Understanding the Mechanism of PET Scanners
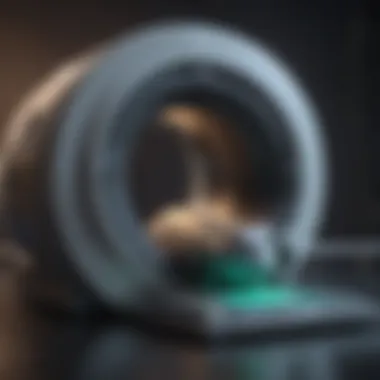
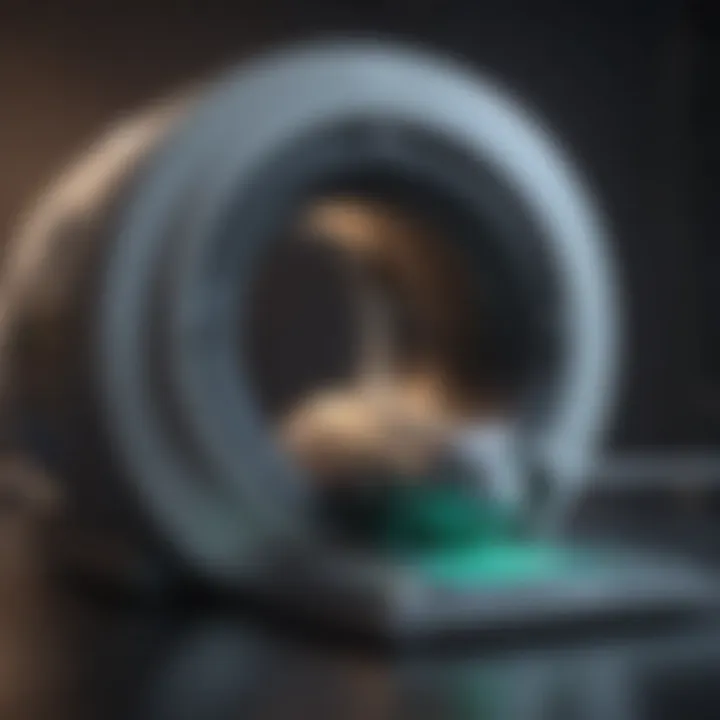
Intro
Positron Emission Tomography (PET) scanners represent one of the most significant advancements in medical imaging technology. Through the use of radioactive tracers, PET scanners provide a unique insight into metabolic processes of the body. This ability to visualize physiological functions stands in contrast to traditional imaging techniques like X-rays or CT scans, which predominantly focus on anatomical structures. Understanding the mechanisms that drive PET technology is crucial for students, researchers, and professionals who seek to leverage this technique in clinical and research settings.
Research Overview
Summary of Key Findings
The operation of PET scanners is based on the principles of nuclear physics. When a radionuclide decays, it emits positrons. These positrons, when they encounter electrons in the body, annihilate each other, resulting in the emission of gamma rays. The detection of these gamma rays is what enables the generation of images. This section will expand on the method and technology behind the imaging process.
Relevance to Current Scientific Discussions
PET scans have become integral in various fields, including oncology, cardiology, and neurology. The burgeoning knowledge around tracer development and imaging techniques is essential to advancing the diagnostic accuracy and effectiveness of therapies. Current discussions also focus on enhancing the safety of PET scans through dose reduction methods and considering the ethical implications of using radioisotopes in research and clinical practice.
Mechanism of Action
PET technology involves several steps:
- Tracer Production: Tracers are compounds labeled with a radioactive isotope. The most commonly used tracer is fluorine-18, linked to glucose, which plays a vital role in cellular metabolism.
- Injection into the Patient: The tracer is injected into the patient, allowing it to circulate and accumulate in tissues that exhibit high levels of metabolic activity, such as tumors.
- Emission of Positrons: After a certain period, the radioactive tracer begins to decay, emitting positrons.
- Annihilation and Gamma Ray Detection: When positrons meet electrons, they produce gamma rays that travel in opposite directions. Detectors within the PET scanner capture these gamma rays.
- Image Reconstruction: Sophisticated algorithms reconstruct the detected signals into detailed images showcasing the distribution of the tracer and providing insights into metabolic activity.
