Understanding RNA Secondary Structure: Significance and Implications
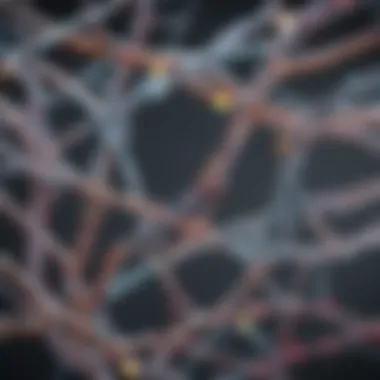
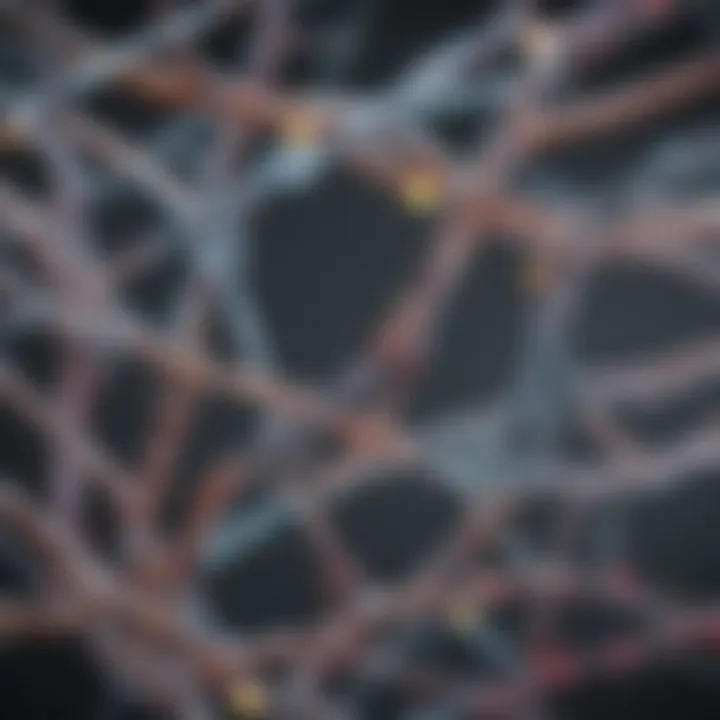
Intro
The field of molecular biology is vast, but one area of particular intrigue is RNA secondary structure. This structure is not merely a byproduct of genetic coding; it plays a critical role in determining RNA's functional capabilities. Understanding this aspect can unlock insights into various biological processes, such as gene regulation and protein synthesis. This article aims to explore these elements in depth, making a compelling case for the significance of RNA secondary structure.
Research Overview
Summary of Key Findings
RNA secondary structure can be defined as the spatial arrangement of nucleotides that influences the molecule's function. Elements such as stems, loops, and bulges form the basic building blocks of this structure. Recent studies emphasize that these structures contribute not just to stability, but also to the versatility of RNA.
Research highlights indicate that mutations affecting secondary structure may lead to significant biological consequences. Understanding these interactions can pave the way for new therapeutic approaches for RNA-related diseases.
Relevance to Current Scientific Discussions
In contemporary scientific discourse, RNA secondary structure has gained attention beyond traditional roles. It is increasingly recognized for its implications in fields like gene therapy and synthetic biology. As we delve deeper into RNAβs multifaceted roles, the significance of its secondary structure becomes undeniable.
Methodology
Research Design and Approach
The understanding of RNA secondary structure relies heavily on both computational and experimental methodologies. The computational tools used for structure prediction include software such as RNAfold and mfold, which offer detailed insights into structural conformations based on sequence data. These models can approximate how RNA will behave under different conditions.
Data Collection and Analysis Techniques
Experimental techniques to validate computational models often include techniques like X-ray crystallography and nuclear magnetic resonance (NMR) spectroscopy. These methods allow researchers to observe RNA structures in real-time, providing empirical data that supports or refutes computational predictions.
Through a balanced approach combining both computational and experimental methods, the scientific community can form a more nuanced understanding of RNA secondary structure.
Defining RNA Secondary Structure
RNA secondary structure refers to the spatial arrangement of nucleotides within an RNA molecule that is stabilized by hydrogen bonds and other non-covalent interactions. This arrangement is essential as it influences the RNA's function and interactions within the cell. Understanding RNA secondary structure is a fundamental part of molecular biology since it aids in deciphering how RNA molecules contribute to various biological processes.
