Exploring Astrocytes: Their Key Locations and Functions
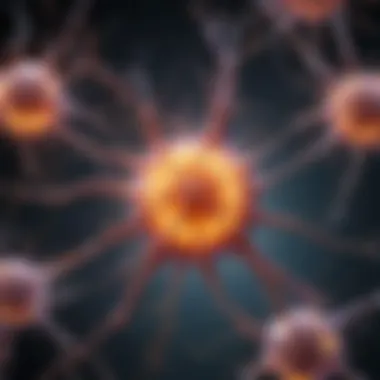
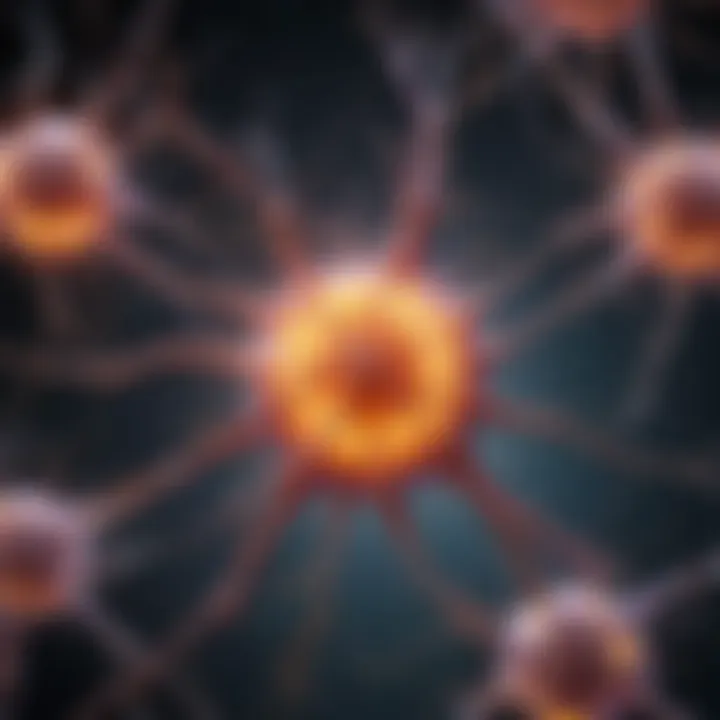
Intro
Astrocytes are fundamental components of the central nervous system (CNS). Their locations throughout the brain and spinal cord are not random; they are strategically distributed to fulfill their complex functions. These glial cells provide structural support, nutrient supply, and act as intermediaries between neurons and blood vessels. Understanding the specific locations of astrocytes helps to highlight their roles in maintaining neural homeostasis and influencing various neurological processes.
Research Overview
Summary of Key Findings
Recent research has illuminated the diverse locations of astrocytes across the brain and spinal cord. Astrocytes are present in all brain regions, with significant concentrations in the cortex, hippocampus, and cerebellum. Each region exhibits unique astrocytic characteristics, which correlate with the specific functional demands of that area. For example, astrocytes in the hippocampus are crucial for memory formation and retrieval, while those in the cortex are integral to sensory processing.
Furthermore, interactions between astrocytes and neurons are vital for synaptic plasticity. This relationship underlines the importance of astrocytic location.
Relevance to Current Scientific Discussions
The exploration of astrocyte locations contributes to ongoing discussions about neurodevelopment and pathology. For instance, research indicates that alterations in astrocyte distribution can lead to various neurodegenerative diseases. Conditions such as Alzheimer's and Parkinson's have been linked to dysfunctional astrocytes. The examination of astrocytic roles provides insights into potential therapeutic approaches aimed at restoring healthy neural environments.
Methodology
Research Design and Approach
This article synthesizes results from various studies, employing a comprehensive review methodology. The design involves analyzing both recent and foundational research regarding astrocyte function and localization. By integrating findings from histological studies, advanced imaging techniques, and genetic profiling, this work provides a thorough understanding of astrocytic distributions.
Data Collection and Analysis Techniques
Data collection involved multiple approaches:
- Immunohistochemistry: This method allowed for the visualization of astrocytes in different brain regions. It provided clear evidence of their spatial distribution.
- In vivo imaging: Techniques like functional MRI and PET scans were utilized to observe astrocytic activity in live subjects.
- Genetic markers: Specific markers were used to analyze astrocyte populations and their interactions with surrounding cellular structures.
These methods collectively yield a robust framework for understanding astrocyte locations and their implications for neuronal health and disease.