Methodology
Research Design and Approach
A comprehensive understanding of the PET scanner mechanism requires both theoretical and practical examination. This involves reviewing existing literature to outline major technological advancements and their impact on medical practice.
Data Collection and Analysis Techniques
Data on the effectiveness of PET scans in various medical fields is gathered from peer-reviewed articles, clinical trials, and case studies. This two-pronged approach ensures that current knowledge is accurately represented and incorporated into future applications of PET technology.
Prelude to PET Scanners
Positron Emission Tomography (PET) represents a significant leap in medical imaging. It plays a crucial role in diagnosing various conditions by providing insights into metabolic activity in tissues. Understanding PET scanners is essential because they help clinicians make informed decisions based on precise imaging data. This section will clarify what PET scanners are, their purposes, and how they developed over time.
Definition and Purpose
A PET scanner is a sophisticated imaging device used primarily in the medical field to visualize metabolic processes in the body. By detecting gamma rays emitted from positron annihilation, it creates detailed three-dimensional images of biological functions. The primary purpose of these scanners is to aid in the diagnosis, staging, and monitoring of diseases, particularly cancer.
PET imaging is invaluable because it allows physicians to see not just structural abnormalities but also the physiological changes associated with disease states. This can inform treatment plans and improve patient outcomes. The utilization of specific radiotracers, which emit positrons, enables the visualization of particular organs and systems, thereby enhancing diagnostic precision.
This imaging technique serves several critical functions:
- Diagnosis of diseases: PET scans help identify conditions such as cancer, neurological disorders, and cardiac diseases.
- Treatment monitoring: Ongoing assessments can show how well a treatment is working over time.
- Research applications: In clinical trials, PET plays a role in understanding disease mechanisms and evaluating new therapies.
Historical Development
The history of PET scanners is a tale of innovation and interdisciplinary collaboration. The roots of PET technology trace back to the mid-20th century when the foundations of nuclear medicine were laid. The concept of using radioactive isotopes in medical diagnostics emerged in the 1950s, gradually paving the path for more refined techniques.
In 1974, the first PET scanner was developed by Michael E. Phelps and his colleagues at Washington University, marking a significant milestone. This early device allowed for the measurement of biological processes, setting the stage for subsequent advancements. Over the next few decades, improvements in detector technology and computational capabilities led to enhancements in image quality and resolution.
Today, PET scanners have become more advanced, integrating with other imaging modalities such as CT and MRI. The fusion of technologies provides comprehensive datasets, allowing clinicians to derive more meaningful insights. The historical progression reflects ongoing research and development efforts aimed at improving diagnostic capabilities and expanding the potential applications of PET in medicine.
Basic Principles of Positron Emission Tomography
Positron Emission Tomography, commonly referred to as PET, serves as a crucial technique in the realm of medical imaging. Understanding the basic principles of PET is essential to grasp how this technology functions and why it is so effective in diagnosing various health conditions. PET enables clinicians to visualize physiological processes within the body, thus providing vital information that traditional imaging techniques may miss. This section delves into the core fundamentals of positron emission and the production of radioisotopes, both of which are integral to the function of PET scanners.
Fundamentals of Positron Emission
The premise of positron emission is fundamentally linked to the behavior of certain unstable atomic nuclei. These nuclei undergo decay, releasing positrons—positively charged counterparts to electrons. When these emitted positrons encounter electrons, annihilation occurs, producing a pair of gamma photons. The detection of these photons is essential to construct an image of metabolic activity in tissues.
