Exploring the Revolutionary CRISPR Gene Editing
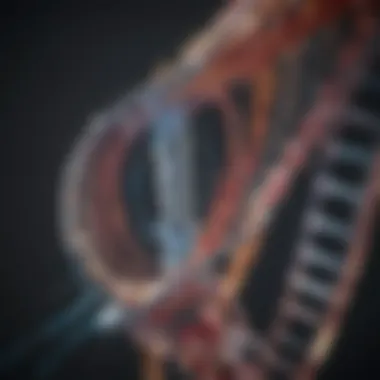
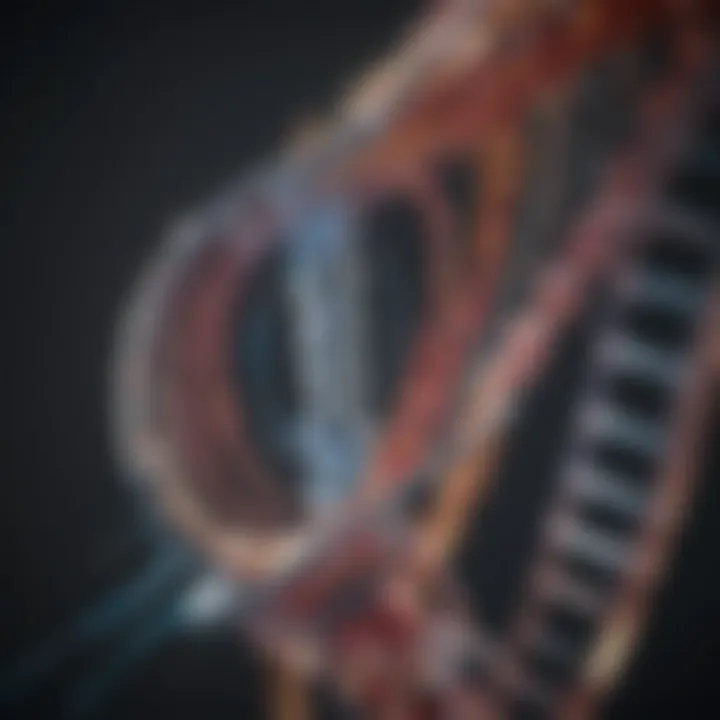
Intro
Gene editing has come a long way since its inception, with CRISPR standing out as a transformative technology in the field of genetics. The mechanisms behind CRISPR (Clustered Regularly Interspaced Short Palindromic Repeats) and its associated protein, Cas9, allow scientists to target specific areas within DNA with remarkable precision. This has opened up numerous possibilities in medicine, agriculture, and various scientific fields. Understanding CRISPR is vital, as its implications extend far beyond laboratory walls, potentially reshaping our approach to genetic diseases and biotechnology.
Research Overview
Summary of Key Findings
Research surrounding CRISPR technology indicates several key findings. The ability to edit genes with accuracy has demonstrated potential in areas including:
- Medical applications: Targeting genetic disorders such as sickle cell disease and cystic fibrosis.
- Agricultural improvements: Enhancing crop resistance to pests and environmental conditions.
- Basic research: Allowing scientists to better understand gene functions and interactions.
These findings showcase CRISPR’s versatility and efficiency, marking it as a pivotal tool in genetic research.
Relevance to Current Scientific Discussions
CRISPR has sparked intense discussions within the scientific community and beyond. It raises important questions about ethics, such as the implications of genetic modifications in humans. Current dialogues focus on:
- The potential for unintended consequences in gene editing.
- Regulatory frameworks needed to govern its use.
- The socio-economic impacts of widespread gene editing technology.
Overall, CRISPR is not just a topic of scientific interest but a subject that invites complex ethical considerations and societal dialogues.
Methodology
Research Design and Approach
The research conducted on CRISPR technology primarily involves both experimental and observational studies. These studies focus on the efficacy and safety of gene editing techniques in varied contexts. Researchers often employ:
- Lab experiments to explore specific genetic alterations.
- Field trials in agricultural settings to assess practical applications.
This diverse approach enriches the understanding of CRISPR’s potential and limitations.