Understanding Astrocytes
Astrocytes are a crucial element of the central nervous system (CNS), representing a large population of glial cells. An understanding of these cells is vital for several reasons. Firstly, they ensure the proper functioning of neurons by providing structural support, which allows for efficient communication within the nervous system. Secondly, astrocytes play an active role in maintaining the homeostasis of the neural environment, including the regulation of ion concentrations and neurotransmitter levels. Moreover, the interactions between astrocytes and neurons significantly influence synaptic activity and neuroplasticity, which are essential for learning and memory processes.
It is important for researchers and students alike to explore the multifaceted roles of astrocytes. The insights gained from studying astrocytes extend to understanding various brain diseases and disorders, including neurodegenerative conditions and traumatic injuries. A nuanced comprehension helps in elucidating potential therapeutic targets that may arise from the dysfunction of these cells.
Definition of Astrocytes
Astrocytes, categorized under the glial cells, are star-shaped cells found throughout the brain and spinal cord. Their distinctive features include numerous processes that extend to envelop nerve cells and blood vessels, which facilitates various functions. Unlike neurons, which are primarily involved in transmitting signals, astrocytes contribute to the overall support and maintenance of the nervous system. They are involved in the formation of the blood-brain barrier and play a role in repairing injuries within the CNS.
General Functions of Astrocytes
Astrocytes perform several critical fonctions in the CNS:
- Structural Support: They provide a framework that supports neurons and blood vessels.
- Nutrient Supply: Astrocytes help transport nutrients from the blood to neurons, ensuring energy requirements are met.
- Maintenance of Extracellular Environment: These cells regulate ions, pH, and neurotransmitter levels, influencing the neuronal activity.
- Participating in Neurotransmission: Astrocytes modulate synaptic activity by releasing gliotransmitters, which can influence neurotransmitter release.
These functions highlight the essential roles astrocytes play beyond simply being supportive cells. Their involvement in various processes is fundamental to the healthy operation of the CNS.
Importance in the Central Nervous System
The significance of astrocytes in the CNS cannot be overstated. They are integral to sustaining neural tissue integrity and facilitating communication between neurons. For instance, astrocytic interactions help maintain the blood-brain barrier, which protects the brain from harmful substances in the bloodstream while regulating the transport of essential molecules. Additionally, astrocytes contribute to the repair process following CNS injury by proliferating and forming a scar that can hinder further damage.
In the context of neurodevelopment, these cells are crucial for regulating the formation and maturation of synapses. Their dysfunction can lead to various neurological issues, ranging from epilepsy to psychiatric disorders. The study of astrocytes thus offers insights into both their normal physiological roles and the implications of astrocytic changes during pathological states.
Anatomical Locations of Astrocytes
The anatomical location of astrocytes within the central nervous system is crucial for understanding their diverse functions. These cells are not randomly distributed; rather, their locations in various brain regions and the spinal cord support their roles in maintaining homeostasis, supporting neurons, and responding to injury. Exploring the anatomical locations of astrocytes helps to elucidate their functional specialization and interaction with other brain cells.
Cortex
Astrocytes in the cortex are primarily protoplasmic astrocytes, characterized by their extensive processes. Their main role here involves regulating the blood-brain barrier and modulating synaptic activity. These astrocytes support neuronal health by clearing glutamate from synapses and providing lactate as an energy source. Their strategic position aids in forming a network that integrates neuronal signals, reinforcing the cortex’s function in higher cognitive processing.
Hippocampus
In the hippocampus, astrocytes show a unique morphology that suits their role in learning and memory. They are instrumental in regulating neural plasticity through their interactions with synapses. Astrocytes in this region help maintain an optimal balance of neurotransmitters, particularly glutamate, enhancing synaptic efficiency. Data suggests a correlation between astrocytic function in the hippocampus and the development of cognitive deficits, highlighting their significance in neurodevelopment.