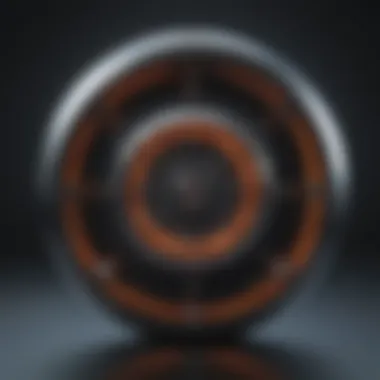
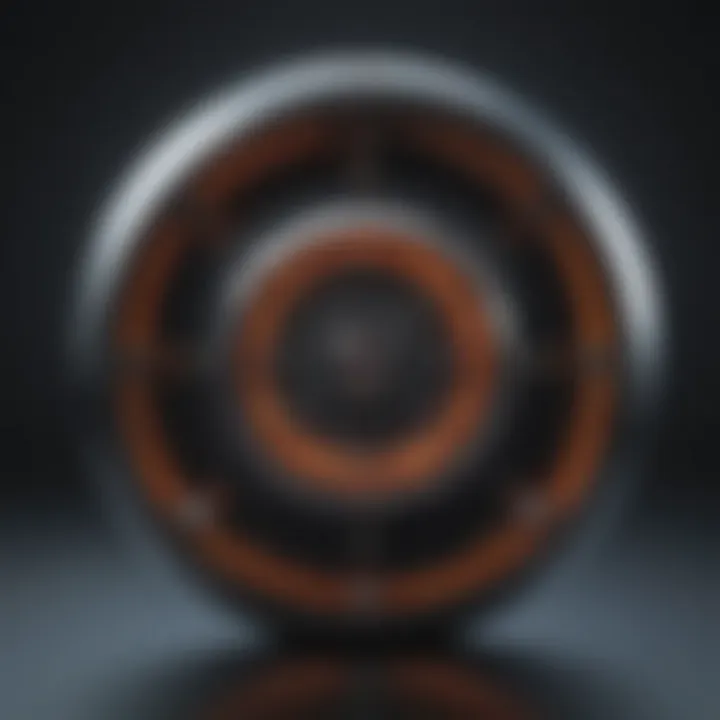
The significance of this process lies in its ability to monitor biochemical processes in real-time. For instance, when a radiotracer, such as fluorodeoxyglucose (FDG), is introduced into the body, it will accumulate in metabolically active tissues, such as tumors. The resulting annihilation events can then be detected by the PET scanner. This reaction is central to the ability of PET to provide insights into how actively tissues are using glucose, a key source of energy.
In addition, positron emission provides information regarding the spatial distribution of radiotracers throughout the body. This helps in identifying any abnormal physiological activity, assisting healthcare professionals in accurate diagnoses and treatment planning.
Radioisotope Production
Radioisotopes are the cornerstone of positron emission tomography. They are produced in cyclotrons or generators and serve as the radiotracers for PET scans. The production process involves creating isotopes that emit positrons during their decay. Commonly used radioisotopes include fluorine-18, carbon-11, and nitrogen-13.
- Fluorine-18: Typically produced in a cyclotron, fluorine-18 has a half-life of approximately 110 minutes, making it a preferred choice for clinical applications.
- Carbon-11: This isotope has a shorter half-life (20 minutes) and is often used to label compounds for very specific imaging purposes.
- Nitrogen-13: With a half-life of about 10 minutes, nitrogen-13 is also utilized for real-time studies of the brain and other organs.
The selection of a particular radioisotope often depends on factors such as half-life, type of decay, and the biological behavior of the resulting radiotracer. The careful planning and execution of radioisotope production is essential to ensure high-quality imaging with PET scanners.
"The meticulous selection and production of radioisotopes determine the accuracy and effectiveness of PET imaging in clinical settings."
Understanding these principles forms the foundation for realizing how PET technology bridges the gap between nuclear medicine and diagnostic imaging. In the following sections, we will explore the intricate working mechanisms of PET scanners.
The Working Mechanism of PET Scanners
Understanding the workings of PET scanners is crucial in comprehending how these advanced imaging tools contribute to modern medical diagnostics. The efficiency and effectiveness of PET technology hinge on its operational principles. This section outlines the intricate layers of image acquisition, data processing, and image reconstruction which are essential components in generating high-quality images. Emphasizing the synergy between these elements helps elucidate the overall mechanics of PET imaging, enhancing its significance in clinical application.
Image Acquisition Process
The image acquisition process in PET scanning is fundamental to gathering the necessary data for analysis. It begins with the administration of a radiotracer, which contains a positron-emitting isotope. As the tracer travels through the body, it accumulates in areas of high metabolic activity, often indicating disease.
