Exploring the Scientific Tube: Principles and Innovations
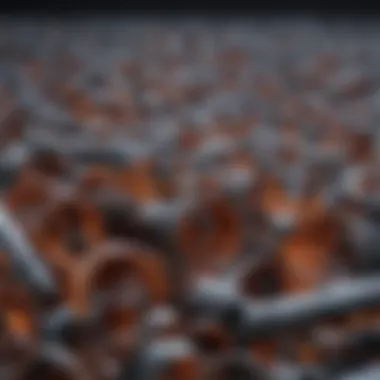
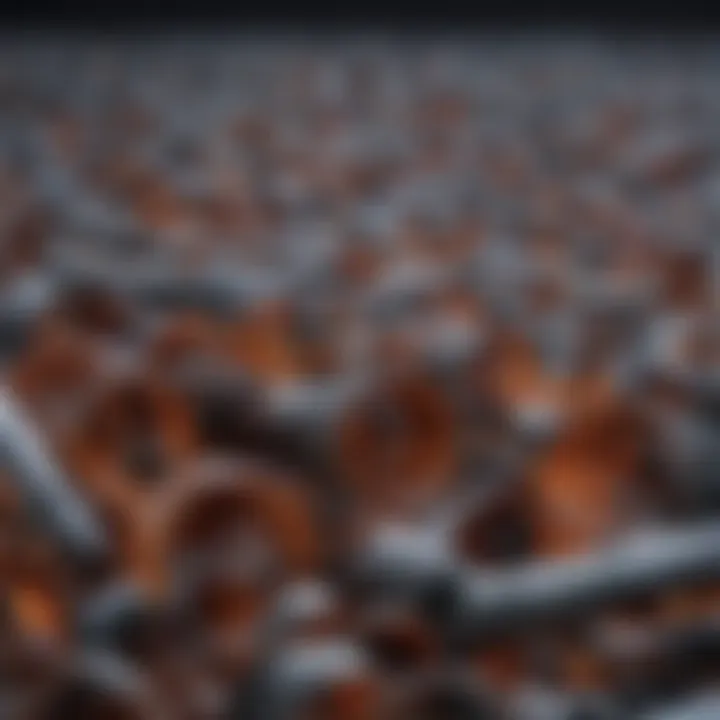
Intro
Scientific tubes have become integral components in a range of disciplines, facilitating numerous experiments and processes. Their design and material properties dictate performance and applicability. This article reviews these tubes, focusing on their principles, applications, and current innovations.
Understanding the functionality of scientific tubes is crucial for students, researchers, and professionals. The discussion encompasses fundamental concepts that govern their operation and effectiveness in various settings. By exploring these elements, we will uncover how these seemingly simple objects play a pivotal role in advancing scientific inquiry.
Research Overview
Summary of Key Findings
The exploration of scientific tubes reveals several key findings:
- Material properties: The choice of materials such as glass, plastic, and metals greatly impacts durability and chemical compatibility.
- Design considerations: Dimensions and shapes are tailored to suit specific applications, enhancing fluid dynamics and ease of use.
- Innovative applications: From laboratory settings to industrial processes, scientific tubes are utilized in diverse fields, including biology, chemistry, and engineering.
Relevance to Current Scientific Discussions
Recent advancements in materials science have significantly influenced the development of scientific tubes. Discussion around these innovations is relevant to ongoing dialogues about efficiency and sustainability in research. The integration of new materials and design methodologies aligns with broader trends in minimizing waste and maximizing functionality in scientific practices. This is especially pertinent in the context of growing environmental awareness and regulatory standards.
Methodology
Research Design and Approach
A systematic review was conducted to synthesize existing literature on scientific tubes. This involved examining peer-reviewed articles, industry reports, and case studies to gather a comprehensive understanding of the topic.
Data Collection and Analysis Techniques
Data collection involved gathering quantitative and qualitative data from multiple sources. Analytical techniques included:
- Comparative analysis of material properties
- Evaluation of design specifications across applications
- Case studies highlighting innovative uses in various industries
These methods provide a robust foundation for understanding the multifaceted nature of scientific tubes and contribute to a detailed analysis of recent trends and future directions in tube development.
"Scientific tubes are not mere vessels; they are the backbone of modern experimental science, allowing precise manipulation and analysis of substances in diverse environments."
By diving deep into the principles and applications of these tubes, we aim to provide valuable insights that can inform the work of researchers and practitioners alike.
