CRISPR and Cas9: Transforming Genome Editing Today
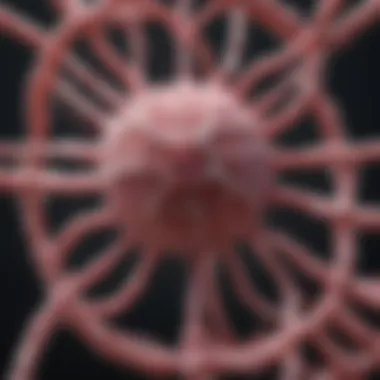
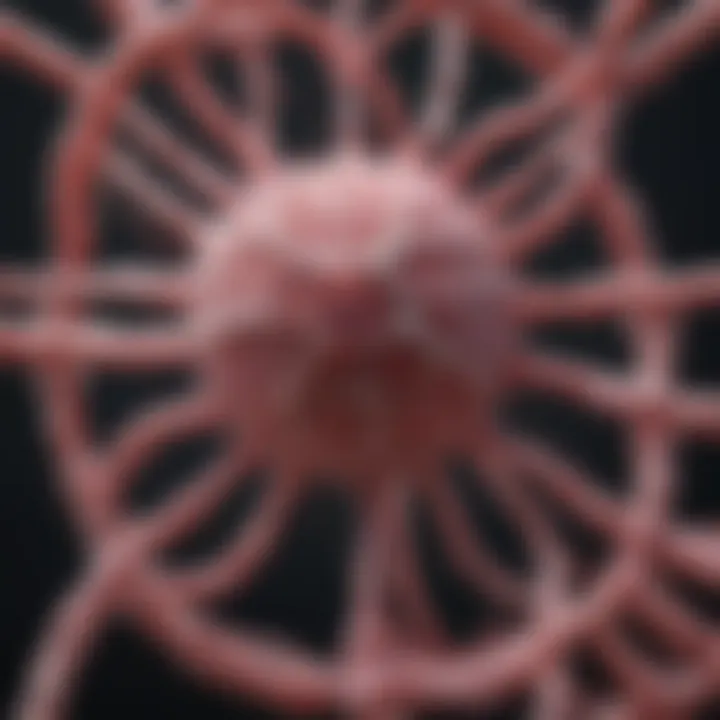
Intro
CRISPR-Cas9 has emerged as a revolutionary technology in the field of genetics. This innovative tool is reshaping how scientists approach genome editing. With its ability to target specific DNA sequences, CRISPR-Cas9 allows for precise modifications in the genetic code of various organisms, including plants, animals, and even humans. Understanding this technology is crucial for grasping its implications in medicine, agriculture, and ethics.
In recent years, CRISPR-Cas9 has made headlines due to its potential to treat genetic disorders, enhance crop resilience, and even combat diseases. The simplicity and effectiveness of this methodology distinguish it from previous genome editing tools. The marriage of CRISPR, which stands for Clustered Regularly Interspaced Short Palindromic Repeats, and Cas9, the associated protein that acts as a molecular scissors, forms an accessible platform for genomic modification.
As we proceed, this article will cover various aspects of CRISPR-Cas9 technology, including its fundamental mechanisms, applications across different sectors, ethical considerations surrounding its use, and the future landscape of genetic editing. A comprehensive exploration of CRISPR-Cas9 provides valuable insights into its role in modern genetics and its implications for society's progression.
Prolusion to CRISPR and CAS9
In contemporary genetics, CRISPR and Cas9 technologies have emerged as transformative forces. Understanding CRISPR and Cas9 is essential as they represent the forefront of gene-editing capabilities, enabling precision that was once thought unattainable. This introduction seeks to provide clarity about these remarkable tools, emphasizing their foundational significance within genetic science.
Defining CRISPR and CAS9
CRISPR stands for Clustered Regularly Interspaced Short Palindromic Repeats. This refers to a set of DNA sequences found in bacteria. These sequences function similarly to a genetic memory. Bacteria utilize them to remember and defend against viruses. Cas9, or CRISPR-associated protein 9, is an enzyme responsible for cutting the target DNA. Together, they form a system that allows scientists to alter DNA sequences in various organisms.