When these isotopes decay, they emit positrons that travel short distances before encountering electrons in the body. The annihilation of these particle pairs generates gamma rays, which are directed outward. Detectors surrounding the patient capture these gamma rays, converting them into electrical signals.
The signal detection is critical. Precision in this step affects image quality. Key factors include:
- Time of Flight: This technique improves the accuracy of locating the annihilation events by measuring the time it took for gamma rays to reach detectors.
- Detector Material: Common materials like bismuth germanate (BGO) and lutetium oxyorthosilicate (LSO) are used for their effective gamma-ray absorption.
- Scanner Geometry: The arrangement of detectors plays a role in optimizing the imaging field of view.
Data Processing Techniques
Data processing after image acquisition involves multiple steps to transform raw signals into interpretable images. The primary focus lies in filtering, correction, and compression of the acquired data.
A critical technique is the application of scatter correction, which addresses inaccuracies due to scattered photons not originating from the point of interest. Attenuation correction is also vital, as it accounts for the varying absorption of signals by different tissues. These corrections ensure that the final images are as true to reality as possible.
Furthermore, the processing software analyzes the data to enhance clarity and contrast. Employing sophisticated algorithms, it handles:
- Noise Reduction: To remove irrelevant information and improve image fidelity.
- Normalization: Standardizing the effects of varying detector response.
- Dynamic Imaging: Capturing changes over time, revealing metabolic processes.
Image Reconstruction Algorithms
Once the data is processed, the next phase is image reconstruction. Several algorithms are utilized to convert the processed data into coherent images. Among these, the most common are:
- Filtered Back Projection (FBP): A traditional, simpler method used for its efficiency but may not always deliver the best quality.
- Iterative Reconstruction: This advanced technique, although more computationally intense, often produces higher-quality images by refining estimates progressively. It improves on FBP by addressing artifacts and statistical noise.
- Maximum Likelihood Expectation Maximization (MLEM): An advanced form of iterative reconstruction that maximizes the statistical likelihood of the data, further enhancing image quality.
"Effective image reconstruction transforms raw data into meaningful diagnostics, directly impacting clinical decisions."
Each algorithm has its own strengths and weaknesses, and the choice of method can influence diagnostic accuracy. With ongoing advancements in technology, the efficiency and effectiveness of image reconstruction algorithms continue to evolve.
Technological Framework of PET Scanners
The technological framework of PET scanners encompasses the underlying systems and processes that facilitate the effective operation of this imaging modality. Understanding this framework is essential for appreciating how PET scans produce detailed images that inform medical diagnoses. The interplay of various components in the framework not only defines the functionality of PET technology but also contributes to its growing significance in clinical settings.
Detector Systems
Detector systems are one of the core elements within the technological framework of PET scanners. These systems are responsible for capturing the gamma photons emitted during positron annihilation. Common types of detectors include scintillation detectors and semiconductor detectors. Each type has unique characteristics.
- Scintillation Detectors: Uses materials that emit light when gamma rays hit them. The light produced is then converted into electrical signals.
- Semiconductor Detectors: Based on materials such as germanium and silicon, these detectors offer higher resolution and faster response times.
The efficiency and accuracy of these detector systems significantly influence image quality. Proper calibration and maintenance of detectors ensure optimal performance, which reduces the likelihood of artifacts in the imaging results. The advancements in detector technology are essential for improving sensitivity and resolution in imaging.