Preface to Scientific Tubes
Scientific tubes are fundamental tools in various scientific fields. They serve multiple purposes, from transporting gases and liquids to facilitating experiments in laboratory settings. Understanding the principles governing these tubes is essential. This knowledge is relevant for students, researchers, and industry professionals seeking to enhance their work efficiency and experimental outcomes.
Scientific tubes come in different materials, shapes, and sizes, each tailored for specific applications. The importance of selecting the correct type of tube cannot be overstated. It directly impacts the performance of experiments and the safety of procedures. Thus, comprehending the relationship between material properties and design is vital for optimal use.
This section examines how scientific tubes contribute to research and industry. Tubes are not just passive conduits; they play active roles in the manipulation and analysis of substances. Through various applications, they enable groundbreaking discoveries and innovations.
The choice of scientific tube can significantly influence both results and safety in experiments.
Definition and Overview
Scientific tubes can be defined as cylindrical containers used in scientific applications. They are typically hollowed-out structures designed to hold, mix, or transport fluids or gases. Tubes are made from various materials, including glass, plastic, and metal, each selected based on the specific requirements of the task at hand.
In laboratories, for example, glass test tubes are commonly used for chemical reactions. They offer excellent transparency, allowing for observation of reactions without interference. In contrast, plastic tubes may be employed in applications where breakage is a concern, such as fieldwork.
Tubes also vary in dimensions, influencing their suitability for different applications. Selecting the appropriate size and type is fundamental for achieving desired outcomes in experiments. This understanding is crucial for anyone involved in scientific exploration, ensuring they can choose the most effective tools at their disposal.
Historical Context of Tubular Research
The historical examination of tubular research holds significant value in understanding the current advancements in the field of scientific tubes. It is a journey that not only charts the evolution of designs and materials but also underscores the impact of these innovations on various scientific disciplines. Recognizing early milestones provides insights into how the initial discoveries have paved the way for modern applications. Understanding this context illuminates the ongoing progression of tube technology, showcasing its relevance in both laboratory settings and industrial applications.
Early Discoveries
The initial exploration of scientific tubes can be traced back to fundamental scientific inquiries of the past. In the early days, tubes were primarily made of materials such as wood and primitive glass. Scientists utilized these rudimentary designs to gather essential data in fluid mechanics and gas behaviors. Notably, the development of the Barometer in the 17th century by Evangelista Torricelli, which employed a column of mercury within a glass tube, marked a pivotal point. This not only demonstrated the principles of atmospheric pressure but also showcased how tube design could effectively aid in scientific discovery.
These early designs laid a foundation for further studies in chemistry and physics. They highlighted the necessity for reliable containment of substances under varying pressures. As a result, the application of tubes in laboratory practices gradually increased, where they served for both investigation and experimentation. The significance of these discoveries didn't merely rest in their immediate scientific contributions. They demonstrated the transformative potential of tubes in advancing knowledge across various fields.
Evolution of Materials
Over time, the materials used in constructing scientific tubes experienced significant advancements. The transition from simple glass to more sophisticated composites and metals expanded the functionality and application of these tubes. Initially, glass remained the workhorse due to its ease of production and transparency, which allowed for the observation of contents within. However, limitations in strength and thermal resistance prompted researchers to seek alternatives.
The introduction of plastics revolutionized tube manufacturing during the 20th century. Materials such as polyvinyl chloride (PVC) and polytetrafluoroethylene (PTFE) offered outstanding chemical resistance and lightweight properties. This transition not only facilitated handling but also enabled tubes to be used in a wider variety of experiments and industrial contexts.
Metals like stainless steel have also become prominent in tube applications, particularly due to their mechanical strength and durability. They are indispensable in medical applications, including transportation of fluids in surgical settings. The continuous evolution of materials reflects the dynamic nature of scientific research, constantly adapting to meet the requirements of new technologies and methodologies.
The historical context thus emphasizes the significance of advances in material science in enhancing the functionality and safety of scientific tubes. Recognizing these historical milestones equips current researchers and professionals with a better understanding of the principles that govern tube design and selection in modern applications.
Types of Scientific Tubes
Understanding the different types of scientific tubes is crucial in various fields, including chemistry, biology, and engineering. Each type of tube serves specific functions, and the selection often depends on the application's requirements. Knowledge about these tubes allows researchers and professionals to choose the right equipment for experiments, production, and innovative applications. The consideration of material properties, design, and functionality directly impacts efficiency in both laboratory and industrial settings.