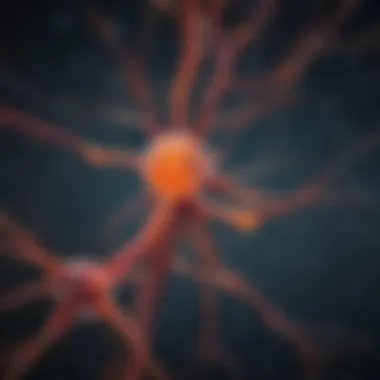
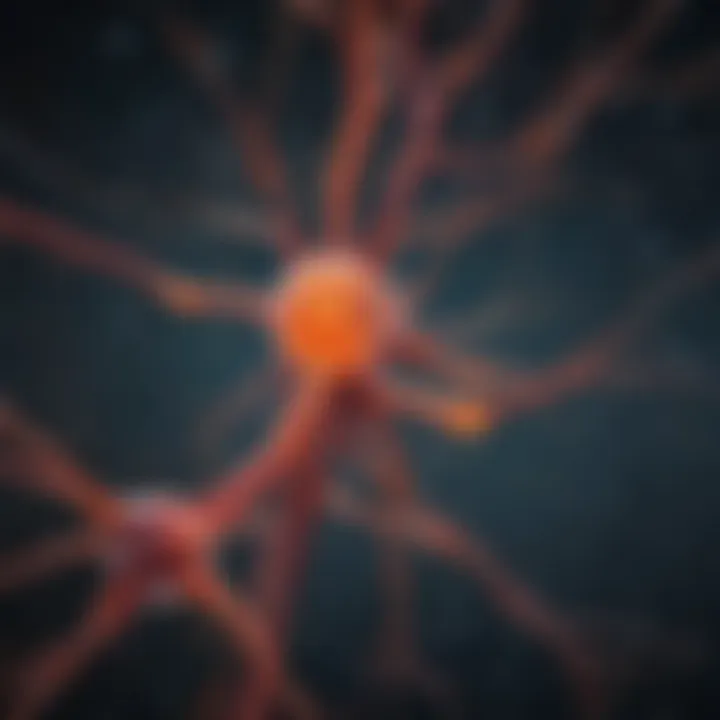
Thalamus
Astrocytes located in the thalamus serve as a crucial interface between sensory signals and cortical processing. They contribute to the modulation of synaptic transmission, affecting the integration of sensory inputs. Thalamic astrocytes play a role in wave activity during sleep, facilitating cerebral recovery. Their presence in a relay center of information reinforces their importance in attention and sensory perception.
Cerebellum
The cerebellum hosts a different subtype of astrocytes called fibrous astrocytes. These cells form a scaffold that supports Purkinje cells, which are essential for motor coordination. Astrocytes in the cerebellum also regulate ion balance and supply nutrients. The coordinated activities of cerebellar astrocytes contribute to motor learning and balance, underlying the cerebellum’s role in executing fine motor skills.
Basal Ganglia
In the basal ganglia, astrocytes have an essential part in modulating the dopaminergic system, which influences movement and reward signaling. They interact closely with neurons and regulate neurotransmitter levels, particularly dopamine. Astrocytic dysfunction in this area has been linked to disorders such as Parkinson's disease, indicating their role in both normal and pathological states.
Spinal Cord
Astrocytes in the spinal cord are involved in maintaining the environment surrounding motor and sensory neurons. They play a significant role in the repair process after spinal cord injuries by promoting neuroprotection and potentially reducing inflammation. Their presence is crucial for maintaining the signal transmission essential for reflex actions and sensory signaling. Understanding spinal cord astrocytes may provide insights into therapeutic approaches for neurotrauma.
Types of Astrocytes by Location
Understanding the various types of astrocytes by location is essential for comprehending their diverse roles within the central nervous system. Different astrocytic types exhibit unique structural and functional characteristics aligned with the demands of their specific environments. This section will explore three primary types: protoplasmic astrocytes, fibrous astrocytes, and radial astrocytes. Each type serves a distinct purpose and contributes to the overall functionality of neural networks.
Protoplasmic Astrocytes
Protoplasmic astrocytes are predominantly found in the gray matter of the brain. They are characterized by their star-like shape and possess numerous processes or extensions that interact closely with neurons, blood vessels, and other glial cells. These astrocytes play a vital role in maintaining the brain's microenvironment. Their primary functions include:
- Providing structural support to neurons.
- Regulating ion concentrations, particularly potassium and calcium, to maintain neuronal excitability.
- Modulating synaptic transmission by influencing neurotransmitter levels, especially glutamate.
- Facilitating the transport of nutrients from blood vessels to neurons.
In summary, protoplasmic astrocytes are essential for sustaining neuronal health and enhancing communication within neural circuits.
Fibrous Astrocytes
Fibrous astrocytes are primarily located in the white matter of the brain and spinal cord. They have a more elongated shape compared to protoplasmic cells, with fewer branching processes. The functions of fibrous astrocytes include:
- Providing structural stability to white matter tracts.
- Assisting in the repair processes after injury, aiding in the formation of glial scars.