The combination of CRISPR and Cas9 provides a means to edit genes with a high degree of accuracy. This ability to modify the genome has significant implications, not just for scientific research, but also for medicine, agriculture, and biotechnology. Researchers can conduct experiments to understand genetic functions or potentially develop therapies for genetic disorders.
Historical Background
The discovery of CRISPR systems dates back to the 1980s. Scientists identified clusters of repeating DNA sequences in bacterial genomes. However, it wasn’t until the early 2000s that the potential of CRISPR became recognizable. In 2012, Jennifer Doudna and Emmanuelle Charpentier published a pivotal paper, demonstrating that CRISPR-Cas9 could be harnessed for targeted gene editing in eukaryotic cells. This development marked a significant leap forward in genetic engineering.
Following this revelation, the accessibility and efficiency of gene editing improved rapidly. The potential applications expanded dramatically, leading to broad interest from various fields. Health researchers began exploring ways to use CRISPR to combat genetic diseases, while agricultural scientists looked to enhance crop resilience and yield.
CRISPR-Cas9 technology has fundamentally altered the landscape of genetic research, making it an invaluable tool across disciplines.
Understanding both the mechanism and the historical context of CRISPR and Cas9 is critical. Each development in this technology carries the potential for significant societal impact, thus making it crucial for ongoing discourse within both scientific circles and public policy.
The Molecular Mechanism of CRISPR-Cas9
Understanding the molecular mechanism of CRISPR-Cas9 is crucial to appreciating its role in genome editing. By dissecting the structure and function of its components, one uncovers the intricate processes that allow this technology to make precise changes in DNA. The effectiveness and versatility of CRISPR-Cas9 stem from its origin and the evolutionary adaptations it exhibits, enabling nuanced applications in various fields, including medicine and agriculture.
Components of the CRISPR System
CRISPR RNA
CRISPR RNA, or crRNA, is a fundamental element of the CRISPR system. It acts as a guide that allows the Cas9 protein to locate a specific DNA sequence. The ability of crRNA to base-pair with complementary DNA is essential. This characteristic makes it easier for researchers to design crRNAs that match desired target genes. Its inherent flexibility also allows for rapid modifications; a new target can be programmed swiftly by changing the sequence of the crRNA. However, the specificity of crRNA is vital. Off-target effects can lead to unintended mutations, which is a significant disadvantage in research and therapy.
Trans-activating RNA
Trans-activating RNA, or tracrRNA, plays a supportive role in the CRISPR method. It enhances the efficiency of the crRNA by forming a complex with it. This interaction enables Cas9 to recognize and bind to the target DNA more effectively. The distinct feature of tracrRNA is its ability to stabilize the crRNA-Cas9 interaction. This stability ensures that the editing process proceeds smoothly. Nevertheless, the use of tracrRNA also introduces complexity in some applications, as it requires an additional component for successful genome editing.
Cas9 Protein
The Cas9 protein is perhaps the most recognizable component of the CRISPR system. It serves as the molecular scissors that execute the DNA cleavage. With its precise ability to create double-strand breaks in DNA, Cas9 allows for various outcomes, including gene knockout or insertion. One key characteristic of Cas9 is its ability to be made into several engineered variants, improving specificity and efficiency. However, advancements in Cas9 technology also bring challenges such as delivery mechanisms. In addition, ensuring its safe use in clinical applications requires careful consideration of potential off-target effects.