Data Collection and Analysis Techniques
Data collection methods vary, including:
- Genomic sequencing to analyze edited genes.
- Bioinformatics tools to interpret large sets of genetic data.
- Surveys for gauging public perception on gene editing technologies.
Analysis often involves statistical methods to ensure the integrity of findings. The combination of these methods provides a comprehensive view of CRISPR’s capabilities and its impact on society.
Preamble to CRISPR
CRISPR stands as a pivotal advancement in the field of genetic engineering, embodying a sophisticated yet straightforward approach to gene editing. The introduction of CRISPR technology sheds light on the potential to not only alter genetic sequences with precision but also the profound implications it holds for various fields such as medicine, agriculture, and biotechnological research. As scientists and researchers continue to explore the depths of CRISPR, understanding its foundational aspects becomes essential for grasping its relevance in contemporary science.
Background of Gene Editing
Gene editing has a long and complex history, beginning with early techniques like restriction enzymes and developing into more sophisticated methods. Traditional forms of gene editing, such as homologous recombination, often faced challenges such as inefficiencies and off-target effects. These approaches laid the groundwork for the need for a more effective method. The advent of new technologies, like TALENs and zinc-finger nucleases, marked significant advancements but still had limitations in terms of ease of use and specificity.
The need for more efficient, accurate, and less intrusive methods of gene manipulation led scientists towards CRISPR. CRISPR, an acronym for Clustered Regularly Interspaced Short Palindromic Repeats, offers a groundbreaking method for gene editing that is not only efficient but also cost-effective. This technology provides the ability to target specific genes for modification, opening a myriad of possibilities in fields ranging from genetic research to clinical applications.
The Emergence of CRISPR Technology
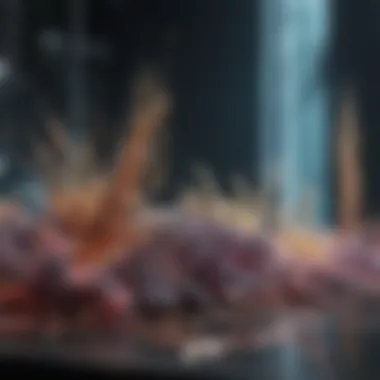
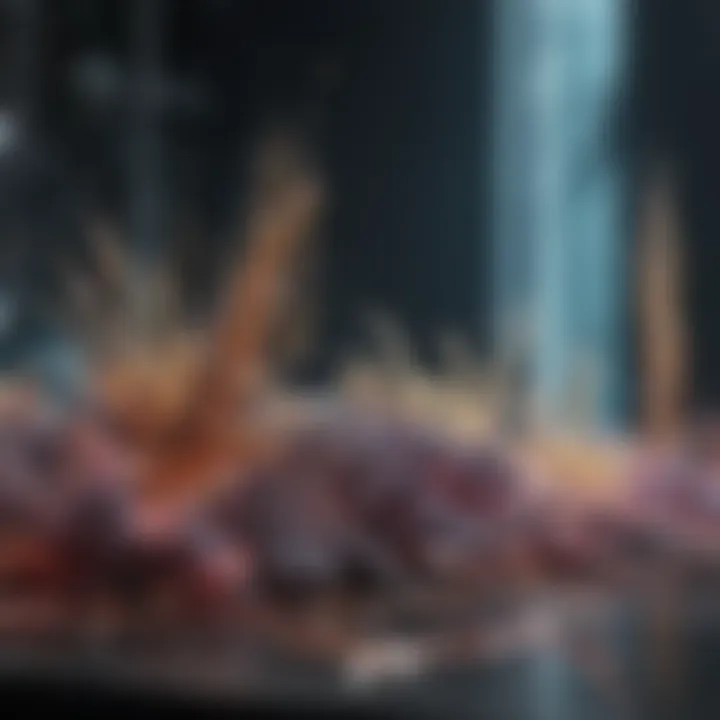
The discovery of CRISPR can be traced back to bacterial immune systems, where these sequences act as a defense mechanism against viruses. This natural process of adaptive immunity sparked interest among scientists who saw its potential for gene editing. The pivotal moment came when Jennifer Doudna and Emmanuelle Charpentier successfully repurposed this bacterial system for targeted gene editing in 2012. Their work laid the foundation for scientists to harness CRISPR-Cas9 as a tool to create precise modifications in DNA strands.