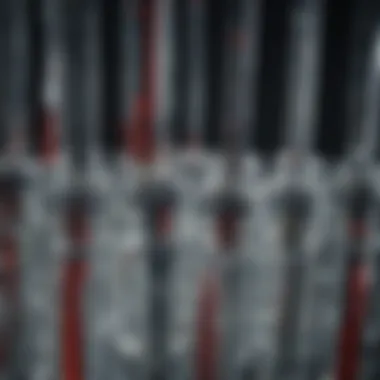
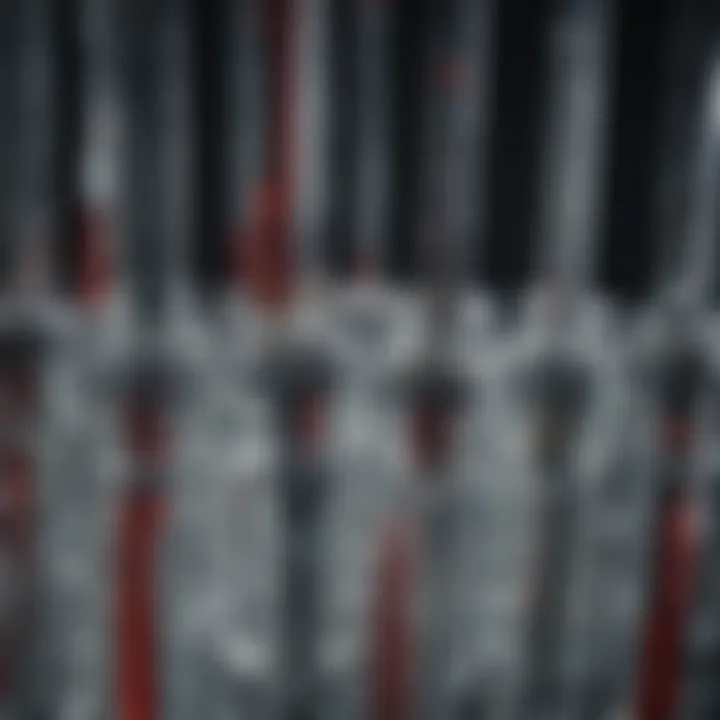
Glass Tubes
Glass tubes are widely recognized for their durability and resistance to a variety of chemicals. Their transparency makes it easy to observe reactions and processes. Borosilicate glass, specifically, is common in laboratory settings due to its low thermal expansion. This property reduces the risk of breakage when subjected to sudden temperature changes.
Applications of glass tubes include:
- Test tubes: Essential in any laboratory, these are used for mixing and heating liquids.
- Pipettes: Used for transferring precise volumes of liquids.
- Reaction vessels: Ideal for conducting chemical reactions under controlled conditions.
However, one must handle glass tubes with care, as they can be fragile. Despite this, their overall performance and utility in scientific work are unrivaled, making them a staple in laboratories worldwide.
Plastic Tubes
Plastic tubes offer a more lightweight and flexible alternative to their glass counterparts. Made from various polymers like polyethylene or polypropylene, these tubes are resistant to impact and breakage. This attribute makes plastic tubes suitable for environments where safety is a concern. They are also available in various sizes and shapes, catering to different laboratory needs.
Key features of plastic tubes include:
- Chemical resistance: Many plastics withstand a broad range of chemicals, although not as much as glass.
- Cost-effectiveness: Generally cheaper to produce and replace when damaged.
- Versatility: Used in both laboratory and industrial applications, such as sample collection and storage.
Despite their advantages, plastic tubes have limitations in strength and thermal resistance compared to glass. It's essential to choose the right type of plastic according to the intended application to prevent issues like leaching or deformation under heat.
Metal Tubes
Metal tubes, typically made from stainless steel or aluminum, are known for their outstanding strength and durability. They are tailored for high-pressure environments and extreme temperatures, making them suitable for industrial applications. Because of their robustness, metal tubes are often utilized in areas where glass or plastic would not endure.
Common uses for metal tubes include:
- Piping systems: Used in various industries, including oil and gas, to transport fluids.
- Structural components: Important in building frameworks, providing strength and resilience.
- Chemical reactors: Essential for handling corrosive materials in a high-pressure context.
However, they are generally heavier and more expensive than glass or plastic tubes. The choice of metal can significantly affect performance, particularly in corrosive conditions. Therefore, it is crucial to consider the specific properties of the metal when selecting tubes for particular applications.