- Maintaining homeostasis around axons, which is crucial for proper neuronal signal transmission.
Fibrous astrocytes also take part in the management of water and ions in the white matter regions which is critical for the overall functionality of white matter pathways.
Radial Astrocytes
Radial astrocytes, which are prominent during development, primarily act as scaffold-like structures. They are found in the embryonic and early postnatal brain. The key roles of radial astrocytes include:
- Guiding the migration of neurons during development.
- Contributing to the formation of the blood-brain barrier, establishing a protective interface between the brain and the circulating blood.
- Supporting the cerebral cortex's architecture.
Although their prominence decreases after development, their initial roles are vital for proper brain development and organization.
Understanding these types provides valuable insight into the ecological niche that each astrocyte type occupies, showcasing their specialized adaptations and key functions within the brain and spinal cord. The location of these astrocytes not only influences their morphology but also their interactions with neurons and other glial cells.
"The spatial distribution and specific types of astrocytes are fundamental to the neurophysiological processes that underpin cognition and behavior."
Functional Implications of Astrocyte Location
Understanding the functional implications of astrocyte location is essential in the exploration of their role in the central nervous system. The distribution of astrocytes within various regions influences their capacity to support neuronal function and maintain homeostasis. Astrocytes are not just passive support cells; their location allows them to engage actively in synaptic processes, nutrient transport, and neurotransmitter regulation. This importance is observable across different brain regions and can have considerable effects on neural health and disease.
Synaptic Support
Astrocytes provide synaptic support vital for proper neuronal communication. They envelop synapses and participate in the clearance of excess neurotransmitters such as glutamate. By scavening these neurotransmitters, astrocytes help prevent excitotoxicity, which could lead to neuronal injury or death. Their strategic positioning next to synaptic terminals also allows them to respond rapidly to neuronal activity. It has been observed that astrocytes can release gliotransmitters, which can modulate synaptic strength and plasticity, impacting learning and memory processes. This highlights the critical role astrocytes play in maintaining the balance of excitation and inhibition in the brain.
Nutritional Supply to Neurons
Astrocytes are essential in providing nutritional support to neurons. They uptake glucose from the bloodstream and convert it into lactate, which neurons can readily use. This lactate shuttle is especially significant during periods of high neuronal activity, where energy demands spike. Moreover, astrocytes also regulate ion homeostasis, maintaining the balance of potassium ions that is crucial during neurotransmission. Their location, particularly in regions with high neuronal activity, also accounts for their capacity to buffer excessive ion concentration changes, ensuring a stable environment for neurons to function effectively.
Regulation of Neurotransmitter Levels
The regulation of neurotransmitter levels is another critical functional aspect influenced by the location of astrocytes. They play a strong role in maintaining neurotransmitter homeostasis, notably by breaking down excess neurotransmitters like glutamate. Astrocytes also contribute to the recycling of neurotransmitters, ensuring a sustained supply for ongoing synaptic activity. Their interaction with neurons and other glial cells enables them to modulate signaling pathways effectively, adapting to various physiological and pathological states.
These functions underscore the need to consider astrocyte location when assessing their implications for overall brain function and health.
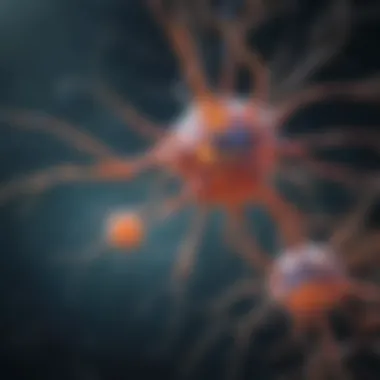
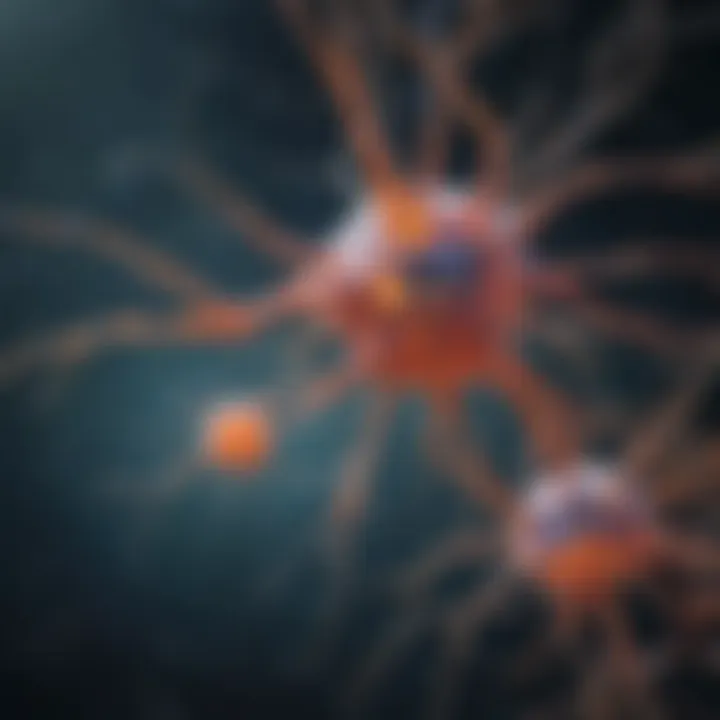
Overall, the functional implications of astrocyte location enhances our understanding of their multi-faceted roles in the central nervous system. Without acknowledging this spatial aspect, one cannot fully grasp their contributions to neural function and integrity.
