just separate entities, but rather, interdependent processes that culminate in the production of vital proteins, underscoring their significance in the greater context of genetics and molecular biology.
Factors Affecting Translation
The process of translation is not merely a linear path from mRNA to protein; rather, it’s a complex journey influenced by various factors that can either promote or impede the efficiency and accuracy of protein synthesis. Understanding these factors is crucial for anyone delving into molecular biology, as they shed light on how cells regulate gene expression and respond to environmental cues. The significance of these elements in protein synthesis cannot be overstated, given their implications for both basic research and applied sciences such as biotechnology and medicine.
Influence of mRNA Structure
The structure of mRNA plays a pivotal role in the translation phase. mRNA is much more than just a linear string of nucleotides; it possesses intricate features that can greatly influence how effectively ribosomes can attach and read the genetic code.
One of the primary considerations is the length of the 5' untranslated region (5' UTR) and the presence of secondary structures. A longer 5' UTR can present a barrier for ribosome recruitment, potentially slowing down the initiation of translation. Moreover, the formation of secondary structures, such as hairpins, can further complicate access to the ribosomal binding site, leading to variability in protein expression.
- Codon Usage: The specific sequence of codons within the mRNA can also sway the efficiency of translation. Some codons are more frequently used than others, which can affect the availability of corresponding tRNAs, thereby influencing the translation rate and fidelity.
- Poly(A) Tail: The poly(A) tail at the 3' end of mRNA not only stabilizes the molecule but also aids in the recruitment of translation initiation factors. A longer poly(A) tail often correlates with higher rates of translation, as it plays a role in the circularization of mRNA, facilitating interactions between the cap and the tail.
Such nuances in mRNA structure are critical in dictating how a gene is expressed in a given cellular context, making it a fundamental aspect of translation.
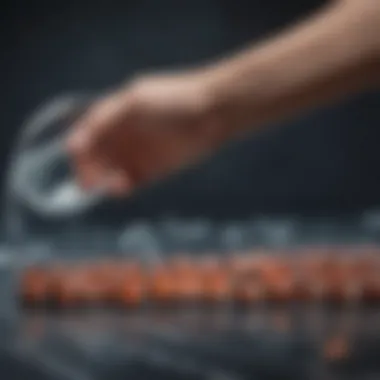
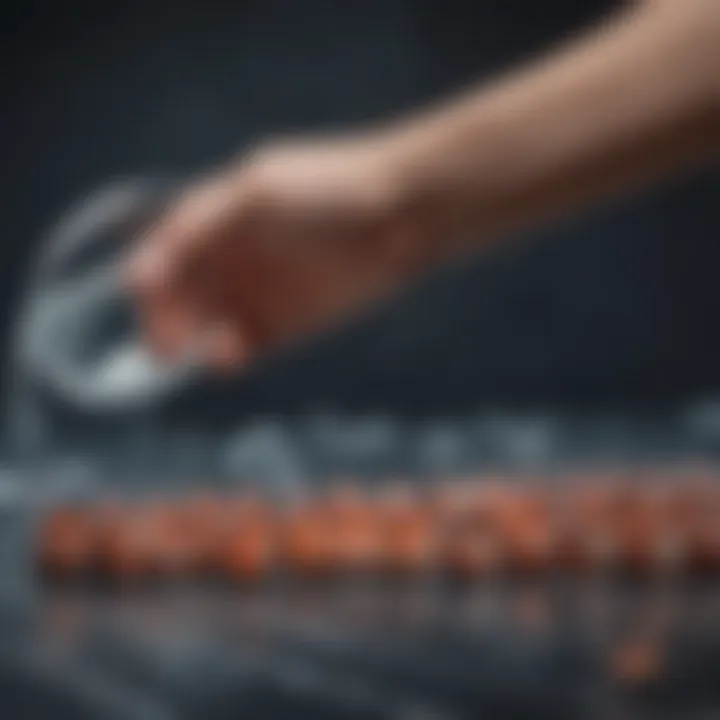
Role of Translation Factors
Translation factors, a diverse group of proteins, also bear a substantial influence on the translation process. They assist in various stages, from initiating the assembly of ribosomes to ensuring accurate peptide bond formation throughout elongation.
- Initiation Factors: These proteins are fundamental in forming the initiation complex. They help load the small ribosomal subunit onto the mRNA, facilitating the correct pairing of the initiator tRNA with the start codon. The efficiency of this process directly impacts how quickly a translation begins.
- Elongation Factors: Once translation is underway, elongation factors come into play, catalyzing the sequential addition of amino acids to the growing polypeptide chain. They enhance the ribosomal movement along the mRNA and ensure that tRNAs deliver the correct amino acids as dictated by the mRNA sequence.
- Termination Factors: Finally, termination factors are essential for the release of the newly synthesized protein from the ribosome. They recognize stop codons and facilitate the disassembly of the translation machinery, marking the end of the synthesis process.