"The choice of material in scientific tubes significantly influences the success of experiments and applications. It is vital to evaluate their properties carefully."
In summary, the classification of scientific tubes into glass, plastic, and metal categories is important for their proper application. Each type has unique properties that cater to specific needs in various scientific fields.
Material Properties and Selection
The significance of material properties and their selection cannot be overstated when discussing scientific tubes. The right material can determine not only the efficacy of the tube in its intended application but also its longevity and reliability. A thorough understanding of how various materials behave under different conditions is essential for researchers, educators, and professionals. In addition, selecting the right material can enhance performance while minimizing costs and environmental impact.
Mechanical Strength
Mechanical strength defines the ability of a tube to withstand external forces without failing. This property is particularly crucial in applications such as gas and fluid transport. A tube with insufficient mechanical strength could deform or break, leading to costly failures or risks to safety.
Some key points regarding mechanical strength include:
- Tensile Strength: The ability of the tube to resist tension forces.
- Flexural Strength: Resistance to bending forces, especially significant in longer tubes subjected to weight.
- Impact Resistance: The capacity to absorb energy during sudden impacts is vital for tubes used in dynamic environments.
When selecting materials, one must consider the specific mechanical requirements of the application at hand.
Chemical Resistance
The interaction between the tube material and the substances it will contain is a critical factor. Chemical resistance is essential for ensuring that the tube will not corrode, degrade, or leach harmful substances into its contents.
Common factors affecting chemical resistance include:
- Material Composition: Different materials react differently with various chemicals. For instance, glass tubes are typically more resistant to acidic solutions than plastic ones.
- Concentration of Chemicals: The strength of the chemical solution can also influence the material’s resistance.
- Temperature Conditions: Higher temperatures can accelerate the degradation process for some materials.
Choosing chemically resistant materials can lead to safer and more effective research outcomes.
Thermal Stability
Thermal stability refers to how well a tube can maintain its properties when exposed to varying temperature conditions. This is vital in applications where tubes may be subjected to extremes of heat or cold, such as in certain laboratory settings.
Consider these aspects of thermal stability:
- Melting Point: Materials with high melting points, like certain metals, are suitable for high-temperature applications.
- Thermal Expansion: Different materials expand at different rates; a mismatch in thermal expansion between connections can lead to failure.
- Heat Resistance: The ability to resist physical and chemical changes at elevated temperatures is critical for maintaining tube integrity.
It is essential to assess all these factors to ensure that the selected material will stand the test of working conditions.
"The careful selection of materials based on their mechanical strength, chemical resistance, and thermal stability is fundamental for the successful implementation of scientific tubes in any application."
In summary, understanding the properties of materials is key to selecting the right tubes for specific applications. Proper selection enhances durability, safety and contributes to the overall success of scientific endeavors.
Design Considerations
Design considerations in the context of scientific tubes significantly influence their functionality and effectiveness. These principles are not just mere preferences; they are essential to ensuring that the tubes perform optimally in various applications. When selecting or designing a tube, factors such as dimensions, tolerances, and surface smoothness must be meticulously evaluated.
Dimensions and Tolerances
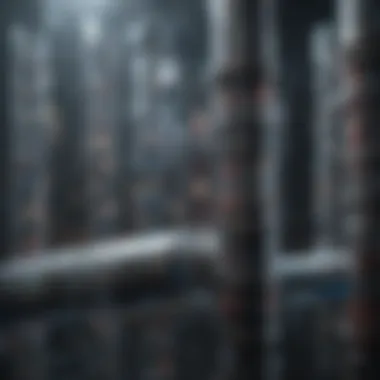
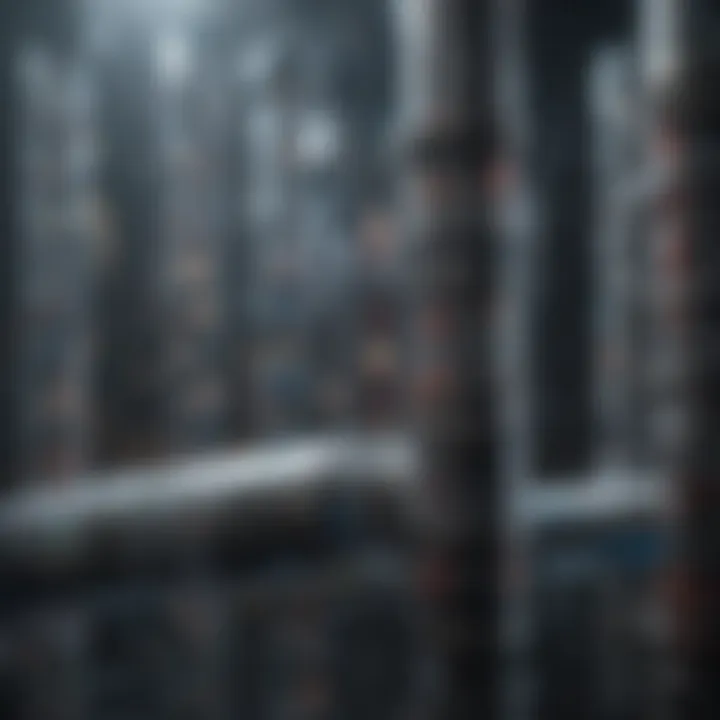
The dimensions of a scientific tube are critical. It is vital to determine the appropriate diameter, length, and wall thickness based on the intended application. For instance, in laboratory environments, glass tubes used for chromatography must have precise dimensions to allow proper fluid movement and separation. Similarly, in medical applications, tubes must fit accurately to ensure safe and effective use.