Development and Migration of Astrocytes
The development and migration of astrocytes are critical components in the overall functioning of the central nervous system. Understanding these processes provides significant insights into how astrocytes contribute to neural homeostasis and respond to various physiological conditions. Moreover, their development is influenced by numerous factors, which can ultimately shape their functional capabilities and interactions within the neural environment. Therefore, this section aims to outline the key elements involved in astrocyte development and how their migration patterns affect their roles in both healthy and diseased states.
Astrogenesis Process
Astrogenesis refers to the formation of astrocytes from neural progenitor cells or astrocyte precursors. This process is essential during neural development and occurs predominantly during the early stages of brain formation. Factors such as growth factors and molecular signals orchestrate this transformation. For instance, the presence of transforming growth factor beta (TGF-β) and ciliary neurotrophic factor (CNTF) has been shown to promote the differentiation of progenitor cells into astrocytes.
Once these cells differentiate, they begin to adopt specific morphologies and functionalities based on their location in the brain. This adaptability is vital for their specific roles in supporting neurons. The impact of astrogenesis extends to synaptic support and modulation of the extracellular environment, thus playing a vital part in maintaining neural connectivity and health.
"Astrogenesis is not merely a precursor to astrocyte existence, but a pivotal event that ensures the functional plurality of astrocytes in various brain regions."
Influence of Environmental Factors
Various environmental factors critically influence the development and migration of astrocytes. These can include cellular interactions, physical conditions, and chemical signals present in their surroundings. For instance:
- The extracellular matrix (ECM) provides essential cues for astrocyte migration and orientation within the tissue.
- Inflammatory factors can change astrocytic behavior, leading to either neuroprotection or neurotoxicity, depending on the situation.
- The presence of other glial cells or neurons can facilitate communications that impact the proliferation and migration rates of astrocytes.
Additionally, the migratory patterns of astrocytes are often dictated by local cues such as injury signals. When tissues are damaged, astrocytes can migrate towards these regions, participating in the repair process, which is essential for regeneration. Thus, understanding these influences is crucial for researchers to develop interventions that could modulate astrocyte behavior in various neurological conditions.
Astrocytes in Pathophysiological States
Astrocytes play a crucial role in the response to various pathophysiological conditions. Their significance goes beyond mere support; they serve as active participants in brain health and disease. Understanding astrocytes in these states helps to delineate their multifaceted responses to injury, neurodegenerative changes, and tumor development. This section examines how astrocytes react in injury scenarios, their involvement in diseases like Alzheimer’s, and their relation to brain tumors, providing a comprehensive view of their importance in maintaining neurological functionality.
Astrocytic Activation in Injury
When neural injury occurs, astrocytes undergo a series of changes known as astrogliosis. This activation is vital for several reasons. First, activated astrocytes help seal off damaged areas, preventing further harm. They initiate a repair process by releasing several neurotrophic factors that support neuronal survival and regeneration. This includes factors like brain-derived neurotrophic factor (BDNF) and glial cell line-derived neurotrophic factor (GDNF).
Moreover, astrocytes regulate the extracellular environment. They manage ion balance, particularly potassium, and uptake excess neurotransmitters that may lead to excitotoxicity. Their ability to modulate inflammation is also critical, as abnormal astrocytic responses can exacerbate injury outcomes. Therefore, astrocytes serve a dual role: protective agents aiding recovery and potential sources of deleterious effects if their activation is uncontrolled.