Moreover, the presence or absence of certain translation factors can dictate how well a cell can adapt to stress conditions. For instance, under nutrient deprivation, cells may downregulate some factors to conserve resources, leading to a decrease in overall protein synthesis. In contrast, during times of nutrient abundance, the upregulation of these factors can boost protein production significantly.
mRNA Technologies in Medicine
mRNA technologies mark a significant evolution in the field of medical science. Historically, vaccine development was a time-consuming process, relying on weakened or inactivated pathogens. However, mRNA approaches have flipped this script entirely. These technologies offer a swift, flexible, and efficient platform for crafting vaccines and therapeutic agents. This transformation is particularly visible in how we can respond to emerging infectious diseases—like the rapid development of COVID-19 vaccines. By deciphering the genetic instructions of a pathogen and transcribing them onto mRNA, scientists can teach the immune system how to recognize and combat the virus without ever exposing it to the actual pathogen itself.
Moreover, mRNA technologies extend their reach beyond just vaccines. They hold potential for treating a variety of diseases that include cancer, genetic disorders, and other viral infections. The adaptability of mRNA allows it to be customized for various targets, paving the path for personalized medicine. As we delve into the details of this groundbreaking approach, it becomes evident that mRNA has a role that goes far beyond current applications, hinting at a future filled with possibilities.
mRNA Vaccines: A Paradigm Shift
The arrival of mRNA vaccines has indeed represented a paradigm shift in immunization strategies. Unlike conventional vaccines, which often require the use of live or killed viruses, mRNA vaccines instruct cells to produce a harmless piece of the spike protein found on the surface of the target virus—such as SARS-CoV-2. This protein piece is enough for the immune system to recognize and remember, thereby eliciting a robust immune response when faced with the actual virus.
- Speed of Development: The design of mRNA vaccines can be rapidly modified, enabling swift responses to outbreaks.
- Safety Profile: Since these vaccines do not contain live virus particles, there is no risk of getting the disease from the vaccine itself.
- Strong Immune Response: Studies have shown that mRNA vaccines can produce strong immune responses, even after a single dose in some cases.
- Education of the Immune System: mRNA serves as a form of biological education, teaching the immune system in a way that traditional vaccines often struggle with.
"The promise of mRNA technology lies in its simplicity and adaptability, representing a new frontier in vaccine development that could change the way we approach infectious diseases."
Therapeutic Applications Beyond Vaccines
The horizon of mRNA technology does not end with vaccines; numerous therapeutic applications are being explored and developed. Perhaps one of the most enticing aspects is its potential in cancer treatment. Oncogenic mRNA can be designed to instruct the body’s immune system to attack cancer cells specifically, providing a targeted approach in contrast to traditional therapies like chemotherapy, which affect both cancerous and healthy cells.
In addition to oncology, mRNA is showing promise in treating various genetic disorders. Here are a few ways mRNA is being utilized:
- Protein Replacement Therapy: For conditions caused by insufficient protein production, mRNA can be engineered to supplement the missing proteins.
- Autoimmune Diseases: mRNA could help recalibrate the immune system to reduce inappropriate responses characteristic of autoimmune conditions.
- Rare Genetic Disorders: Harnessing mRNA could allow tailored treatments for rare diseases that presently have limited options.
The beauty of mRNA technology lies in its versatility and potential to customize treatments to an individual's specific needs. As research progresses, we may see mRNA therapies becoming part of standard medical practices, potentially transforming lives where current treatments have failed.
As this field continues to grow, the long-term implications of mRNA technologies can be profound, not just for public health, but for how we conceive of and treat illnesses at the molecular level.
Ending
The translation process was explored thoroughly in this article, and it is clear that understanding mRNA's role in protein synthesis is not just crucial for students and researchers but has profound implications in various fields including medicine. The essence of this process lies in its complexity and precision, illustrating a meticulous ballet of biological molecules at work.
By dissecting the stages of translation—initiation, elongation, and termination—along with the roles of ribosomes and tRNA, we can appreciate how life itself hinges on these fundamental mechanisms. Each step is not just a cog in a machine, but a unique interplay of biochemical forces that either contribute to health or, if disrupted, can lead to disease.
The benefits of grasping this process extends beyond academic knowledge. It fosters an understanding of genetic diseases, informs drug development, and enhances advancements in genetic engineering. You can't underestimate how hints at translational efficiency can lead to breakthroughs in therapies, particularly in treating conditions previously deemed untouchable.
In looking to the future, the ongoing research into mRNA's potential holds great promise. With mRNA vaccines leading the way, as we discussed earlier, the applications will likely expand further, intertwining with various therapeutic avenues. The dialogue around ethics, accessibility, and effectiveness will become increasingly pertinent.
"Understanding the processes of life at the molecular level enables us to not only unveil the mysteries of biology, but to unlock the potential for innovative therapies and medical advancements."
As we move ahead, keeping tabs on future developments in mRNA research will be essential. The possibilities are vast, descending from minuscule cellular processes to broad, tangible impacts on human health and disease management. This exploration not only enriches our knowledge but also underscores the importance of continued investment in scientific research.