Tolerances refer to the allowable variations in dimensions. High precision is often required in research and industrial settings. For example, deviations beyond specified tolerances can lead to equipment failure, inaccuracies in measurements, or even safety hazards. Therefore, precise machining and quality control processes are crucial in the tube fabrication process.
Surface Smoothness
The surface smoothness of a scientific tube also plays a significant role in its operational capabilities. A smoother inner surface minimizes resistance to fluid flow, which is essential for maintaining accurate flow rates during experiments. Additionally, surface smoothness can impact cleaning efficiency and contamination risk in laboratory settings.
In industrial applications, such as those involving corrosive chemicals, smooth surfaces reduce the risk of buildup that might compromise the material's integrity over time. Optimal surface finish can prolong the lifespan of the tube and enhance its overall effectiveness in various environments.
"In scientific applications, neglecting the finer details of design can lead to substantial consequences in outcomes and results."
Core Applications in Science
Scientific tubes play a crucial role in various domains of research and industry. Their design and materials have been fine-tuned to meet specific needs, making them essential tools for experimentation and production. In this section, we will explore the core applications of scientific tubes, focusing on their significance, benefits, and vital considerations in laboratory, medical, and industrial contexts.
Laboratory Use
In laboratories, scientific tubes are integral for conducting experiments and tests. They serve multiple purposes, such as mixing, heating, and storing samples. For instance, test tubes allow for easy observation of reactions, while graduated cylinders enable precise measurement of liquids.
Some key benefits include:
- Versatility: Tubes come in various shapes and sizes, accommodating diverse experimental requirements.
- Reactivity: Depending on the material, different tubes can withstand various chemical reactions, thus ensuring integrity of samples.
- Accessibility: Many types of tubes are designed for single-use, which helps minimize contamination risks.
The choice of tube can impact experimental outcomes significantly; hence, researchers must consider factors such as material compatibility and thermal properties during selection.
Medical Applications
Within the medical field, scientific tubes hold substantial importance. Blood collection tubes, for example, are designed specifically to store and preserve specimen integrity for analysis. Different additives are included in some tubes to aid in coagulation or to stabilize certain components for accurate results.
Key considerations include:
- Sterilization: Tubes must be sterilized appropriately to avoid infection.
- Material Safety: The materials used must not interact negatively with biological samples.
- Regulatory Compliance: Medical tubes need to adhere to strict regulatory standards for safety.
The innovation of materials, such as those used in nano-tube applications, further expands their potential, leading to improved diagnostic methods.
Industrial Applications
In the industrial sector, scientific tubes contribute significantly to manufacturing processes and product development. For example, they are commonly used in systems for conveying fluids, ensuring optimal flow rates and durability.
Important aspects include:
- Durability: Metal and high-grade plastic tubes are often chosen for their strength and resistance to wear, especially in high-pressure environments.
- Customization: Industries often require tubes that are tailored to specific dimensions and requirements, making adaptability a vital factor.
- Cost Efficiency: Selecting the right tube can lead to lower operational costs over time, by reducing maintenance and replacement needs.
In summary, scientific tubes are indispensable resources across various sectors. Their applications in laboratories, medical facilities, and industries not only highlight their versatility but also underline the importance of material selection and design considerations. > This complexity is essential for ensuring optimal results in a wide range of applications.