The emergence of CRISPR technology has since revolutionized the field of genetics with its remarkable capabilities. Within a short period, CRISPR has progressed from laboratory curiosity to a widely accepted tool used in both research and practical applications. Its versatility and adaptability contribute to its broad acceptance in various disciplines, ultimately reshaping how researchers address genetic disorders and environmental challenges.
"CRISPR technology marks the beginning of a new era in genetic research, providing tools that were previously unimaginable."
In summary, the introduction to CRISPR highlights essential aspects of gene editing. Its background reveals the evolution of genetic manipulation leading to the development of CRISPR. Furthermore, the emergence of this technology underscores its transformative impact, positioning CRISPR as a cornerstone of modern genetic research.
Understanding CRISPR Mechanism
The understanding of the CRISPR mechanism is paramount for comprehending how gene editing works at a molecular level. This section delves into the fundamental components that make CRISPR a powerful tool in genetic engineering. Studying this mechanism reveals insights into its precision, efficiency, and versatility. These elements not only elucidate how CRISPR operates but also invite discussions regarding its potential applications and limitations.
Components of CRISPR
The CRISPR system consists of various components that work in conjunction to facilitate targeted gene editing. Each component has a distinct role, contributing to the overall function and effectiveness of the editing process. Understanding these components enhances one’s grasp of how CRISPR achieves its desired outcomes.
CRISPR RNA
CRISPR RNA (crRNA) is essential for the targeting mechanism of the CRISPR system. It is synthesized from the CRISPR array, which contains sequences of foreign DNA from viral pathogens. The crRNA guides the Cas proteins to the specific DNA sequences in the target organism. One key characteristic is its ability to form a complex with Cas proteins, thus allowing for the precise binding to complementary DNA sequences.
Unlike other RNA forms, crRNA's unique feature lies in its ability to be specifically designed to match any DNA sequence of interest, making it a highly versatile choice for gene editing tasks. However, the reliance on accurate crRNA design is essential, as incorrect sequences can lead to unsuccessful edits or off-target effects.
Cas Proteins
Cas proteins, short for CRISPR-associated proteins, play a critical role in the CRISPR mechanism. The most widely studied protein is Cas9, which acts as a molecular scissor. It introduces double-strand breaks in the DNA at the targeted site dictated by the crRNA. The primary characteristic of Cas proteins is their programmable nature, enabling researchers to alter target sites easily.
The unique feature of Cas proteins, especially Cas9, is their dual functionality: they can cut DNA and facilitate repair through various cellular mechanisms. This ability is advantageous when implementing precise gene modifications. However, challenges arise regarding specificity, as these proteins can occasionally engage with non-target sites, leading to unintended genetic alterations.
DNA Targeting Mechanism
The DNA targeting mechanism is fundamental to CRISPR's success in gene editing. This component refers to the process through which the crRNA and Cas proteins identify and bind to the target DNA sequence. The key characteristic of this mechanism is its dependability on base-pair complementarity between the crRNA and the target DNA.
This distinctive feature allows for a high degree of accuracy in targeting, essential for reducing off-target effects during editing. However, one disadvantage of this mechanism is the requirement for specific protospacer adjacent motifs (PAMs) in the target DNA, restricting the locations where CRISPR can effectively operate.
How CRISPR Works: A Step-by-Step Process
The CRISPR gene editing process can be broken down into several steps, providing clarity on how this technology achieves its remarkable effects. This sequence of actions illustrates the practical application of each component previously discussed.
- Designing the crRNA: Scientists first design an appropriate crRNA that complements the target DNA.
- Formation of the Complex: The crRNA binds with Cas proteins, forming a ribonucleoprotein complex.
- Target DNA Recognition: The complex scans and identifies the target DNA by matching the crRNA sequence.
- Cas Protein Action: Once the target is located, the Cas protein makes a double-strand break in the DNA.