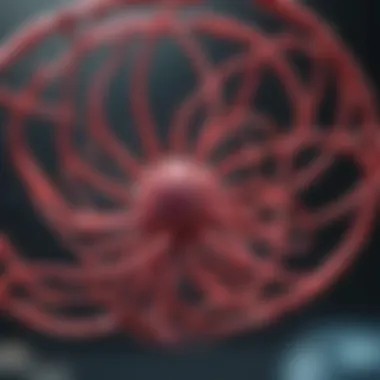
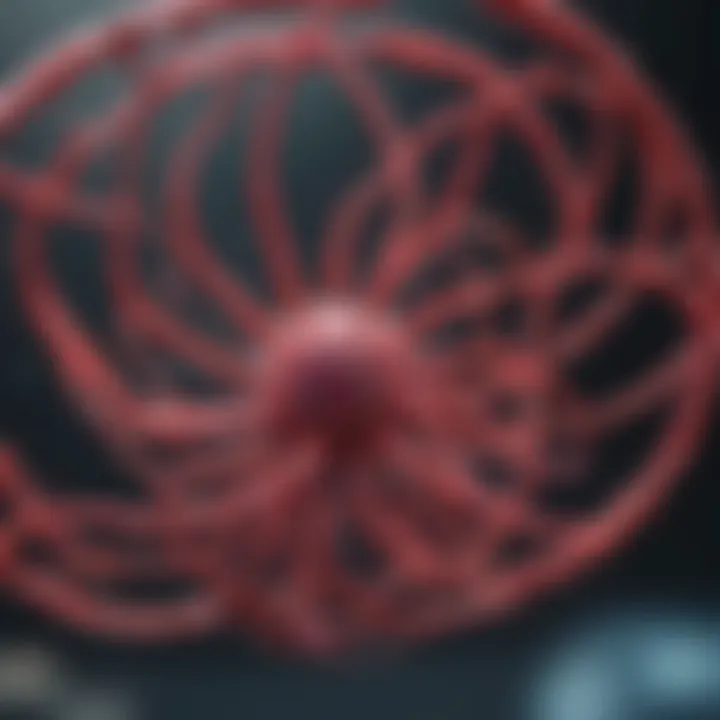
How CRISPR-Cas9 Works
The CRISPR-Cas9 system operates through a simple sequence of actions. Firstly, the crRNA identifies the target DNA sequence. The crRNA and tracrRNA combine to form a guide RNA that leads Cas9 to the exact location on the DNA strand. Once aligned, Cas9 introduces a cut, disrupting the gene and allowing for subsequent DNA repair mechanisms to kick in. This method can harness cellular machinery to insert, delete, or alter genes as needed. Overall, this process exemplifies an evolution of genome editing, showcasing technological potential and its balance with biological intricacies.
Targeting and Cutting DNA
Targeting and cutting DNA involves the decisive actions of the Cas9 protein once guided by the RNA. The precision of this process is essential for successful gene editing and minimizing unintended consequences. When Cas9 binds to the target DNA, it scans for the complementary sequence and aligns precisely before executing the cut. This ability to efficiently disrupt genes allows researchers to pinpoint genetic variations related to diseases, making this an indispensable tool in biomedical research.
Applications of CRISPR-Cas9 Technology
The application of CRISPR-Cas9 technology extends into multiple disciplines, reflecting its versatility and transformative potential. Understanding these applications is critical, as they underscore the significance of CRISPR in addressing real-world challenges ranging from healthcare to environmental management. Each area reveals how this simple yet powerful tool modifies our approach to genetics, contributing to innovation and solutions that impact society at large.
Biomedical Research
CRISPR-Cas9 has revolutionized biomedical research by simplifying gene editing. Researchers can now create precise modifications in the genome of various organisms. This capability accelerates the understanding of genetic diseases by allowing scientists to study the effects of specific mutations in model organisms. For instance, by knocking out genes in mice, researchers can observe disease progression or effectiveness of potential treatments.
The implications are profound. With CRISPR, it is possible to develop better in vitro models that mimic human diseases, thus enhancing the reliability of drug testing. More precisely, researchers can also generate genetically modified cells to explore cellular functions or screen for therapeutic targets. The increased efficiency of CRISPR compared to earlier methods like zinc-finger nucleases makes it an essential tool in laboratories.
Agricultural Biotechnology
In the realm of agricultural biotechnology, CRISPR-Cas9 is being applied to enhance crop resilience and yield. By editing specific genes, scientists can develop plants that tolerate environmental stresses such as drought or disease. This has essential implications for food security, particularly in regions vulnerable to climate change.
Moreover, CRISPR could allow for less reliance on chemical pesticides and fertilizers, promoting more sustainable farming practices. The technology enables precise changes, minimizing unintended traits that can arise from traditional breeding methods. This precision is particularly beneficial when developing crops that meet specific market demands or nutritional profiles without compromising plant health.
