XRF Analysis Procedure: A Comprehensive Overview
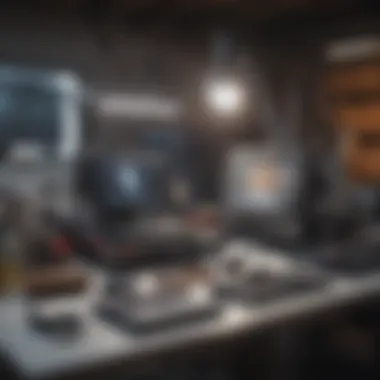
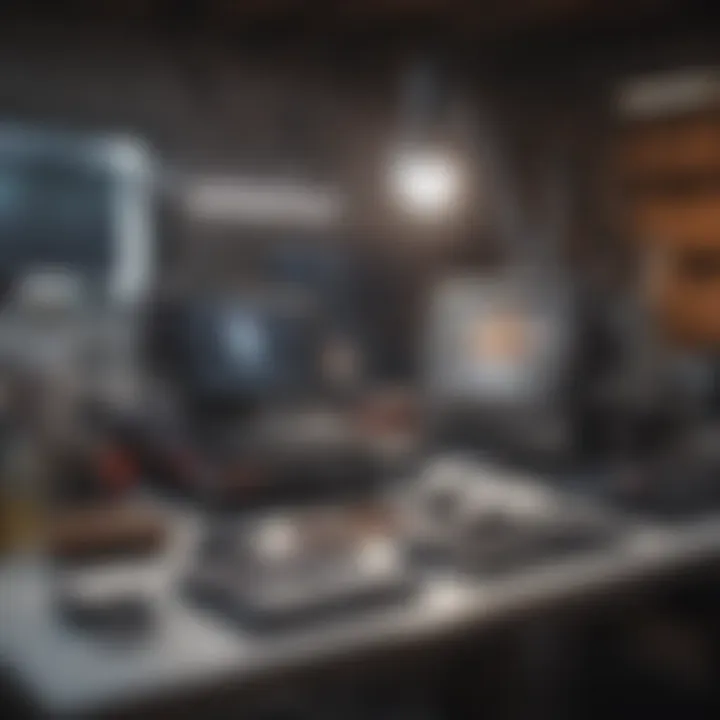
Intro
X-ray fluorescence (XRF) analysis is a significant technique within materials science, facilitating the elemental analysis of various samples. It leverages the principles of fluorescence to provide detailed insights into the composition of materials. As researchers and professionals delve into this method, understanding the procedure and its applications becomes crucial. This article will unpack the XRF analysis procedure comprehensively, starting from the basic concepts to the more intricate aspects.
Research Overview
Summary of Key Findings
The XRF analysis procedure has evolved significantly over the years. Recent advancements have increased its accuracy and range of applications. Key findings indicate that proper sample preparation is essential for effective analysis. Moreover, calibration techniques have shown to enhance the reliability of results, while data interpretation plays a vital role in extracting useful information from the collected data.
Relevance to Current Scientific Discussions
In the current landscape, XRF technology addresses critical challenges in various scientific domains. As environmental regulations tighten, the demand for accurate elemental analysis has never been higher. Professionals in chemistry and materials science are exploring how XRF can be applied to fields such as archaeology, geology, and even crime scene investigations. Thus, discussions around its methodologies and potential limitations are increasingly relevant.
Methodology
Research Design and Approach
The research design for studying XRF involves a thorough examination of its procedural steps. It encompasses defining objectives, selecting appropriate methodologies, and integrating findings to showcase the broader implications of XRF analysis. This structured approach aids in comprehending how each facet of the procedure contributes to accurate analysis.
Data Collection and Analysis Techniques
Data collection with XRF typically involves the following:
- Sample Preparation: Ensures the sample is ready for analysis and free from contamination.
- Calibration: Adjusts the instrument to measure the elemental composition accurately.
- Data Acquisition: Involves collecting data using the XRF instrument.
Once data is acquired, analysis techniques are employed. These include:
- Comparing results against known standards.
- Utilizing software for spectral interpretation.
- Validating findings through repeat testing to ensure accuracy.