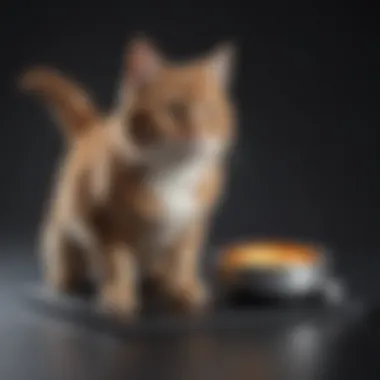
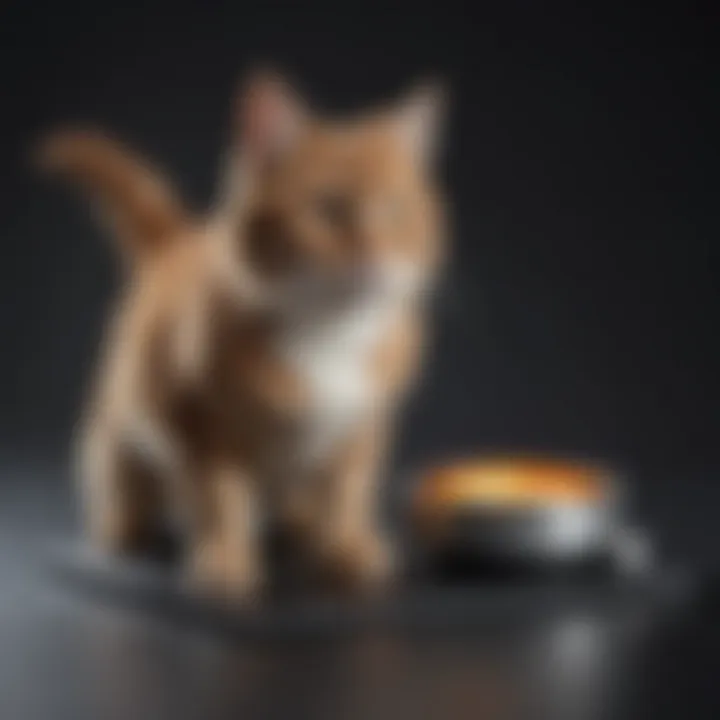
Software and Imaging Techniques
In conjunction with hardware components, software plays a pivotal role in the operation of PET scanners. Advanced software algorithms enable the processing and visualization of complex data obtained from the imaging procedure. Some critical aspects include:
- Image Reconstruction Algorithms: These algorithms, such as filtered back projection and iterative reconstruction, convert raw data into interpretable images. The choice of algorithm can affect both the speed and quality of the images produced.
- Analysis Software: Tools for analyzing PET images help clinicians assess areas of interest more effectively. These tools are essential for identifying pathologies and making informed decisions regarding treatment.
Moreover, integration with other imaging modalities, such as CT and MRI, is becoming more prevalent. This synergy enhances diagnostic capabilities as it allows for a better anatomical and functional correlation.
The accuracy of PET imaging lies not only in the hardware but significantly in the sophistication of the software used for data interpretation. This interplay is crucial for effective diagnostics.
To summarize, the technological framework of PET scanners, including both detector systems and imaging software, establishes the foundation for the detailed imaging provided by this diagnostic tool. Understanding these components allows a greater appreciation for the advancements made and the potential future improvements that can arise in this high-impact field.
Applications of PET Scanning in Medicine
Positron Emission Tomography (PET) scanners have revolutionized medical imaging by providing functional insights into various biological processes. This section will explore the significant applications of PET scanning in clinical settings, particularly in oncology, neurology, and cardiology. Understanding these applications offers vital context for how PET technology aids in diagnostics, treatment planning, and patient management.
Oncology Utilization
PET scanning plays a crucial role in oncology. It helps in the diagnosis, staging, and monitoring of various cancers. The technique utilizes fluorodeoxyglucose (FDG), a radiolabeled glucose analog. Cancer cells typically exhibit increased glucose metabolism compared to normal cells. Thus, PET scans can detect tumors that show higher FDG uptake. In this way, oncologists can locate malignancies earlier, potentially leading to more effective intervention.
Key aspects of PET in oncology include:
- Staging of Cancer: PET scans can determine the extent of cancer spread, which helps in deciding treatment strategies.
- Monitoring Response to Treatment: After initiating therapy, PET can evaluate how well a patient is responding to treatment, allowing modifications if necessary.
- Detecting Recurrence: Follow-up PET scans are critical in identifying the return of cancer earlier than conventional imaging.
Neurology Insights
In neurology, PET scanning provides valuable insights into brain function. It helps in the diagnosis and management of conditions such as Alzheimer's disease, epilepsy, and brain tumors. The ability of PET to visualize metabolic processes in the brain allows for functional assessments, which is particularly important in degenerative conditions where structural changes may not yet be evident.
Some points of significance in neurology include:
- Alzheimer's Disease: PET can show patterns of glucose metabolism that correlate with cognitive decline, aiding early diagnosis.
- Epilepsy Evaluation: The technology helps identify seizure foci, which is vital for determining surgical options.