Understanding these core applications allows professionals in research and industry to effectively utilize tubes, leading to enhanced outcomes in their respective fields.
Innovations in Tube Technology
Innovations in tube technology have made significant impacts in several fields, including material science, engineering, and biology. These advancements not only enhance the functionality and versatility of scientific tubes but also open new possibilities for their applications. Understanding these innovations is essential for researchers and industry professionals who aim to leverage tubes' potential in various projects.
One notable trend is the development of advanced materials that improve several properties of the tubes, such as mechanical strength and chemical resistance. These materials allow for more complex experiments and applications, leading to more reliable results in laboratory settings. Additionally, innovations in manufacturing techniques, such as 3D printing, are streamlining production and reducing costs, making scientific tubes more accessible.
The significance of innovations also lies in their capacity to integrate with other technologies, such as sensors and data collection devices. This development can lead to the creation of tubes that actively monitor conditions within the tube itself, thus providing real-time data.
In short, innovations in tube technology expand the boundaries of what is possible, enabling groundbreaking work across science and industry.
Nanotubes and Their Applications
Nanotubes represent a fascinating area in tube technology, known for their unique properties. These cylindrical structures, composed of carbon atoms, are roughly one nanometer in diameter. Their exceptional strength and lightweight characteristics make them suitable for various applications.
Nanotubes are gaining traction in areas such as electronics, where they can be used to create smaller and more efficient components. In the medical field, they are being explored for drug delivery systems, providing targeted therapies directly to cells. Furthermore, their unique thermal and electrical properties make them valuable in aerospace and energy applications, including improved battery technologies and lightweight structural materials.
Several key benefits arise from the use of nanotubes:
- High Strength-to-Weight Ratio: Offers increased performance and reduced material use.
- Electrical Conductivity: Essential for electronic applications, which can lead to advancements in circuit design.
- Thermal Conductivity: Allows for heat dissipation in sensitive applications, such as electronics.
While promising, the implementation of nanotubes does come with challenges such as production costs and potential environmental concerns if not managed properly. Achieving a balance between innovation and safety is crucial in furthering nanotube applications.
Smart Tubes
Smart tubes signify a remarkable advancement in tube technology, combining traditional functions with innovative features. These tubes are equipped with sensors and electronic components that allow for enhanced monitoring and control of their environment. This capability is particularly beneficial in scientific research and industrial applications, where conditions need to be closely observed.
Smart tubes are capable of detecting various parameters such as pressure, temperature, and chemical composition. This enables researchers to monitor experiments in real-time, increasing efficiency and precision.
For example, in medical applications, smart tubes can be integrated into devices to monitor patient health metrics continuously. In the industrial sector, these tubes can help ensure that processes operate within desired parameters, preventing catastrophic failures that might arise from unnoticed fluctuations.
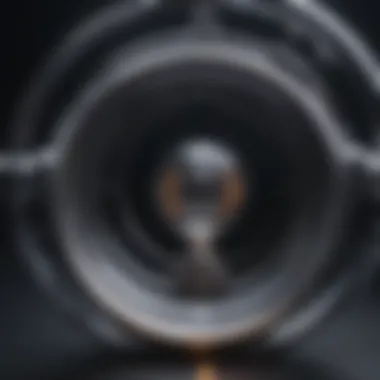
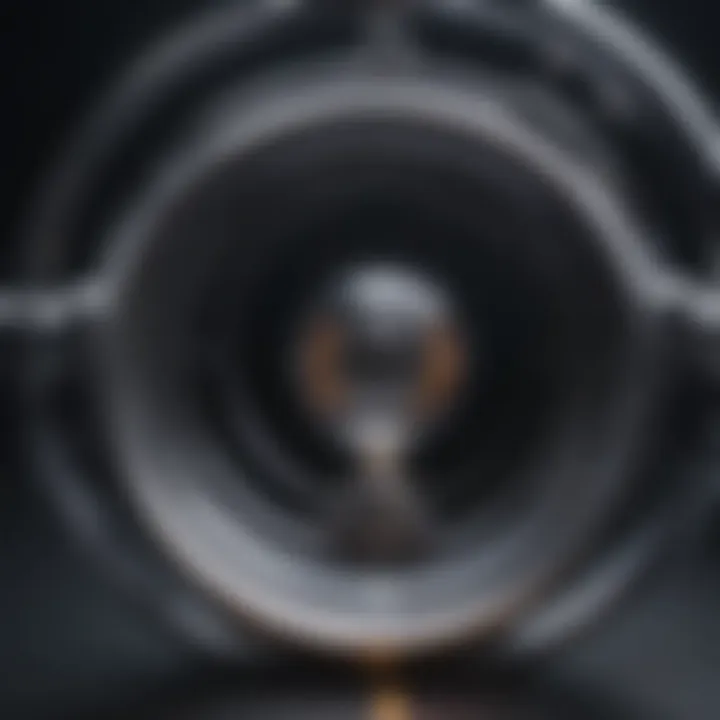
The innovations brought by smart tubes can be highlighted in several areas:
- Real-Time Monitoring: Enhances the accuracy of data collection, which is vital for research.