Proper calibration and meticulous sample preparation are key in enhancing the accuracy of XRF results.
Foreword to XRF Analysis
X-ray fluorescence (XRF) analysis is a significant technique widely used in materials science and other scientific disciplines. It allows researchers to determine the elemental composition of materials quickly and effectively. Understanding XRF is crucial for anyone involved in scientific research or industry applications where material analysis is required. Its relevance extends across various fields, such as environmental monitoring, metallurgy, and cultural heritage conservation.
The importance of XRF analysis lies in its non-destructive nature and capability for rapid assessment. Unlike methods that may alter the sample being analyzed, XRF retains the integrity of the material, providing insights without damage. This is particularly valuable when dealing with precious artifacts or sensitive environmental samples.
In this section, we delve into the fundamentals of X-ray fluorescence. We will explore specific elements and benefits, including a comprehensive understanding of how samples are selected and prepared, the calibration process, execution of analysis, and subsequent data collection methods. The significance of these aspects cannot be underestimated, as they contribute to the accuracy and reliability of the results.
Understanding XRF provides a solid foundation for tackling real-world problems and contributes to advancing research methodologies. For students, researchers, educators, and professionals, grasping the essentials of XRF underpins their ability to leverage this technology effectively in their work.
Importance of grasping the principles of XRF:
- Enables effective application in diverse fields.
- Supports innovation in analysis techniques.
- Strengthens the results from scientific experimentation.
Understanding X-ray Fluorescence
X-ray fluorescence occurs when a sample is irradiated with high-energy X-rays or gamma rays. This energy causes the sample atoms to become excited and emit secondary X-rays specific to their elemental composition. The emitted rays can be detected and measured to identify and quantify the elements present in the sample. This process provides a powerful method for elemental analysis.
XRF can be used to analyze liquid, solid, or powdered samples in various environments. The technology is flexible and adaptable, allowing for both laboratory and in-field applications. This versatility enhances the method's utility across different sectors, effectively facilitating quick assessments in complex scenarios.
Historical Context and Development
The origins of X-ray fluorescence date back to the early 20th century, with foundational research conducted by physicists exploring the interactions of X-rays with matter. Over the decades, advancements in technology have led to refinement in XRF techniques, improving sensitivity and detection limits.
Notably, the introduction of energy dispersive spectrometry (EDS) and wavelength dispersive spectrometry (WDS) further enhanced the capabilities of XRF analysis. These developments brought about greater precision in elemental detection and quantification, thus broadening the application scope for this analytical method.
The ongoing evolution in instrumentation and software has also accelerated the adoption of XRF in various industries, making it an essential tool for scientists and professionals alike.
Fundamental Principles of XRF
Understanding the Fundamental Principles of XRF is crucial as it lays the groundwork for any detailed analysis using X-ray fluorescence. Comprehension of these principles enhances operational effectiveness and contributes to accurate results. Knowing how x-ray emission works and distinguishing between the types of XRF methods can significantly impact an analysis's outcome. Understanding these principles will not only enrich the reader's knowledge but will also familiarizes them with the terms and concepts that come into play during the XRF analysis procedure.
Principle of X-ray Emission
X-ray fluorescence analysis centers on the principle of x-ray emission. When a material is irradiated with high-energy x-rays, elements within the sample absorb some of this energy. This absorption leads to the ejection of inner shell electrons from the atoms of the material, causing a vacancy. Other electrons from higher energy shells transition down to fill these vacancies. In this transition, energy is released in the form of characteristic x-ray photons. Different elements emit x-rays at specific energies, which allows for elemental identification. This technique establishes a link between the acquired fluorescence spectrum and the elemental composition of the examined sample.