- DNA Repair Mechanism: Following the break, the cell repairs the DNA, utilizing either non-homologous end joining or homology-directed repair.
- Outcome: The repair process can introduce desired genetic changes, effectively editing the target gene.
By understanding these mechanisms and processes, researchers and professionals can harness CRISPR technology for various applications, refining its use in medicine, agriculture, and more.
Applications of CRISPR Technology
The applications of CRISPR technology are vast and transformative. Its potential impacts feed into critical areas such as healthcare, agriculture, and biotechnological developments. Understanding these applications is essential for grasping how CRISPR reshapes these domains. The main benefits are its precision and ability to facilitate rapid advancements.
Medical and Therapeutic Applications
Gene Therapy
Gene therapy represents a significant stride in medical science. It focuses on correcting defective genes responsible for disease development. One key characteristic of gene therapy is its potential to treat genetic disorders at their root. This makes it a beneficial approach in the context of the article. The unique feature of gene therapy is its direct influence on the patient’s genome.
In cases like cystic fibrosis or muscular dystrophy, gene therapy offers hope for effective treatments. However, it has challenges, such as potential immune responses or unintended consequences in editing. Despite this, its innovative approach marks it as a crucial application of CRISPR technology.
Genetic Disease Prevention
Genetic disease prevention aims at reducing the incidence of hereditary conditions. CRISPR's role here is promising. One notable characteristic is the ability to edit genes before they lead to disease. Because of this, it is considered a beneficial strategy for prevention. By utilizing CRISPR to modify embryos or germline cells, it could help avert diseases such as Huntington's or certain cancers.
However, ethical concerns arise regarding the implications of editing germline cells. These include potential long-term effects on future generations. Balancing these concerns with the potential benefits is a significant challenge.
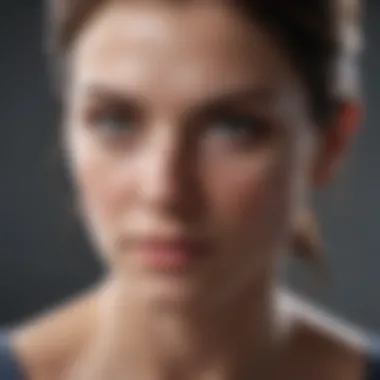
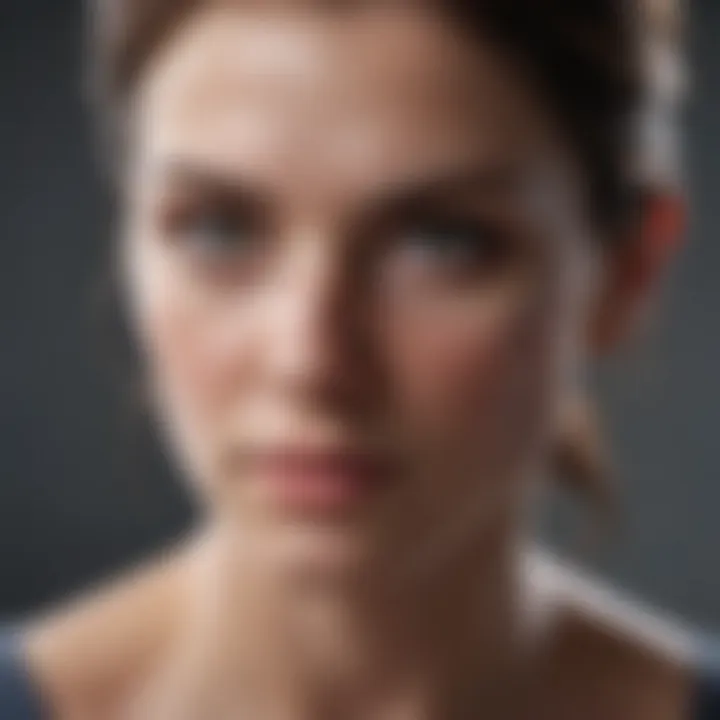
Oncology Applications
Oncology applications of CRISPR focus on cancer treatment strategies. By genetically modifying immune cells to target tumors more effectively, CRISPR presents an innovative approach. The key facet of oncology applications is personalized treatment options, enabling therapies tailored to individual tumor profiles.