- Automation: Reduces human error and allows for seamless operation of systems.
- Versatility: Their application spans multiple fields such as pharmaceuticals, construction, and environmental science.
"The integration of smart technology into tube systems marks a significant step toward more efficient and reliable research and industrial processes."
Regulatory Standards and Safety
Regulatory standards and safety considerations are paramount in the realm of scientific tubes. The use of these tubes spans various fields, including laboratories, medical facilities, and industrial applications. Therefore, adherence to established safety guidelines is crucial to ensure that the materials used in scientific tubes are not only effective but also safe for users and the environment. The implications of regulations extend to health, performance, and legal compliance, making this a vital topic for all stakeholders involved.
Material Safety Guidelines
Material safety guidelines are designed to ensure that the materials employed in the construction of scientific tubes do not pose risks to health and safety. Various organizations provide these guidelines, which dictate the testing and certification procedures for materials like glass, plastic, and metal. Here are some key points regarding material safety:
- Testing Requirements: Materials must undergo rigorous testing for toxicity, chemical resistance, and structural integrity. This is essential to ascertain that no harmful substances leach into samples.
- Standards Compliance: Materials should comply with recognized standards set by agencies such as the American National Standards Institute (ANSI) or the International Organization for Standardization (ISO).
- Supplier Verification: Users need to verify that suppliers provide certified materials that adhere to safety guidelines.
Not only is this beneficial for research integrity, but it also diminishes the risk of hazardous exposure for users.
Environmental Impact
The environmental impact of scientific tubes is another consideration that comes under regulatory scrutiny. Improper disposal and production of these tubes can lead to detrimental effects on the ecosystem. Addressing these concerns involves:
- Sustainable Materials: Promoting the use of biodegradable or recycled materials can greatly lessen environmental footprints. Such innovations align with green chemistry principles and decrease reliance on non-renewable resources.
- End-of-Life Disposal Protocols: Clear guidelines need to be established regarding the disposal or recycling of used tubes. Many materials can be recycled, while others may require special handling to mitigate environmental risks.
- Life Cycle Analysis: Conducting life cycle analyses of scientific tubes can help in assessing their overall environmental impact, from production to disposal.
"Adhering to material safety guidelines and understanding environmental impact helps secure the health of users and the planet."
Challenges and Limitations
In the pursuit of excellence in scientific methodologies, the challenges and limitations associated with scientific tubes are critical for researchers, educators, and industry professionals to understand. These factors can significantly impact the performance and applicability of tubes in various contexts. Understanding these challenges allows for informed decisions and can lead to innovations that improve utility and efficiency.
Cost of Production
The cost of production for scientific tubes varies based on several factors, including materials, manufacturing processes, and scale of production. Each type of tube—glass, plastic, and metal—carries its own unique cost implications.
- Material Choices: High-quality materials often equate to higher costs. For instance, borosilicate glass is favored for its thermal resistance, but it is more expensive compared to regular soda-lime glass.
- Manufacturing Process: Different methods, such as blow molding for plastics, can influence costs. Speciality techniques, like creating seamless metal tubes, add to expenses due to precision requirements.
- Economies of Scale: Larger production runs can reduce individual unit costs. However, the initial investment in machinery and setup can be a barrier for smaller companies.
Balancing these cost factors is crucial. A low-cost tube might not meet performance standards, resulting in higher long-term costs due to replacements or failures.
Durability Issues
Durability is a vital consideration when choosing scientific tubes. The ability of a tube to withstand various environmental factors can affect its longevity and operational efficiency.
- Material Weakness: Different materials have different weaknesses. For example, glass can be fragile, making it unsuitable for high-impact environments. On the other hand, plastic can degrade under UV exposure or extreme temperatures.