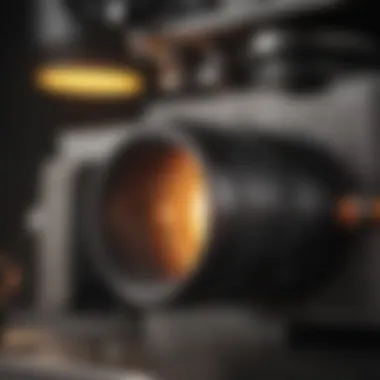
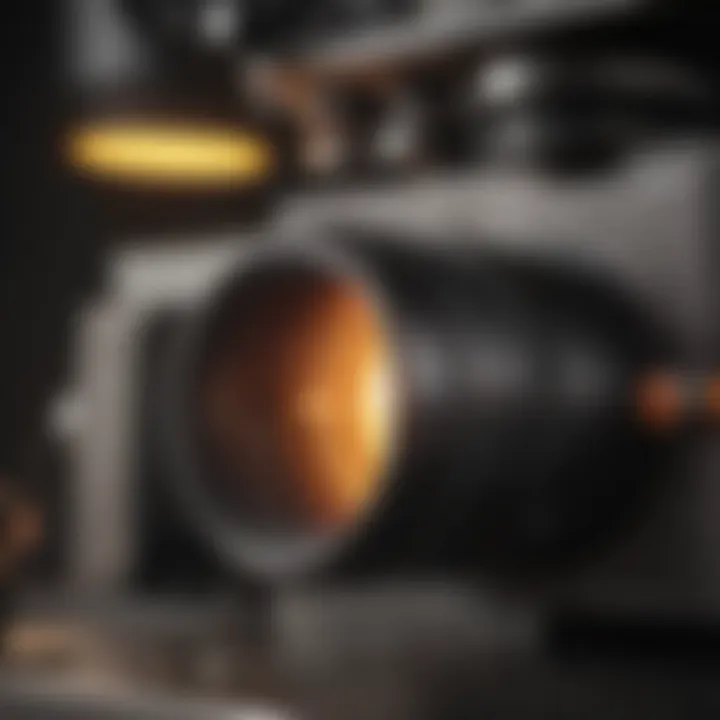
Understanding this principle is vital because it delivers insight into detecting various elements. Each element emits at distinct wavelengths. Hence, analyzing these emissions allows researchers to ascertain qualitative and quantitative data about the sample. The efficiency of the x-ray emission process is influenced by several factors. These include the x-ray source's energy, the sample's composition, and the detector's characteristics. The precise interpretation of the emitted energy peaks is instrumental in the analysis and requires a well-calibrated system.
Energy Dispersive XRF vs. Wavelength Dispersive XRF
When it comes to x-ray fluorescence, two predominant techniques exist: Energy Dispersive XRF and Wavelength Dispersive XRF. Understanding the differences between these two is essential for selecting the most appropriate method for a specific analysis.
- Energy Dispersive XRF (EDXRF): This method detects x-rays based on their energy levels using a solid-state detector. It is known for its simplicity and rapid analysis, making it popular for various applications. EDXRF can simultaneously measure multiple elements and is effective for bulk material inspections. The setup is less complex compared to WDXRF, allowing for more portable equipment and quicker results. However, while its detection limit is decent, it may not be sensitive enough for trace element analysis in certain materials.
- Wavelength Dispersive XRF (WD-XRF): In contrast, WDXRF operates by dispersing the emitted x-rays using a crystal or a diffraction grating. This approach allows for the measurement of x-rays at very precise wavelengths, yielding better resolution and sensitivity. WDXRF is particularly effective for analyzing samples with closely related elemental compositions and for samples where high precision is required. However, WDXRF systems are typically larger, more expensive, and take a longer time to acquire results.
Both methods have unique advantages and are used based on specific project requirements. Knowledge of these techniques enables researchers and professionals to make informed decisions when choosing the appropriate method for elemental analysis.
"Understanding the fundamental principles of x-ray fluorescence analysis is essential for anyone looking to utilize this powerful technique in their scientific work."
By comprehending these basic principles, readers will be better equipped to navigate the intricacies of XRF technology and its applications. The understanding gained here serves as a solid foundation for later sections of the article, particularly in the context of XRF analysis procedures.
XRF Analysis Procedure
Understanding the XRF analysis procedure is crucial for obtaining reliable elemental data. This process provides a standardized approach to sample evaluation, ensuring consistency and accuracy. Proper execution of this procedure can yield significant insights into material composition, which is essential across various scientific and industrial fields.