Astrocytes in Neurodegenerative Diseases
Neurodegenerative diseases represent a significant challenge in understanding astrocyte function. In conditions like Alzheimer’s and Parkinson’s disease, astrocytes exhibit characteristics of chronic activation. This persistent activation, while initially protective, can lead to an inflammatory environment that contributes to neuronal death and disease progression.
In Alzheimer’s disease, for instance, astrocytes may accumulate amyloid-beta plaques. Their response can lead to neuroinflammation, which is detrimental to normal neuronal activity. Interestingly, some studies suggest that specific astrocytic functions, such as the uptake of amyloid-beta, may offer avenues for therapeutic intervention. Promoting healthy astrocytic activity might reduce plaque accumulation and mitigate the inflammatory response.
Relation to Brain Tumors
Astrocytes are integral in the context of brain tumors, particularly gliomas. These tumors arise from astrocytic cells, highlighting the importance of astrocyte behavior in pathology. Tumor-associated astrocytes often exhibit altered functions that facilitate tumor growth and malignancy. They can promote an immune-evasive environment, enabling tumors to grow unhindered by the body's defenses.
Furthermore, gliomas may manipulate astrocytic signaling and metabolic pathways to their advantage. This can include shifting astrocytes towards a more supportive role, inadvertently aiding in the nutrient supply necessary for tumor maintenance. Understanding these intercellular dynamics is essential. It opens the possibility of targeted therapies aimed at astrocytes to disrupt this supportive tumor microenvironment.
Key Insight: Astrocytes, while essential for neural protection and support, can complicate pathophysiological states. Their dual roles in both beneficial and harmful contexts underline their complexity in neuroscience research.
Experimental Techniques to Study Astrocyte Location
Understanding the intricate locations of astrocytes in the central nervous system is vital for deciphering their roles in neurological health. Various experimental techniques have emerged as essential tools in this endeavor. They offer unique insights into astrocyte behavior and distribution, enabling researchers to examine their contribution to neuronal function and pathology. This section will cover three primary techniques: immunohistochemistry, fluorescence microscopy, and in vivo imaging techniques. Each method provides distinct advantages and challenges, which researchers must weigh carefully in their studies.
Immunohistochemistry
Immunohistochemistry is a powerful technique used to visualize specific proteins in tissue sections. It employs antibodies that bind to target proteins associated with astrocytes. This method enables precise identification of astrocyte markers, supporting localization and understanding of their morphological properties.
One significant advantage of immunohistochemistry is its ability to provide detailed spatial information about astrocyte distribution in various tissues, including the brain and spinal cord. Moreover, the technique can be performed on archived tissue samples, facilitating studies on neurodevelopment and pathology.
However, certain challenges exist. The quality of tissue preparation impacts results considerably. Also, non-specific binding of antibodies can lead to unreliable data interpretation. Thus, careful optimization of protocols is essential.
Fluorescence Microscopy
Fluorescence microscopy is a technique widely used to visualize live cells and tissues. It allows for real-time observation of astrocyte dynamics. By using fluorescent markers, researchers can visualize astrocytic processes and their interactions with neurons in a living system. This approach facilitates a deeper understanding of the functional implications of astrocyte location.
This method's major benefit is the ability to perform time-lapse imaging, providing insights into dynamic processes, such as astrocyte migration and signaling. However, the complexity of developing specific fluorescent probes can pose challenges. Furthermore, photobleaching can affect long-term observations, necessitating careful planning of experiments.