- Brain Tumor Assessment: PET is useful in differentiating between tumor recurrence and radiation necrosis, guiding therapeutic decisions.
Cardiology Assessments
Cardiology is another field significantly benefiting from PET scanning. It allows for detailed assessment of coronary artery disease and myocardial viability. By using radiotracers that measure blood flow and metabolism in the heart muscle, PET helps in evaluating the functional capacity of the heart.
Important uses in cardiology include:
- Ischemic Heart Disease: PET is used to assess myocardial perfusion and detect areas of decreased blood flow.
- Viability Studies: It can determine whether heart tissue is viable and can recover post-reperfusion, influencing treatment decisions.
- Risk Stratification: Accurate risk assessments can be made regarding potential cardiac events in patients with known heart conditions.
"The applications of PET scanning in oncology, neurology, and cardiology showcase its multifaceted utility in modern medical diagnostics."
In summary, PET scanning is indispensable in various medical fields. Its applications facilitate early diagnosis, enhance treatment planning, and improve patient outcomes. As technology advances, the role of PET in medicine is likely to widen, potentially introducing new applications that can revolutionize patient care.
Comparison with Other Imaging Modalities
In the realm of medical imaging, various modalities exist, each with unique strengths and clinical utility. Understanding the comparisons between these techniques is crucial for healthcare professionals targeting optimal diagnostic outcomes. This section will focus on the nuances of Positron Emission Tomography (PET) in relation to other common imaging methods such as Computed Tomography (CT) and Magnetic Resonance Imaging (MRI). Evaluating these methods will provide deeper insight into their collaborative potentials, ultimately enhancing patient care.
PET vs. CT Imaging
PET and CT are frequently employed in tandem to offer a more comprehensive view of medical conditions. While CT uses X-rays to produce detailed images of the body's interior structures, PET measures metabolic activity through radiopharmaceuticals. This fundamental difference leads to distinct advantages:
- Structural vs. Functional Insights:
CT is excellent for identifying structural abnormalities like tumors or fractures, whereas PET provides functional insights indicating diseases through metabolic changes. - Combined Techniques:
Often, a PET scan is performed concurrently with a CT scan to merge functional and anatomical information. This hybrid approach enhances diagnostic accuracy, particularly in oncology. It helps to pinpoint the location of a tumor and determine its metabolic activity. - Radiation Dose:
Both modalities expose patients to ionizing radiation, but the doses vary. Typically, a CT scan results in higher radiation exposure compared to PET. This consideration is pivotal, especially for repeated imaging or young patients. - Time Efficiency:
A CT scan generally takes less time than a PET scan, which can be beneficial in emergency cases where rapid diagnosis is essential.
The decision on using PET vs. CT or the combination thereof should take into account the patient's unique clinical scenario and what information is necessary for diagnosis or treatment management.
Synergy of PET and MRI
Magnetic Resonance Imaging (MRI) and PET scanning have recently been integrated more frequently, resulting in substantial improvements in diagnostic capabilities. MRI offers superior soft tissue contrast and detailed images of anatomy, while PET depicts metabolic processes. Their synergy creates a robust platform for multifaceted analysis:
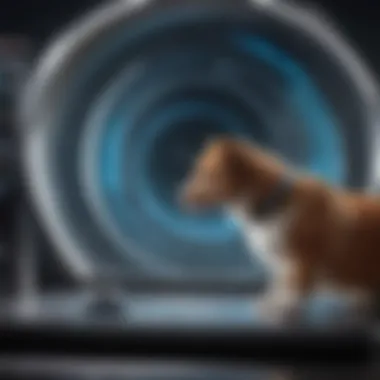
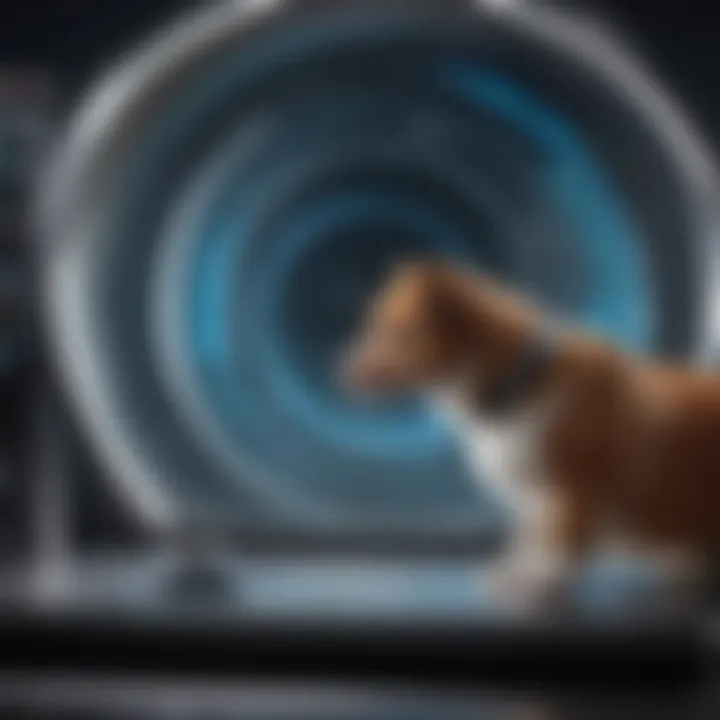