Potential Therapeutics
The therapeutic applications of CRISPR-Cas9 are some of the most anticipated advancements in modern medicine. By addressing the genetic basis of diseases, potential cures could emerge for conditions like cystic fibrosis, sickle cell anemia, and even certain types of cancer. Preliminary clinical trials are already investigating the feasibility of CRISPR-based therapies, demonstrating promising results.
However, potential therapeutics are not without challenges. The safety of gene editing in humans remains a primary concern. Off-target effects, where unintended edits might occur, pose significant risks. Therefore, rigorous evaluation of the techniques is critical before widespread implementation. Researchers must develop delivery methods that ensure precise targeting while minimizing complications. As techniques evolve, regulatory frameworks are likely to adapt as well, reflecting the need for ethical oversight in these emerging treatments.
The applications of CRISPR-Cas9 in various fields emphasize the technology's revolutionary potential, offering solutions across health and agriculture that could reshape our genetic approach to pressing global issues.
Ethical Considerations in CRISPR Research
The rapid advancement of CRISPR technology invites a thorough examination of its ethical implications. Addressing ethical considerations is crucial to ensuring responsible usage of genome editing. As this technology develops, the potential impact on society, individuals, and the environment becomes increasingly significant. Ethical discussion centers around issues such as consent, modification of human embryos, and the unforeseen consequences that might arise from editing the genetic code.
Gene Editing Ethics
Gene editing ethics deals with fundamental questions about who should have the authority to manipulate genetic materials. The core of this discussion is about the guidelines for responsible action. It is essential to consider the distinction between therapeutic and enhancement purposes. For example, treating genetic disorders through CRISPR is generally viewed more favorably than using the same technology for non-essential enhancements.
Concerns related to consent are also prominent. Gaining informed consent becomes complex when dealing with germline editing, which impacts not just the individual but future generations as well. The potential to create inequalities in society due to unequal access to these technologies raises critical moral questions. The possibility of 'designer babies,' where traits can be selected at will, challenges traditional views about the human experience and what it means to be human. This brings about the discussion of natural vs. artificial selection and the value of human diversity.
Regulatory and Safety Issues
Regulatory frameworks are critical in mitigating risks associated with CRISPR applications. Governments and organizations are tasked with developing robust guidelines that ensure safe practices in gene editing. Current regulations vary widely across countries, making it difficult to establish a cohesive global policy.
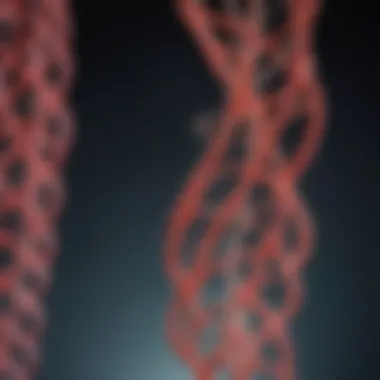
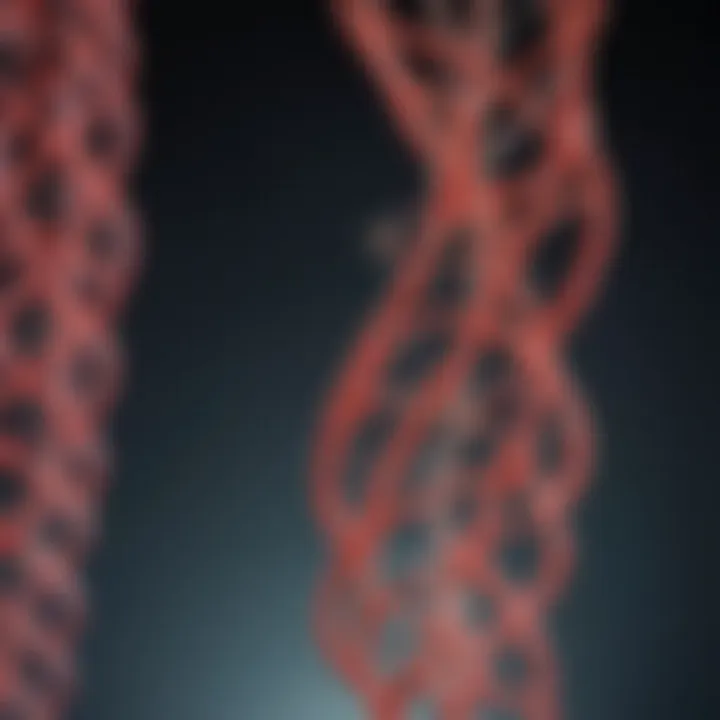
Safety issues must also be heavily prioritized. There is always a risk of off-target effects when utilizing CRISPR. These unintended modifications can lead to harmful consequences, making stringent testing and oversight essential. Approaches to address these challenges include:
- Developing improved versions of CRISPR that reduce off-target activity.