In Vivo Imaging Techniques
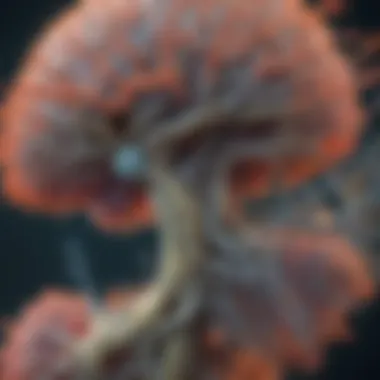
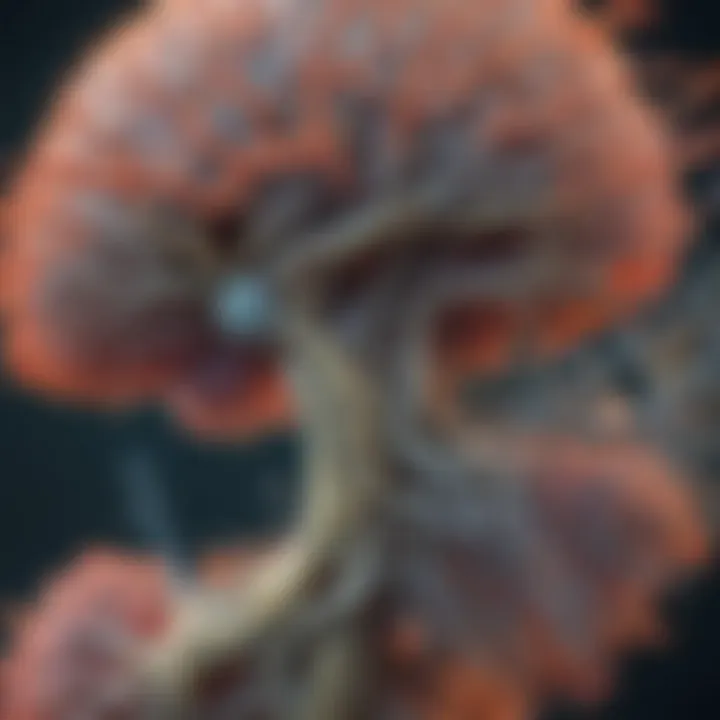
In vivo imaging techniques include various methods, such as magnetic resonance imaging (MRI) and two-photon microscopy. These techniques allow researchers to study astrocytes in their natural environment. In vivo imaging is crucial for studying the spatial organization of astrocytes in different pathophysiological states.
The main benefits of in vivo imaging are its capability for longitudinal studies and the non-invasive nature. It enables researchers to observe changes in astrocyte behavior over time, which is particularly important for understanding diseases and recovery processes. Yet, technical limitations remain, including resolution constraints and the need for specialized equipment.
In summary, each experimental technique discussed provides valuable insights into astrocyte location and function. By leveraging these methods, researchers can significantly advance our understanding of astrocyte roles in health and disease, ultimately informing therapeutic strategies.
Intercellular Interactions of Astrocytes
Astrocytes are not only essential cells for providing structural and metabolic support to neurons, but they also engage in intricate intercellular interactions. These interactions play a crucial role in the overall function and health of the central nervous system. The importance of studying these interactions extends beyond mere academic interest; it provides insights into fundamental neurological processes and potential therapeutic avenues for neurodegenerative conditions.
In the realm of astrocytes, two primary types of intercellular interactions can be examined: astrocyte-neuron interactions and astrocyte-astrocyte interactions. Understanding these relationships is paramount to comprehend how astrocytes contribute to neural network functionality and overall brain health.
"The interactions among astrocytes and between astrocytes and neurons contribute significantly to synaptic regulation, nutrient supply, and the maintenance of the blood-brain barrier."
Astrocyte-Neuron Interactions
The interaction between astrocytes and neurons is one of the most vital connections in the nervous system. Astrocytes surround synapses, providing an essential role in modulating synaptic transmission. They respond to neurotransmitters released from neurons, enhancing or inhibiting signals based on physiological needs. Through the release of gliotransmitters such as glutamate, ATP, and D-serine, astrocytes can influence neuronal excitability.
Moreover, astrocytes regulate the extracellular environment surrounding neurons. They help maintain ion balance, particularly potassium ions, which is crucial for neuronal signaling. Additionally, they transport glucose and lactate from blood vessels to neurons, ensuring a steady energy supply.
Key aspects of astrocyte-neuron interactions include:
- Nutrient support: Astrocytes uptake glucose and convert it to lactate, which neurons utilize for energy.
- Signal modulation: Gliotransmitters released by astrocytes can either enhance or suppress synaptic activity, contributing to synaptic plasticity.
- Ion homeostasis: Astrocytes absorb excess potassium from the extracellular space, preventing neuronal excitotoxicity.
These functions highlight how astrocytes are not merely support cells but active participants in neural communication and maintenance of homeostasis within the nervous system.
Astrocyte-Astrocyte Interactions
Astrocytes also engage in a variety of interactions with one another. These interactions are essential for the coordination of their overall functions and the maintenance of the neural environment. Astrocytes communicate through gap junctions, allowing for the passage of small signaling molecules and ions. This connectivity facilitates the sharing of information about local changes in the neuronal environment.
Furthermore, astrocyte-astrocyte interactions play a significant role in modulating inflammatory responses in the central nervous system. When faced with injury or disease, astrocytes can signal to each other, amplifying their reactive responses. This reflects a collective mechanism that can be beneficial in acute situations, such as after injury, but may contribute to pathological states in chronic conditions.