Factors such as the choice of samples and meticulous calibration of instruments can greatly influence results. This section will detail the vital steps involved in the XRF analysis procedure to ensure effective application in your research or work.
Sample Selection Criteria
Selecting the appropriate samples for XRF analysis is fundamental. The selected samples should reflect the material's overall composition and characteristic features. It is important to consider the sample type, which can vary significantly, such as solids, liquids, or powders.
- Homogeneity: Ideally, samples should be uniform and representative of the larger material.
- Size: The size of the sample should fit the XRF equipment's specifications to ensure optimal results.
- Surface Preparation: Clean, flat surfaces improve data accuracy, overcoming surface contaminants.
- Moisture Content: Excessive moisture can lead to low-energy absorption, affecting analysis.
Preparation of Samples for XRF
Effective sample preparation is essential for accurate XRF analysis. The samples should be treated carefully to avoid contamination or changes in composition. Here are some common preparation methods:
- Grinding: For solid samples, fine grinding can enhance surface area and uniformity.
- Pelletization: This involves compressing powdered samples into pellets, allowing for direct measurement.
- Drying: Samples should be dried appropriately to eliminate moisture, especially for hygroscopic materials.
The preparation phase lays the groundwork for successful analytical results, making it imperative to follow protocols rigorously.
Calibration of XRF Equipment
Calibration of the XRF equipment is a critical step. It ensures that the measurements are accurate and reliable. Calibration should be performed regularly and involves the following:
- Standard Samples: Using certified reference materials to create a calibration curve.
- Background Correction: Adjusting for any background interference that might skew results.
- Energy Calibration: This ensures that the X-ray detector is responding correctly across its energy range.
Each calibration should be documented meticulously, allowing for traceability and reproducibility in future analyses.
Conducting the Analysis
When conducting the analysis, it is pivotal to ensure that all parameters are set correctly. Following the calibration, the sample is placed in the XRF equipment. The analysis proceeds with the following considerations:
- Measurement Time: Longer measurement times can increase data quality but may also lead to sample changes (for some materials).
- Positioning: Accurate positioning of the sample in the beam is essential.
- Environmental Factors: Minimize vibrations, air currents, and other disturbances for more stable readings.
Attention to these details will enhance the integrity of the results produced.
Data Collection Methods
Data collection in XRF analysis is often facilitated by software designed for analysis. The collected data includes information about the elemental composition and concentrations. Common methods of data collection include:
- Quantitative Analysis: Provides precise measurements of element concentrations.
- Qualitative Analysis: Identifies present elements without measuring their amounts.
Both methods require proper interpretation to derive meaningful conclusions.
Accurate data collection is pivotal in determining the results of XRF analysis. Missing or erroneous data can lead to significant misunderstandings.
In summary, understanding each step in the XRF analysis procedure is essential for achieving reliable results. By paying close attention to sample selection, preparation, calibration, and analysis, researchers can harness the full potential of XRF technology.
Post-Analysis Procedures
Post-analysis procedures play a critical role in the context of XRF analysis. After the completion of the analysis, the next steps are essential for interpreting data accurately and ensuring the reliability of results. Without proper post-analysis procedures, valuable insights may remain obscured, diminishing the analysis's overall impact.
One key aspect involves data interpretation techniques. Understanding how to analyze and interpret spectra enables practitioners to draw correct conclusions about elemental composition. This process entails not only recognizing peaks in the spectrum but also understanding their relative magnitudes to estimate concentrations of various elements.