- Stress Resistance: Tubes used in high-pressure or high-temperature applications must be able to handle these stresses without failure. The wrong choice of material or design can lead to catastrophic failures, endangering both data integrity and safety.
- Maintenance Requirements: Durable tubes might require less frequent replacements but could need careful cleaning or maintenance. This adds another layer of cost and logistical considerations.
"Choosing the right tube is not just a matter of performance but also of ensuring long-term reliability and safety in experiments."
Future of Scientific Tubes
The future of scientific tubes is pivotal as they play a significant role in both research and industrial applications. As science and technology advance, these tubes are becoming essential components in various innovative processes and products. With an increasing emphasis on precision and efficiency, developments in tube design, materials, and technology ensure that they remain relevant in a rapidly evolving landscape.
Emerging Research Fields
Emerging research fields are continually reshaping the landscape of scientific tubes. One notable field is nanotechnology. Here, researchers investigate the properties of nanoscale materials, leading to the creation of specialized tubes, such as carbon nanotubes. These tubes offer exceptional strength and conductivity, paving the way for applications in electronics, materials science, and even medicine.
Another significant area is biomedical research. Scientists are exploring tubes that can target specific cells for drug delivery or that can serve as scaffolding for tissue engineering. For example, biodegradable tubes are being developed for controlled release of medications, which could revolutionize treatment options in healthcare.
Moreover, environmental science is also becoming increasingly relevant. Researchers are focusing on tubes that incorporate sustainable materials and processes. This includes bioplastics and recycled materials, aimed at reducing the ecological footprint associated with traditional tube production. These developments show how scientific tubes can impact future sustainability efforts.
Technological Advancements
Technological advancements are integral to the future of scientific tubes. One significant advancement is the use of 3D printing techniques. This has made it possible to create custom-sized tubes tailored to specific experimental needs. Researchers can quickly prototype and produce specialized tubes, which saves time and resources.
Another interesting advancement is the integration of smart technology into tube designs. Smart tubes equipped with sensors can monitor conditions within the tube, such as temperature and pressure. This is incredibly valuable in both laboratory settings and industrial applications, as it allows for better control and more accurate data collection.
Additionally, advancements in materials science are leading to the development of stronger, lighter materials that can withstand extreme conditions. This is particularly vital for tubes that will be used in aerospace and automotive applications, where performance and durability are crucial.
"Innovations in scientific tube technology are aimed at meeting the demands of various fields while minimizing environmental impact."
The convergence of these emerging fields and technological advancements highlights the dynamic nature of the science behind tubes. As the demands for customized and high-performance solutions increase, the future of scientific tubes appears promising.
The End
The conclusion serves as a vital component of any scholarly article, encapsulating the essence of the discussion around scientific tubes within this work. Revisiting key themes allows for a clear understanding of the myriad ways in which scientific tubes contribute to both research and industry. This reflection on the information presented ensures that readers—be it students, researchers, educators, or professionals—are left with a consolidated grasp of the material properties, design considerations, core applications, and innovations in tube technology.
Summary of Key Points
In summary, the exploration of scientific tubes encompasses several pivotal aspects:
- Types and Materials: Distinct categories such as glass, plastic, and metal tubes have unique properties and applications.
- Material Properties: Understanding mechanical strength, chemical resistance, and thermal stability is crucial in selecting appropriate tubes for specific uses.
- Design Considerations: Dimensions, tolerances, and surface smoothness are fundamental in ensuring functionality and reliability.
- Applications: From laboratories to industrial settings, the versatility of tubes demonstrates their integral role in various fields.
- Innovations: Advances like nanotubes and smart tubes point toward a future where the functionality of scientific tubes continues to evolve.
- Safety and Regulations: Awareness of material safety guidelines and environmental impacts is necessary for sustainable practices.
- Challenges: Recognizing cost and durability issues can inform future improvements in tube production and design.
Final Thoughts on Scientific Tubes
As this article illustrates, scientific tubes are not merely physical objects; they are essential tools that drive scientific inquiry and industrial processes forward. With ongoing research and technological advancements, the future of scientific tubes holds great promise. The development of new materials and designs will likely not only enhance existing applications but also open up new avenues in scientific exploration.
Understanding the principles and innovations surrounding these tubes is crucial for anyone engaged in research or related fields. It emphasizes the importance of these components in achieving accurate, reliable results in both experimental and practical contexts. A comprehensive knowledge of scientific tubes equips professionals with tools necessary for progression in their respective fields, reinforcing the need to stay informed about new developments and applications.