This personalization makes CRISPR a potent tool in precision medicine today. However, risks involve the unpredictability of tumor responses and off-target effects. Continued research will be essential to overcome these hurdles and maximize benefits.
Agricultural Advancements
Crop Enhancement
Crop enhancement using CRISPR involves improving characteristics like yield, taste, and nutritional content. This application is highly relevant due to the growing demand for food. One important trait of crop enhancement through CRISPR is the speed with which modifications can be achieved. This aspect makes it a favorable choice for agriculture.
Unique features include the ability to create crops that are more resilient to climate change. However, concerns about ecological impacts and public perceptions of GMO foods remain. Addressing these issues is important for the future of CRISPR in agriculture.
Pest Resistance
Pest resistance is another vital agricultural application of CRISPR technology. This method enables crops to defend against common pests without chemical pesticides. The central feature is the enhancement of a plant’s natural defenses. This leads to reduced loss from pests, making it a beneficial solution for farmers.
Moreover, it can promote environmental sustainability. Nonetheless, challenges include potential resistance development among pests, which might diminish the effectiveness over time. Continuous monitoring and adaptation strategies must be part of the implementation process.
Sustainable Farming Practices
Sustainable farming practices utilize CRISPR to promote agricultural practices with minimal environmental harm. This application emphasizes efficient resource use and biodiversity preservation. The key characteristic here is the alignment with ecological principles, making it a valuable choice in modern agriculture.
Sustainable practices could lead to reduced water usage and lower carbon footprints. However, scalability and acceptance by farmers and consumers present obstacles. Changing entrenched practices requires not just technology but also education and policy support.
Biotechnological Innovations
Biomanufacturing
Biomanufacturing refers to using living cells to create products, aided by CRISPR technology. This area drastically enhances production efficiency and sustainability. One notable feature is the customization of microbial strains for specific product outcomes, making it a sought-after option in industry.
The advantages include lower costs and reduced waste production. However, standardization and regulatory compliance remain challenges. Establishing guidelines for biomanufactured products is crucial for wider acceptance.
Environmental Applications
CRISPR's environmental applications focus on ecosystem restoration and pollution control. One of the main aspects here is modifying organisms to mitigate environmental issues. This includes using modified bacteria to clean up oil spills or degrading plastics.
The key feature is the potential for significant ecological benefits, making it a beneficial application of CRISPR technology. Nevertheless, the ecological consequences of releasing modified organisms are not fully understood. Ensuring safety and efficacy will be essential to these applications.
CRISPR technology holds promise in various fields, from medical applications to environmental solutions. Its versatility signifies an exciting frontier in genetic applications.
Ethical Considerations Surrounding CRISPR
The introduction of CRISPR technology presents consequential ethical challenges. The power to edit human DNA holds immense potential, yet it raises numerous moral questions. It is essential to explore these concerns to ensure that gene editing aligns with societal values and human rights. As CRISPR becomes more accessible and widely used, discussions about its implications are becoming increasingly relevant. The ethical landscape of CRISPR is complex and multifaceted, needing careful consideration from all stakeholders involved.
Moral Implications of Gene Editing
Gene editing, particularly in humans, touches on fundamental moral questions. One critical issue is the potential for creating so-called "designer babies". This refers to the possibility of selecting desired traits, such as intelligence or physical appearance. While such advances could eliminate genetic disorders, they may also deepen social inequalities. The risk emerges that only certain demographics can afford enhancements, leading to a divide between the genetically privileged and others.
Another significant moral concern is the question of consent. In cases where modifications are made to embryos, future generations cannot express their preferences. This raises the question of whether it is ethically acceptable to alter someone's genetic makeup without their agreement. These moral implications highlight the necessity for a framework that considers not only the benefits but also the risks and ethical dilemmas involved in gene editing.