Notable aspects of astrocyte-astrocyte interactions include:
- Coordination of responses: Reactive astrocytes can work together to repair damaged tissue and restore homeostasis.
- Regulatory feedback: Interactions can serve as a feedback loop to enhance or reduce astrocytic activation depending on local needs.
- Subcellular communication: Gap junctions enable direct communication, allowing astrocytes to synchronize their activities effectively.
These interactions underscore the complexity of astrocytic function and their role in supporting not just individual neurons, but the entire neural network.
Understanding these intercellular interactions is critical for advancing our knowledge of astrogliosis in various pathological states and may guide future studies aimed at therapeutic interventions.
Future Directions in Astrocyte Research
The exploration of astrocytes and their various functions is an ongoing area of study, with multiple implications for neuroscience and medicine. Understanding the role of astrocytes in health and disease has become increasingly important. Advances in astrocyte research can potentially lead to novel therapies and deeper insights into the central nervous system's complex nature. With proper focus on this emerging field, researchers can unravel the intricacies of astrocytes, shedding light on their significance for overall neural functionality.
Technological Advancements
The landscape of astrocyte research is rapidly evolving due to technological innovations. These advancements have greatly enhanced our capability to visualize and manipulate astrocytes in vivo. Notable techniques include:
- Live-cell Imaging: This allows researchers to monitor astrocyte activity in real-time, providing insights into their dynamic responses in various physiological and pathophysiological conditions.
- Optogenetics: This method enables precise control of astrocyte activity, facilitating studies on their role in neurotransmission and synaptic plasticity.
- Single-cell RNA Sequencing: This technique allows for the characterization of gene expression profiles in individual astrocytes, revealing diverse functional subtypes and responses to environmental cues.
These technologies open new avenues for understanding astrocyte behavior and their interactions within neuronal circuits. Striving for technological advancement can reveal the complexities of astrocyte functions, ultimately assisting researchers in identifying potential therapeutic strategies.
Potential Therapeutic Targets
With a better understanding of astrocytes' roles, researchers are seeking therapeutic targets within these cells. Some promising areas of focus include:
- Modulating Glutamate Uptake: Astrocytes regulate glutamate levels in the synaptic cleft. Enhancing their uptake function may protect against excitotoxicity, which is a contributing factor in various neurological disorders like Alzheimer's disease.
- Inflammation Control: Astrocytes play a vital role in the central nervous system's inflammatory response. Targeting their signaling pathways could offer strategies to mitigate acute and chronic inflammation associated with brain injuries and neurodegenerative diseases.
- Astrocytic Metabolism: Harnessing the metabolic capabilities of astrocytes can lead to therapeutic strategies aimed at maintaining neural homeostasis, especially in conditions of metabolic stress.
"Astrocytes are not merely supportive cells; they are active participants in brain function and disease. Understanding their behavior can pave the way for innovative treatment options."
In summary, focusing on future directions in astrocyte research is crucial for uncovering their multifaceted roles in the nervous system. By tapping into technological advancements and identifying potential therapeutic targets, the scientific community can enhance its understanding of astrocytes and ultimately improve neurological health.
Closure
The exploration of astrocytes and their locations within the central nervous system holds significant importance. Understanding where these cells reside enhances our comprehension of their roles in maintaining neuronal health and overall brain function. Throughout this article, we have highlighted that the distribution of astrocytes is not random; it is intricately linked to the structural and functional demands of specific brain regions.
Several key points emerged in our discussion. First, each region in the brain, from the cortex to the cerebellum, features uniquely adapted astrocytic populations which perform specialized functions. This diversity suggests that the location of astrocytes is crucial not only for supporting neurons but also for modulating the local environment. Moreover, the significance of astrocytic interactions emphasizes their role in synaptic support and nutrient supply, thus ensuring homeostasis in neural activities.
In considering future directions for research, there are extensive implications that arise from the findings presented. The cellular mechanisms underlying astrocytic function in health and disease are still not fully understood. Continued investigation into the environmental influences on astrocyte behavior can offer valuable insights into potential therapeutic avenues. Developing targeted treatments for neurological disorders could greatly benefit from further elucidation of astrocytic roles across various brain regions.
"The spatial distribution of astrocytes is essential. It fosters not only neuronal health but also impacts neurological diseases directly."
In summary, the understanding of astrocytic locations and their consequential roles is pivotal. Researchers and clinicians can leverage this knowledge to inform strategies aimed at mitigating neurological impairment and enhancing brain health.