- Mandating rigorous evaluations before clinical applications.
- Formulating international agreements to create consistency in ethical practices.
"The ethical landscape of CRISPR-Cas9 technology evolves rapidly as advances are made. Discussions surrounding its implications are essential for any significant progress to be made responsibly."
Ultimately, the ethical discourse surrounding CRISPR technology plays a vital role. It not only ensures that the research aligns with societal values but also fosters trust among the public. As genome editing continues to develop, ethical considerations must remain at the forefront of research and application.
Emerging Trends and Future Directions
The field of CRISPR-Cas9 technology is rapidly evolving. New methods and applications are continuously emerging, driving the potential of this genome editing tool to new heights. Understanding these trends is crucial. For researchers and practitioners, staying updated is not just beneficial but essential for advancement in various domains, such as medicine and agriculture.
Advancements in CRISPR Techniques
Base Editing
Base editing represents a significant leap forward in precision for genome editing. Unlike traditional CRISPR approaches, which cut both strands of DNA, base editing enables the conversion of one DNA base pair into another without breaking the double helix. This characteristic of base editing allows for more subtle modifications in the genome. As a result, it minimizes the risk of unintended mutations.
One key aspect is the ability to correct point mutations, which are responsible for a large number of genetic disorders. This method is a beneficial choice in therapeutic contexts. However, there are also disadvantages. Base editing still requires considerable optimization to ensure efficient delivery and expression within human cells.
Prime Editing
Prime editing is another groundbreaking advancement. It aims to provide a more versatile and precise approach to gene editing. Prime editing differentiates itself by allowing for the insertion, deletion, or replacement of sequences with high accuracy. This method is particularly valuable for correcting a wide range of genetic mutations.
The unique feature of prime editing lies in its flexibility. It can target multiple gene sequences in a single operation, broadening its applicability compared to earlier methods. However, the technology is still in the early stages, and challenges remain. For instance, efficient delivery to cells and potential off-target effects are areas needing further investigation.
CRISPR-Based Diagnostics
CRISPR-based diagnostics are reshaping how we detect diseases. This technology uses the CRISPR mechanism to identify specific DNA or RNA sequences associated with various pathogens. The rapid and accurate nature of these diagnostics points to a significant advantage in medical testing.
The standout characteristic of CRISPR diagnostics is their sensitivity. They provide results in a fraction of the time required by traditional tests. However, challenges exist in terms of regulatory approval and standardization. Ensuring that these tests meet stringent quality controls is essential before they can be widely implemented.
CRISPR in Synthetic Biology
Synthetic biology, a burgeoning field, is experiencing a transformative influence from CRISPR technology. By harnessing CRISPR, scientists are designing and constructing new biological parts, devices, and systems. This integration is essential for developing innovative solutions to complex problems, such as sustainable biofuel production or biomaterials.
The collaboration between CRISPR and synthetic biology offers vast potential. It facilitates the engineering of organisms to carry out specific tasks, improving efficiency in research and industrial applications. Despite its promise, significant hurdles remain. Concerns about ethics and environmental implications must be addressed as these technologies progress.
CRISPR technology is leading the charge towards a new era of genome editing, with advancements paving the way for unprecedented possibilities in various biological fields.