Regulatory Frameworks
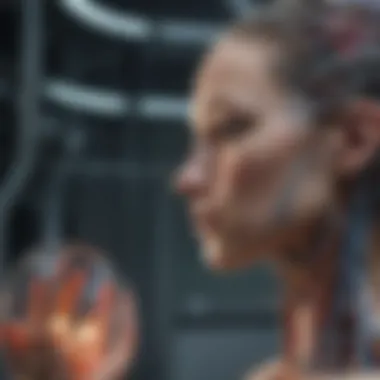
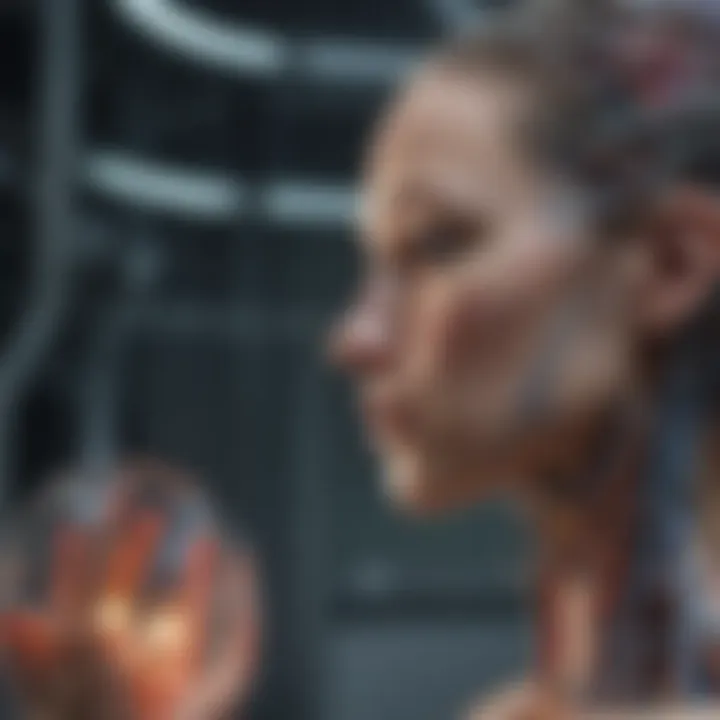
Regulatory frameworks play a crucial role in governing the use of CRISPR technology. They establish the boundaries within which research must operate, ensuring safety and ethical compliance. Varying guidelines have been proposed to navigate this complex terrain, and their effectiveness can significantly impact the development and application of CRISPR.
International Guidelines
International guidelines provide a shared understanding among nations about the ethical use of CRISPR. One significant characteristic of these guidelines is their emphasis on transparency and collaboration in research. This characteristic ensures that scientific advancements can benefit all of humanity, rather than being monopolized by a few countries or organizations.
The unique feature of international guidelines is their ability to set universal standards that transcend national boundaries. This facilitates a more equitable approach to gene editing. However, challenges remain, as some nations may not adhere to these guidelines, which can lead to unethical experimentation without oversight.
Nations' Policies on CRISPR Research
Nations’ policies regarding CRISPR research vary widely, reflecting differing societal values and ethical beliefs. Some countries, like Germany and Japan, maintain strict regulations to prevent unethical applications of gene editing. On the other hand, countries such as the United States and China are more permissive, facilitating rapid advancements but raising concerns about oversight.
The key characteristic of these policies is their adaptability. Countries are learning from each other's experiences and adjusting regulations accordingly. However, the unique feature also presents drawbacks. The lack of a cohesive, global policy can lead to research tourism, where scientists move to less regulated countries to pursue controversial experiments.
Overall, the interplay between moral implications and regulatory frameworks is essential for guiding the future of CRISPR technology. As the field of gene editing continues to evolve, it is vital to foster an ethical culture that prioritizes safety, consent, and fairness.
The Future of CRISPR Technology
The potential of CRISPR technology extends far beyond its current applications. As researchers delve deeper, the future of CRISPR gene editing unveils new possibilities that promise to transform genetics, agriculture, and medicine. Understanding these future implications is crucial, as it enables us to anticipate the benefits and ethical considerations associated with transformative gene editing technologies.
Emerging Trends in Genetic Editing
The landscape of genetic editing is evolving rapidly. Notable trends in CRISPR technology encompass the development of second-generation CRISPR tools, such as base editing and prime editing. Base editing allows precise changes to single DNA bases, minimizing errors. Prime editing enhances this further by enabling targeted insertions or deletions. This marks a shift from mere DNA modification to intricate gene rewriting capability.