Components of RNA Secondary Structure
Stems
Stems are formed through the base-pairing of complementary nucleotides and play a crucial role in stabilizing RNA structures. The unique aspect of stems lies in their ability to create double helical regions, which are integral for the overall structural integrity of the RNA. They provide a framework that ensures the molecule can maintain its functional conformation.
A key characteristic of stems is their rigidity compared to loops and bulges. This rigidity makes them a beneficial component of RNA secondary structure as they directly contribute to the stability of RNA under physiological conditions. The formation of stems is a popular feature seen in RNA folding and is essential for the proper functioning in many biological mechanisms.
Unique to stems is their ability to form Watson-Crick base pairs, which align perfectly with each other, enhancing stability. However, a disadvantage can be that rigidity limits flexibility which some RNA functions may require.
Loops
Loops are unpaired regions of RNA that connect stem regions. They represent variability in RNA structure. Loops are important for the flexibility they introduce into the RNA molecule, allowing it to interact with other molecules effectively. A significant aspect of loops is their role in mediating protein-RNA interactions, which are vital for many cellular functions.
The unique feature of loops is their ability to adopt various conformations, which can enhance the specificity of RNA interactions. This characteristic is very beneficial for processes such as splicing and ribosome assembly. One downside is that loops can sometimes introduce instability if not properly folded.
Bulges
Bulges are structural elements where one or more unpaired nucleotides protrude from a stem region. They provide critical insights into RNA folding and stability. Bulges can affect how RNA folds by disrupting the symmetry of stems, allowing for unique interactions with proteins and other RNA molecules.
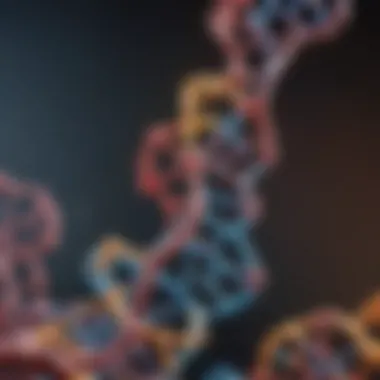
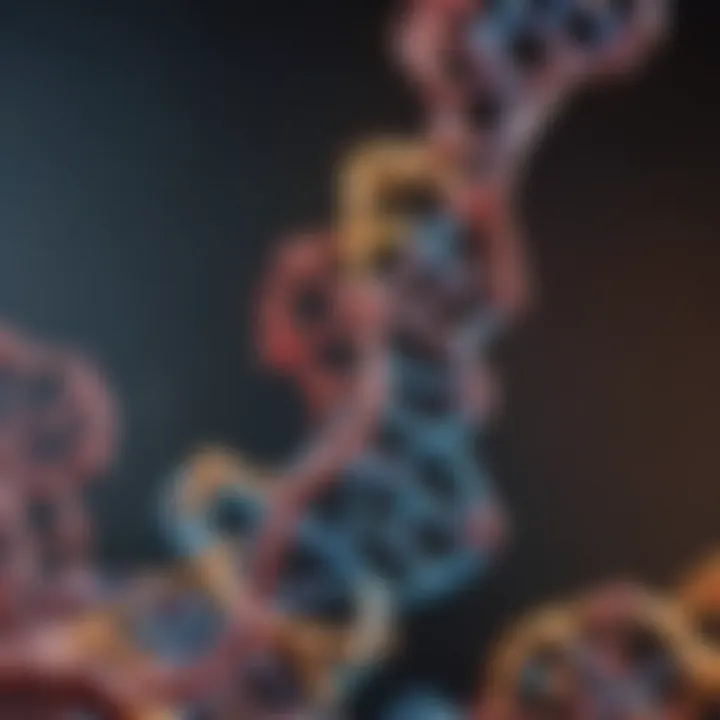
A defining characteristic of bulges is their disruptive nature, which can promote diverse structural conformations. This trait is beneficial as it allows for a range of functions; however, it can also lead to instability if not adequately positioned within the secondary structure.
Importance of RNA Structure
Despite the simplicity of RNA as a genetic material, its secondary structure is intricately tied to various functional roles within the cell. Understanding these roles is integral to grasping the complexities of molecular biology.
Functional Roles
The functional roles of RNA structures are extensive. They are involved in a vast range of cellular processes such as the regulation of gene expression and the facilitation of protein synthesis. Each structural element of RNA can have implications for how effectively these functions occur. The ability of RNA to fold into different structures allows it to take on roles that would not be possible otherwise. For example, specific loops may influence the assembly of ribosomes, demonstrating how diverse functional roles can emerge from RNA structure.