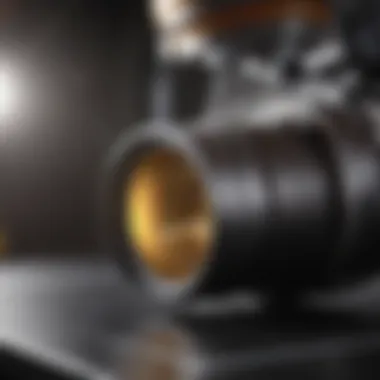
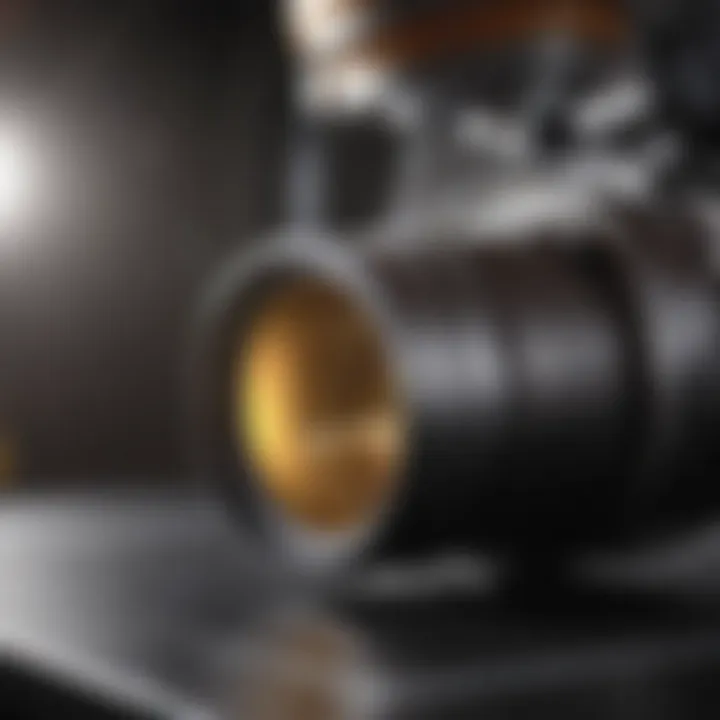
Data Interpretation Techniques
The interpretation of data generated from XRF analysis requires technical expertise. Initially, analysts must familiarize themselves with the spectral data, which reflects the intensity of emitted X-rays as a function of energy. Interpreting the peaks correctly is fundamental, as each peak corresponds to specific elements present in the sample.
To facilitate interpretation:
- Utilize software tools designed for spectral analysis. These tools can enhance accuracy and efficiency in interpreting complex spectral data.
- Compare spectral results with reference databases for elemental identification.
- Apply calibration curves to convert peak intensities into quantitative measurements of elemental concentrations.
Given the complexity of some samples, analysts may encounter overlapping peaks. In such instances, deconvolution techniques may be necessary. Special algorithms can help separate the contributions of various elements, leading to more accurate results.
Validation of Results
Validation of results is another crucial post-analysis procedure. It ensures that the findings from XRF analysis are reliable and trustworthy. Various methods can be employed for validation purposes. A common approach is to compare the obtained results with known standards or reference materials. Analyzing certified reference materials helps verify that the data falls within acceptable ranges.
Key points for validation include:
- Performing multiple analyses on the same sample to assess reproducibility.
- Cross-referencing results with other analytical techniques such as ICP-MS (Inductively Coupled Plasma Mass Spectrometry).
- Engaging in peer review or collaboration with other experts in the field to scrutinize findings.
"The reliability of any analytical technique is heavily contingent on how data is analyzed and validated; XRF is no exception."
Embedding such methodologies into practice underscores the professional rigor expected in scientific research. By adhering to these procedures, practitioners can ensure that their XRF analysis offers accurate, reproducible, and actionable insights.
Applications of XRF Analysis
X-ray fluorescence (XRF) analysis is a versatile tool used in various fields, making it essential for both research and industry. Its ability to provide accurate elemental analysis without destroying the sample offers significant benefits. Understanding the applications of XRF helps illustrate its relevance in modern science, particularly in material science, environmental monitoring, and cultural heritage preservation.
Environmental Monitoring
Environmental monitoring is one of the critical areas where XRF analysis proves invaluable. The technique enables scientists to assess soil, water, and air quality quickly and effectively. By identifying the presence of harmful elements like lead, mercury, or cadmium, XRF helps ensure compliance with environmental regulations and standards.
Key aspects of using XRF in environmental monitoring include:
- Real-Time Analysis: The ability to conduct field tests with portable XRF devices allows for immediate results, enhancing decision-making processes.
- Non-Destructive Nature: Since XRF does not alter or destroy the sample, it is ideal for sample preservation, especially in sensitive environments.