Challenges Facing CRISPR Technology
The discussion surrounding CRISPR technology is often filled with both optimism and concern. This section aims to highlight the significant challenges that the field faces. Important aspects include technical limitations and the public's perception, which can greatly impact the future development of CRISPR applications. Addressing these challenges is crucial for the responsible advancement of genome editing techniques.
Technical Limitations
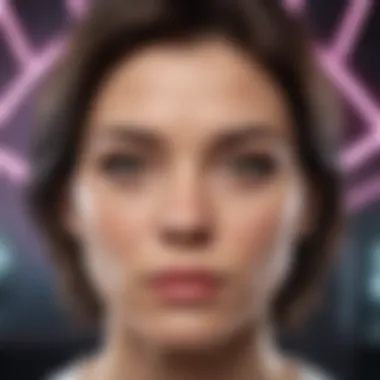
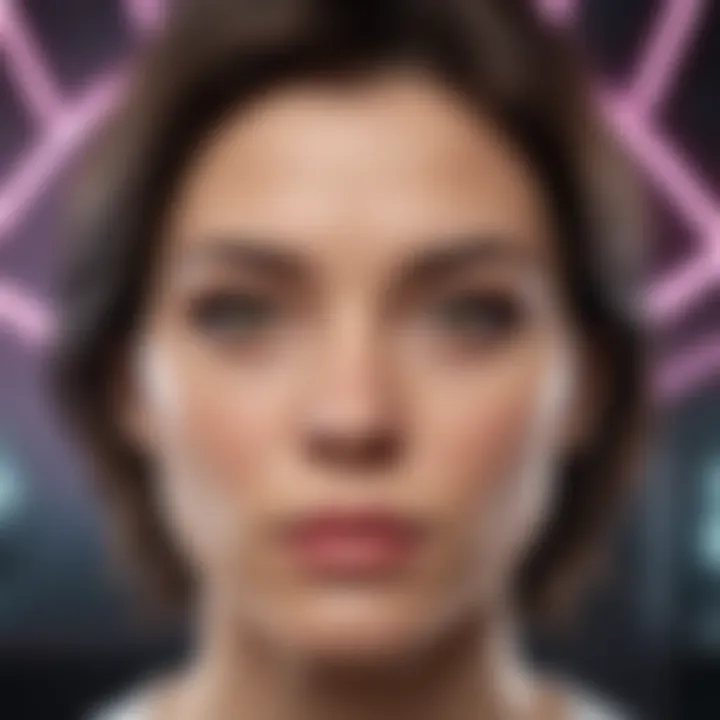
While CRISPR offers powerful genome editing capabilities, it does have some technical limitations.
- Off-target effects: One of the major concerns is the unintended modifications to the genome. This can lead to unintended consequences, making it difficult to guarantee safety in therapeutic applications.
- Delivery mechanisms: Efficiently delivering the CRISPR components to the targeted cells remains a challenge. Current methods, such as viral vectors, have limitations and can introduce additional complications.
- Complexity of traits: Some traits are influenced by multiple genes. Targeting a single gene may not lead to the desired outcome when several are involved in a particular biological process.
- Ethical concerns over germline editing: Editing germline cells raises questions about heritable changes. These changes affect future generations, increasing ethical concerns within society.
Public Perception and Misconceptions
Public understanding of CRISPR technology varies, often influenced by media portrayals and hearsay. Misconceptions can hinder the acceptance and development of this technology.
- Fear of the unknown: Many people fear genetic editing due to lack of understanding. This fear can stem from misconceptions regarding the safety and implications of such interventions.
- Biomedical and ecological consequences: Concerns arise about potential negative impacts on ecosystems if gene drives are introduced. Public unease can lead to restrictive regulations that slow research.
- Frankenstein fears: References to 'designer babies' misrepresent the technology's aims and capabilities. This perception can lead to polarized opinions and resistance in discussing its benefits.
- Importance of clear communication: Educating the public about science can help bridge gaps between scientists and laypeople. This therfore enhances trust and fosters informed discussions about CRISPR technology.
"Understanding scientific advancements is crucial for societal acceptance. Misconceptions can stifle innovation and progress."