- Enhanced Imaging Quality:
When combined, MRI's high-resolution images complement PET's metabolic insights. This union aids in assessing complex conditions like brain tumors, whereby tumors can be identified anatomically through MRI and functionally through PET. - Lower Radiation Exposure:
Since MRI does not use ionizing radiation, pairing it with PET may lower patients’ overall radiation exposure while retaining the advantages of functional imaging. - Innovative Technologies:
Emerging technologies, including PET/MRI systems, exemplify advancements in imaging. These devices allow for simultaneous acquisition of images from both modalities, improving efficiency and patient throughput. - Clinical Applications:
The synergy of PET and MRI is particularly prominent in neurology, oncology, and cardiology. It enhances precision in diagnosing and managing neurodegenerative diseases and malignancies while offering comprehensive cardiotoxicity studies.
The collaborative use of PET and MRI signifies a shift towards personalized medicine, optimizing patient diagnostic journeys.
Advancements in PET Technology
Advancements in PET technology are critical for enhancing the efficacy and precision of medical imaging. As health care continues to evolve, the demand for advanced imaging modalities like positron emission tomography increases. These innovations are not confined to hardware upgrades; they encompass software enhancements and better integration with other imaging techniques. This section explores recent innovations in PET technology and examines future directions in PET research. Such insights could benefit countless professionals in the field by improving diagnostic capabilities and patient outcomes.
Recent Innovations
Several recent innovations have transformed the landscape of PET scanning. One noteworthy development is the integration of time-of-flight (TOF) technology. This method improves image resolution by utilizing the time it takes for detected photons to reach the detector following annihilation events. Utilizing TOF enables clinicians to obtain higher-quality images, allowing for better differentiation of tissues or lesions.
Another significant advancement involves the use of hybrid imaging systems, which combine PET with other modalities like computed tomography or magnetic resonance imaging. Systems such as the PET/CT have become increasingly standard. They provide complementary information, allowing for more comprehensive assessments. These integrated systems can help in accurately staging cancers and assessing treatment response.
Furthermore, advances in radiotracer development have expanded the applications of PET scanning in various fields. Newer tracers can target specific biological processes, leading to improved diagnosis and monitoring of diseases such as Alzheimer’s or cardiovascular conditions. The progression towards specific radiotracers means that PET can perform more complex assessments than ever before, contributing to precision medicine.
Future Directions in PET Research
The future of PET research promises continued growth in various areas. One emerging focus is on the development of smaller, portable PET scanners. These devices could allow for bedside imaging, making PET accessible in emergency settings or rural hospitals lacking advanced imaging tools. The portability concept could significantly impact how and where imaging is performed, increasing patient comfort and convenience.
Another promising direction involves the exploration of artificial intelligence algorithms. These AI techniques can enhance image reconstruction and data interpretation, possibly reducing the time between image acquisition and diagnostic reporting. The synergy between AI and PET could transform efficiency while maintaining high accuracy levels.