Additionally, the integration of CRISPR with artificial intelligence is gaining traction. This collaboration enhances target recognition efficiency and reduces off-target effects. Such innovations promise to improve the overall safety and efficacy of gene editing, drawing more attention from researchers and the biotech industry. The enhancement of delivery mechanisms, such as nanoparticles and viral vectors, is also a significant trend. These advancements could make it easier to apply gene editing therapies in clinical settings.
Potential Challenges Ahead
Despite its remarkable promise, CRISPR technology faces several challenges. One primary concern is the precision of gene editing. Although improvements like base and prime editing address this, the possibility of off-target edits remains a significant risk. These unintended mutations can pose health risks in human applications, necessitating rigorous testing and validation.
Regulatory hurdles also present challenges. Governments and regulatory bodies grapple with establishing comprehensive frameworks that ensure safety while fostering innovation. The lack of universally accepted guidelines leads to fragmented policies across regions. This can hinder collaboration and slow down progress in gene editing research.
Ethical concerns further complicate advancements. Questions surrounding the modification of human embryos and the long-term effects of such interventions on future generations must be considered seriously. Society's acceptance of gene editing profoundly impacts research funding, which also influences the pace of innovation.
Impact on Society and Medicine
The societal implications of CRISPR technologies are profound. As advances in gene editing continue, we can expect significant changes in healthcare. The potential for personalized medicine becomes more tangible, allowing treatments tailored to individual genetic profiles. This shift could improve patient outcomes and reduce the costs associated with trial-and-error approaches.
Moreover, CRISPR holds the promise of eradicating genetic diseases. Conditions such as cystic fibrosis or sickle cell disease might become treatable or preventable, marking a substantial milestone in public health. Access to such technologies, however, raises questions about equity in healthcare. Ensuring that everyone can benefit from these innovations is crucial in discussions about future applications.
In agriculture, CRISPR could revolutionize how crops are engineered. With the ability to create drought-resistant plants or enhance nutritional profiles, the technology can contribute to food security. These advancements can help address global challenges such as hunger and malnutrition, but it is vital to balance environmental impacts with agricultural productivity.
"CRISPR technology offers unprecedented opportunities, but its future must be navigated with care to maximize benefits."
Epilogue
The conclusion of this article serves as a crucial synthesis of the discourse surrounding CRISPR gene editing technology. It provides the necessary recapitulation of the significant insights and findings explored in earlier sections. By emphasizing the importance of CRISPR, we can appreciate how it stands as a pivotal tool in modern science, bridging gaps between theoretical biology and practical applications.
Specifically, the historical context provided gives a clear backdrop to the tremendous advancement CRISPR represents in genetic engineering. Moreover, the exploration of its mechanism reveals the intricacies behind its functioning, making the concept of gene editing more accessible and understandable.
The applications discussed highlight the diverse fields benefiting from this technology, ranging from medicine to agriculture. These insights are essential for students, researchers, and professionals, as they contemplate the multifaceted implications of CRISPR in their respective disciplines.
Summarizing Key Takeaways
- CRISPR technology offers precise and efficient gene editing capabilities. It allows for targeted modifications in DNA, paving the way for advancements in treating genetic disorders.
- The applications of CRISPR are vast and varied. From medical therapies to agricultural enhancements, its potential impacts are impressive.
- Ethical considerations are paramount. The moral implications of altering genetic material raise important questions about the future of genetic engineering.
- CRISPR's future looks promising but is fraught with challenges. These challenges include regulatory hurdles and the societal implications of widespread gene editing.
Final Thoughts on the Role of CRISPR
In closing, CRISPR technology is not merely a scientific novelty. It represents a fundamental shift in how we approach genetic research. The potential it offers for both healing and enhancement is profound, yet it necessitates careful ethical considerations. As society grapples with these changes, it is imperative to foster informed discussions on the implications and regulations surrounding CRISPR applications. This will ensure that we harness the technology in ways that are beneficial and equitable, keeping in mind our collective responsibility toward future generations.