A unique feature of functional roles in RNA is that it can exhibit a degree of redundancy. This redundancy means that multiple structures or conformations can perform similar roles, which enhances the adaptability of RNA in cellular environments.
Structural Diversity
The structural diversity found in RNA secondary structures is another critical aspect that should not be overlooked. Different RNA sequences can fold into varied structures which dictate their functionality. This diversity allows RNA molecules to participate in an array of tasks such as catalyzing biochemical reactions, acting as signaling molecules, and maintaining genomic integrity.
The variety of structures is beneficial as it permits RNA to evolve new functions, granting it the ability to adapt to the specific needs of the cell. However, the complexity of this diversity can be a disadvantage when attempting to predict structure from sequence, a challenge faced by many researchers in the field.
Overall, the intricate relationship between RNA secondary structure and its functional implications underscores the need for continued research and understanding in this area. By exploring these components, we can gain insights into the fundamental workings of cellular processes.
Methodologies for Analyzing RNA Structure
Understanding the methodologies for analyzing RNA structure is crucial for comprehending its functional roles in biological systems. This section highlights the computational tools and experimental techniques that are essential in elucidating the complexities of RNA secondary structure. The information provided here lays the groundwork for the subsequent discussions on how these methodologies contribute to our understanding of RNA's pivotal role in cellular processes.
Computational Tools for Structure Prediction
RNAfold
RNAfold is a widely used software for predicting the secondary structure of RNA sequences. Its principal feature is the ability to calculate the minimum free energy (MFE) structure, which indicates the most stable conformation of an RNA molecule. RNAfold applies dynamic programming algorithms to efficiently predict secondary structures while taking into account base pairings and folding constraints.
One of the main advantages of RNAfold is its user-friendly interface that allows researchers to quickly input sequences and obtain results. This ease of use makes it a popular choice among researchers, particularly those who may not have extensive programming experience. However, one limitation to consider is that while RNAfold provides a solid prediction for secondary structure, it may not always accurately elucidate long-range interactions or complex structures thus needing additional analysis.
Mfold
Mfold is another prominent tool for RNA structure prediction, renowned for its comprehensive approach to modeling RNA. It considers not just the sequence but also various environmental factors, such as temperature, which could affect folding. Mfold allows users to explore a range of possible structures and provides options for analyzing multiple sequences, making it a flexible choice for researchers.
The unique feature of Mfold is its graphical interface that visually represents predicted RNA structures, which aids in the interpretation and understanding of the results. While Mfold delivers high-quality predictions, some users report that it requires a steep learning curve for newcomers due to its extensive options and parameters. Nonetheless, both RNAfold and Mfold are invaluable in the preliminary analysis of RNA secondary structures.
Experimental Techniques
Experimental validation is key to confirming the predictions made by computational tools. Here, we explore several experimental techniques that are essential for analyzing RNA structure and function.
X-ray Crystallography
X-ray Crystallography is a cornerstone technique for determining the three-dimensional structures of biomolecules, including RNA. By crystallizing an RNA molecule and then analyzing the diffraction pattern produced when X-rays bombard the crystal, researchers can derive detailed structural information.
The critical advantage of X-ray Crystallography is its ability to deliver high-resolution structures, making it possible to observe subtle changes in RNA conformations. However, its primary drawback is the difficulty associated with obtaining suitable RNA crystals, which can be a limiting factor in structural studies.
Nuclear Magnetic Resonance (NMR)
Nuclear Magnetic Resonance (NMR) spectroscopy provides another means of analyzing RNA structures in solution. NMR is particularly useful for studying dynamic processes and can provide information on structural flexibility that crystallography may miss.