- Versatility: XRF can be applied to a variety of matrices, including solid, liquid, and gaseous samples, catering to a wide range of environmental studies.
Material Composition Analysis
In the realm of material science, XRF analysis is critical for determining the composition of materials. Many industries rely on accurate elemental analysis to ensure product quality. This has implications ranging from metallurgy to electronics.
Significant points regarding material composition analysis with XRF involve:
- Quality Control: Manufacturers use XRF to verify material specifications, ensuring that each batch meets regulatory and safety standards.
- Recycling and Waste Management: XRF analysis assists in sorting materials for recycling, helping to identify valuable metals in electronic waste, making it an effective tool for sustainable practices.
- Research and Development: In R&D settings, XRF supports the analysis of new materials, contributing to innovations in product development.
Cultural Heritage and Art Conservation
XRF analysis holds great value in the field of cultural heritage and art conservation. It allows conservators and archaeologists to study artifacts without damaging them. Understanding the elemental composition is essential for restoration and preservation efforts.
Important considerations in this domain include:
- Authentication: XRF assists in verifying the authenticity of art pieces by analyzing pigment compositions, determining whether they match the known materials used by artists in specific historical periods.
- Condition Assessment: The technique helps identify the extent of deterioration in artifacts, providing critical data for conservation strategies.
- Non-Invasive Procedure: XRFโs non-invasive nature makes it preferable for analyzing delicate artifacts, ensuring their integrity while gaining essential insights into their composition.
"XRF technology represents a bridge between science and history, allowing us to uncover stories hidden within ancient artifacts without harming them."
Advantages of XRF Techniques
X-ray fluorescence (XRF) is a powerful method for elemental analysis that offers several advantages over other analytical techniques. Understanding these benefits is crucial for researchers and professionals who seek to implement XRF in their workflows. The significance of XRF lies in its ability to provide fast, reliable, and non-destructive analysis, making it a preferred choice in various fields.
Non-Destructive Analysis
One of the most compelling features of XRF is its non-destructive nature. Unlike many analytical methods that require sample alteration or destruction, XRF enables the analysis of samples without compromising their integrity. This is particularly beneficial for precious artifacts or samples that are rare or difficult to replace. For example, archaeologists can analyze ancient artifacts without risking damage. By maintaining the original state of the sample, XRF facilitates repeated testing over time, allowing for ongoing studies without the need for new samples.
In practice, this means that multiple measurements can be taken from the same specimen, enhancing the reliability of results. Additionally, non-destructive analysis opens opportunities for further studies in multiple domains. For instance, with XRF, materials in museum collections can be analyzed for their composition, leading to better conservation strategies and methods.
Rapid Analysis Capability
Another significant advantage of XRF is its rapid analysis capability. The speed at which XRF can deliver results is unmatched compared to traditional methods. The process of collecting data can take only a few minutes, which is essential in fast-paced environments such as manufacturing or field investigations. Researchers conducting on-site analyses can obtain immediate feedback, allowing for quick decision-making and adjustments.
Furthermore, advanced XRF instruments are designed to analyze multiple elements simultaneously. This efficiency reduces the time spent on sample preparation and analysis and increases overall productivity. Consider a manufacturing scenario where several components need elemental composition verification; XRF can provide answers quickly, ensuring product quality and compliance.