Overall, addressing both the technical limitations and public perception surrounding CRISPR-Cas9 is essential. Only through careful navigation of these challenges can CRISPR technology reach its full potential in ethical and beneficial applications.
Case Studies of CRISPR Applications
The exploration of CRISPR applications unfolds through tangible case studies, illuminating the real-world potential and transformative impacts of this genetic technology. By examining specific instances where CRISPR-Cas9 has been applied, we gain insights into its capabilities and limitations. These case studies showcase the practical uses of CRISPR, aiding in understanding its role in diverse fields like medicine and agriculture.
Successful Clinical Trials
Successful clinical trials represent a critical aspect of CRISPR research, highlighting how this technology can be applied to address serious health conditions. For instance, researchers have conducted trials targeting genetic disorders such as sickle cell anemia and beta-thalassemia.
In one notable study, scientists used CRISPR to modify patients' cells and then reinfused them into the body. Results indicated a significant reduction in disease symptoms, demonstrating CRISPR's potential to create lasting solutions for genetic diseases.
Additionally, trials investigating cancer treatments illustrate the adaptability of CRISPR technology. In ongoing research, CRISPR is used to edit immune cells, enhancing their ability to target and destroy cancer cells. This approach shows promise, but also raises challenges related to safety and efficacy. These cases underline the need for comprehensive evaluations and regulatory considerations going forward.
Regenerative Medicine
CRISPR's significance in regenerative medicine cannot be overstated. The ability to edit genes offers profound implications for repairing damaged tissues and organs. One prominent example includes using CRISPR technology to develop treatments for degenerative diseases like muscular dystrophy and spinal cord injuries.
In research laboratories, scientists have successfully utilized CRISPR to restore function in models of muscular dystrophy by correcting gene mutations responsible for muscle deterioration. Such advancements could lead to groundbreaking therapies for conditions that currently lack effective treatment options.
Furthermore, CRISPR is being studied for its role in enhancing stem cell therapy. Researchers are investigating how gene editing can improve the safety and effectiveness of stem cells, potentially leading to more successful transplantation outcomes.
"The applications of CRISPR in regenerative medicine hold the promise of not just treating symptoms, but potentially curing diseases at the genetic level."
Overall, these case studies demonstrate CRISPR's transformative potential across various medical fields. They highlight not only the benefits but also the ethical considerations and challenges that must be addressed. As research continues, the outcomes from these studies will play a crucial role in shaping future applications of CRISPR technology.
Culmination
The conclusion serves as a vital component of this exploration into CRISPR and Cas9 technology. It summarizes the complex discussions about genetic editing, laying out the implications and potential future trajectories of this tool. By revisiting the key points from the previous sections, it reinforces the importance of understanding both the practical applications and the ethical dimensions associated with genome editing.
The Future of Genetic Editing
As we move forward, the future of genetic editing appears promising yet challenging. Emerging techniques such as prime editing and base editing enhance precision, offering solutions to previously intractable genetic disorders. These advancements may pave the way for therapies tailored to individual genetic profiles, transforming treatment paradigms in medicine. Furthermore, the integration of CRISPR into synthetic biology could lead to novel biotechnological applications, permitting tailored organisms designed for specific tasks—ranging from environmental remediation to advanced agricultural practices.
Researchers must remain vigilant about responsible innovation. Oversight, ethical considerations, and an informed public discourse will be essential to navigate the complexities introduced by these technologies. Keeping the lines of communication between scientists, ethicists, and the general public open will help ensure that genetic editing technologies are used to benefit society as a whole.
Final Thoughts on CRISPR's Impact
CRISPR-Cas9 technology is more than just a tool; it is a catalyst for profound changes in genetic research and application. Its ability to edit genes with unprecedented precision has already begun to reshape fields like medicine, agriculture, and beyond. However, it is critical to recognize that these advancements come with responsibilities. The potential for unintended consequences—whether ecological, ethical, or social—remains a relevant concern.
In summary, while the impact of CRISPR will continue to expand and evolve, it is the balance of innovation with ethical responsibility that will ultimately define its role in our future. The path forward necessitates careful consideration of the broader implications of genetic editing, ensuring that progress is not only scientific but also aligned with the values o f society.