Moreover, the potential for personalized medicine is increasing. Research is looking into tailoring PET imaging to individual patients based on their unique biochemistry. This customization may lead to better treatment plans and outcomes, especially in oncology.
"The incorporation of AI in PET technology marks a significant milestone in the quest for precise medical diagnostics."
Considerations and Limitations of PET Scanning
In like any medical imaging technique, positron emission tomography (PET) scanning has its benefits and concerns. Understanding these considerations is vital for both practitioners and patients. This section will address two significant aspects: radiation exposure concerns and technical limitations. These discussions will enhance comprehension about the responsible use of PET scanners in medical diagnostics and potential implications for future developments in the field.
Radiation Exposure Concerns
PET scanning involves the injection of a radiopharmaceutical agent, which emits positrons during decay. This process allows for imaging metabolic processes within the body. However, these radiopharmaceuticals also expose the patient to ionizing radiation. Although the levels of radiation are generally low and comparable to that of a single CT scan, it is crucial to mitigate and monitor exposure, especially in vulnerable populations such as pregnant women and young children.
Some points to consider include:
- Cumulative Exposure: For patients requiring multiple scans, the total radiation dose can become significant over time.
- Risk Assessment: Evaluating the risk versus benefit is essential. While PET scans provide critical diagnostic information, the relatively small risk of radiation exposure should be acknowledged.
To minimize radiation dose, clinicians may explore alternative imaging modalities when appropriate. In addition, advancements in technology aim to reduce exposure levels without sacrificing image quality. Continuous research is necessary to create more efficient and safer radiopharmaceuticals, providing clearer imaging results while ensuring patient safety.
"Being informed about radiation risks empowers patients and healthcare providers in their decision-making process regarding PET scanning".
Technical Limitations
Despite the significant advancements in PET technology, the method is not without its limitations. Understanding these concerns allows for better application and prompts continual improvement.
Key technical limitations include:
- Spatial Resolution: The resolution of PET scans is typically lower than that of some other imaging techniques, such as MRI or CT. This limitation can lead to challenges in visualizing small lesions or subtle differences in tissue types.
- Cost Factor: The expense of PET scanners and associated radiopharmaceuticals can be a barrier for some healthcare institutions. This may limit patient access, particularly in resource-constrained settings.
- Limited Availability: Not all medical facilities possess the infrastructure needed for PET scanning, leading to delays in diagnostics for patients.
Moreover, the interpretation of PET scans may require specialized training. The complexity of the data processing techniques must be understood thoroughly to avoid misdiagnosis or missed abnormalities. Practitioners need to stay updated on the latest research and technology to address these limitations effectively.
Ending
The conclusion of this article emphasizes the significance of comprehending the mechanisms behind PET scanners. As one of the leading technologies in medical imaging, PET has advanced diagnostics, allowing for better disease detection and management. The insights shared here serve to highlight several crucial elements regarding its operations, applications, and implications for the future of medical imaging.
Summary of Key Points
In this article, we outlined several key points that are essential for a nuanced understanding of PET scanning:
- Operational Principles: Understanding positron emission, radioisotope production, and how images are acquired is essential.
- Technological Framework: The role of detector systems and imaging techniques provides foundational knowledge for comprehending how PET operates.
- Applications: PET has significant utility in oncology, neurology, and cardiology, showcasing its versatility in medical diagnostics.
- Comparative Analysis: The synergistic effects of PET with other imaging modalities like CT and MRI demonstrate its collaborative potential.
- Advancements: Staying aware of the latest innovations and future directions will be vital for continued improvement in PET technology.
- Considerations: Finally, recognizing limitations such as radiation exposure and technical challenges is crucial for responsible usage and development.
The Role of PET in Future Medical Imaging
The future of PET in medical imaging appears promising. Continuous advancements in technology and technique are pushing the boundaries of what is possible. Innovations in radiotracer development and scanner design may lead to enhanced image quality, reduced scan times, and lower radiation doses. Such improvements will further optimize its application in precision medicine where tailored diagnosis and treatment are essential.
Moreover, integration of artificial intelligence in image analysis may allow for predictive analytics, offering new insights into disease progression and patient outcomes. The collaborative use of PET with other imaging techniques will also likely broaden its diagnostic capabilities.