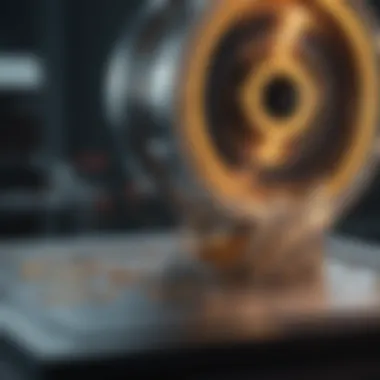
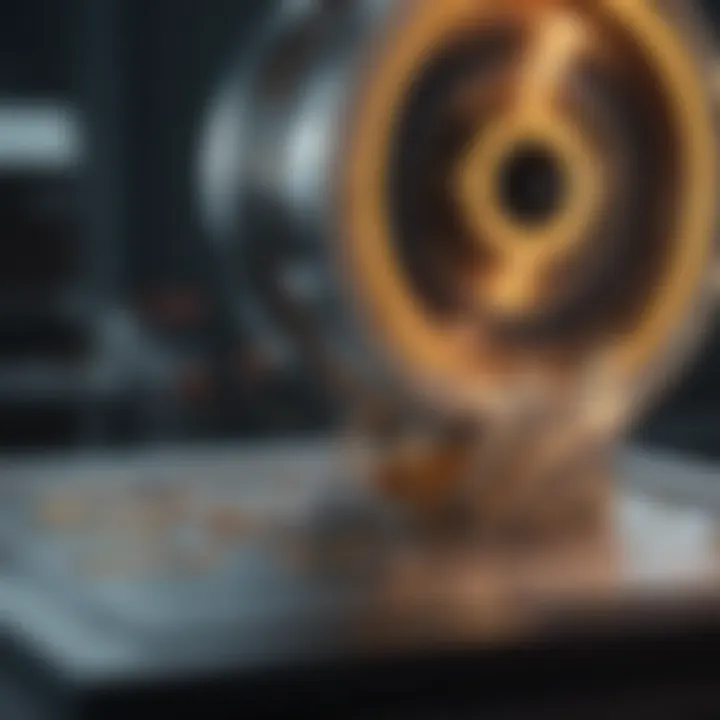
"XRF technology minimizes disruptions and maximizes efficiency, paving the way for innovative practices across various fields."
Limitations of XRF Analysis
While XRF analysis provides significant benefits in materials science, it is crucial to understand its limitations. Acknowledging these limitations can aid in tempering expectations and guiding the selection of appropriate techniques for specific applications. Furthermore, understanding the constraints helps researchers and professionals navigate potential errors in their analyses, avoiding pitfalls and ensuring accurate results. This section will explore key limitations encountered in XRF analysis, divided into two primary aspects: detection limits and sensitivity issues, and matrix effects in sample analysis.
Detection Limits and Sensitivity Issues
XRF analysis excels at providing qualitative and quantitative information about elemental compositions, yet it does come with inherent sensitivity limits. The detection limit refers to the smallest concentration of an element that can be reliably measured. In general, XRF struggles to detect lighter elements, especially those with atomic numbers lower than that of magnesium. Elements such as boron, lithium, and beryllium may elude detection entirely, resulting in incomplete data.
Sensitivity issues can arise from several factors:
- Sample Thickness: Thick samples can absorb incident X-rays, leading to decreased fluorescence signals from deeper layers.
- Elemental Concentration: Low concentrations lead to weak signals, increasing the uncertainty of measurements. The signal-to-noise ratio diminishes, affecting result reliability.
- Interference Effects: Overlapping peaks from different elements can complicate spectral analysis, further lowering sensitivity to the elements of interest.
Overall, the limitations in detection and sensitivity necessitate a careful approach to method selection, particularly for applications requiring precise elemental analysis at low concentrations.
Matrix Effects in Sample Analysis
Matrix effects refer to the influence of the sample's composition on the accuracy and precision of the XRF results. This variability can arise from several interactions that compromise data quality:
- Absorption and Scattering: The physical matrix can absorb or scatter X-rays, leading to altered intensity and skewed results. Variability in sample density and texture can cause significant discrepancies in excitation conditions.
- Chemical Composition: The presence of certain elements can enhance or suppress the signals of others. Competing interactions, such as X-ray reabsorption by heavier elements, complicate analyses.
- Homogeneity of Samples: Non-homogeneous samples can produce inconsistent results. The uneven distribution of elements may lead to sampling errors, affecting accuracy.
Thus, matrix effects are significant considerations in any XRF analysis. Thorough preliminary studies are necessary to understand how sample composition interplays with the analysis, ensuring that results are reliable and valid across different contexts.
Understanding these limitations is as critical as mastering the techniques of XRF analysis, as they dictate the reliability and applicability of obtained data.
Future Directions in XRF Analysis
The future of X-ray fluorescence (XRF) analysis holds immense potential as technological advancements continue to enhance its capabilities. This section aims to explore the pivotal trends and developments that are shaping the trajectory of XRF methodologies, with a focus on emerging technologies and the integration of XRF with other analytical techniques. It is important to recognize not only the benefits of these advancements but also the considerations that come with them.
Emerging Technologies and Innovations
Emerging technologies play a critical role in the evolution of XRF analysis. As industries demand higher accuracy and efficiency, innovations are being integrated into XRF systems.
One significant trend includes the enhancement of detector technologies. For instance, Silicon Drift Detectors (SDDs) provide improved resolution and sensitivity, enabling researchers to detect lower concentrations of elements in various samples. Additionally, advancements in micro-XRF systems allow for high spatial resolution mapping of materials. This opens up new avenues for detailed examinations in fields such as art conservation and materials science.
Another promising area is the development of portable XRF instruments. These portable devices facilitate on-site analysis, greatly increasing ease of use in field applications. This mobility allows for immediate data collection across various environments, such as in metal recycling or environmental monitoring.
Moreover, software innovations contribute significantly to the efficiency of data processing and interpretation. Advanced algorithms and machine learning techniques are becoming increasingly common. These developments streamline quantitative analysis and enhance accuracy in elemental quantification.
Integration with Other Analytical Techniques
Integration of XRF with other analytical techniques represents a frontier with substantial promise. Combining XRF with techniques like scanning electron microscopy (SEM) or inductively coupled plasma mass spectrometry (ICP-MS) offers complementary benefits.
By employing XRF alongside SEM, researchers can achieve elemental analysis while simultaneously obtaining morphological insights. This dual analysis is particularly valuable in materials research, where understanding both composition and structure is crucial.
On the other hand, ICP-MS excels in achieving low detection limits for trace elements compared to XRF. When used together, these techniques can produce a more holistic understanding of the samples under investigation. This synergy leads to more robust conclusions in research and quality control contexts.
In exploring the future directions of XRF, one must also consider the challenges that come with integrating these technologies. Standards and protocols must be developed to ensure consistent and comparable results. Furthermore, researchers must address the complexities introduced by specimen preparation and sample matrix effects.
The integration of XRF with other techniques will enhance its analytical capabilities, enabling researchers to obtain a comprehensive view of materials that was previously unattainable.
Overall, as XRF continues to evolve, the combination of these emerging technologies and integrative approaches will likely redefine its position in both academic and industrial settings. Researchers and professionals working in this domain need to stay updated on these developments to exploit the full potential of XRF analysis.
These innovations collectively demonstrate that the future of XRF analysis is not just about improving existing methodologies, but also about expanding its application and utility in diverse disciplines. By keeping pace with these advancements, the scientific community can enhance the accuracy, efficiency, and relevance of elemental analysis.
Ending
The conclusion section of this article provides a pivotal summary of the extensive content explored throughout the narrative. It synthesizes the critical components of X-ray fluorescence (XRF) analysis, emphasizing its role in materials science and related fields. Understanding the overall importance of XRF is essential for students, researchers, educators, and professionals who seek to incorporate this technique into their work.
Summary of Key Points
XRF analysis plays a vital role in elemental analysis due to several significant factors:
- Accessibility: It allows for quick and non-destructive testing of materials, making it valuable in various applications.
- Versatility: XRF can be used in environmental monitoring, cultural heritage preservation, and material composition analysis among others.
- Data Reliability: The validation processes outlined earlier ensure that results produced from XRF are credible and accurate.
These key points illustrate how XRF is not just a method, but an essential tool in modern scientific inquiry. A comprehensive grasp of XRF procedures ensures that users can implement it effectively in their research or professional practice.
Implications for Future Research
The evolution of XRF analysis continues to open avenues for innovative research and applications. Future investigations may focus on:
- Technological Advancements: The integration of emerging technologies, such as machine learning algorithms, could enhance the precision of XRF data analysis.
- Multi-Technique Approaches: Combining XRF with other analytical methods may lead to more comprehensive assessments and accurate results.
- Expanded Applications: Exploring new areas, such as nanomaterials or complex composites, can significantly benefit from XRF's capabilities.