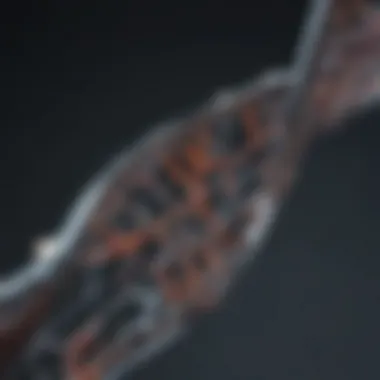
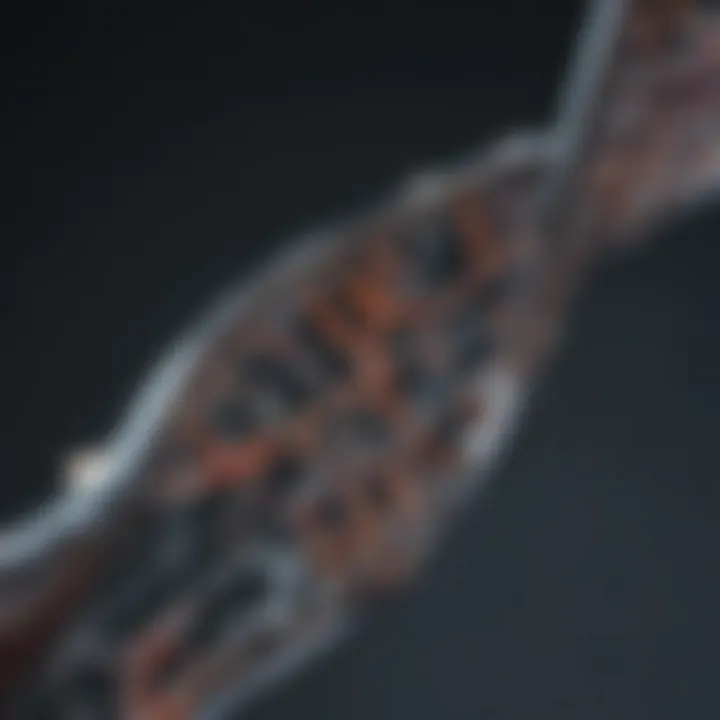
A key characteristic of NMR spectroscopy is its ability to illuminate how RNA behaves in an environment that closely mimics physiological conditions. This is crucial for understanding functional mechanisms. A disadvantage is that NMR is often limited to smaller RNA molecules due to sensitivity and signal-to-noise issues, which can pose challenges in studying larger RNA complexes.
Cryo-Electron Microscopy
Cryo-Electron Microscopy (Cryo-EM) has emerged as a profound technique for investigating large RNA-protein complexes. This technique involves flash-freezing samples to preserve their native state and then imaging them using electron microscopy.
A significant feature of Cryo-EM is its ability to visualize complex, heterogeneous samples without the requirement for crystallization. This allows researchers to analyze structures that may be difficult to crystallize. However, one limitation includes the relatively lower resolution compared to X-ray crystallography, making precise structural determinations more challenging.
Each of these methodologies offers distinct advantages and disadvantages, underscoring the importance of a multi-faceted approach in analyzing RNA secondary structures. By integrating computational predictions with experimental data, researchers can achieve a comprehensive understanding of RNA function in biological contexts.
Structural Elements and Their Functions
Understanding the structural elements of RNA is crucial for grasping its functionality and the vast implications it holds in molecular biology. The key structural components are stems, loops, and bulges. Each of these elements plays a distinctive role in the stability and function of RNA molecules. Research has shown that these structures contribute significantly to the interactions RNA has within a biological system.
Stems: Formation and Stability
Stems are one of the primary structural elements of RNA. They form when complementary regions of RNA base pair with each other, creating a double-stranded structure. This base pairing is pivotal for maintaining the stability of RNA. A well-formed stem can provide a robust structure that withstands various cellular conditions.
The stability of stems is influenced by factors such as the sequence of nucleotides involved, temperature, and ionic conditions. Stems can vary in length, and the length can directly affect the overall stability of the RNA molecule. Research has indicated that stabilized stems are often important in determining the specific folding pathways of RNA.
"The formation of stems is essential for the three-dimensional conformation of RNA, influencing its functional interactions within the cell."
Loops: Variability and Interaction
Loops are non-paired regions in RNA that occur at the ends of stems or between them. These areas are often more flexible than stems, allowing for variability. The variability in loops can facilitate important interactions with proteins and other molecules, which is vital for functions such as regulation and catalytic activities.
Loops can differ greatly in size and sequence, which contributes to their ability to engage in diverse interactions. The unique structural properties of loops enable RNA to adapt and perform a myriad of functions in biological processes. Understanding the variability of loop structures can also shed light on mechanisms facilitating gene expression and regulation.
Bulges: Implications for Folding
Bulges are another significant structural element within RNA. They occur when there are unpaired nucleotides present on one strand of a stem. This unpaired region can dramatically affect the overall topology and stability of the RNA molecule. Bulges can introduce bends and irregularities in the RNA structure, which can be essential for recognition by other biomolecules.
The presence of bulges is often linked to specific functions of RNA, including its ability to act as a catalyst or scaffold during various reactions. These structures can influence the folding dynamics of RNA and affect how it interacts with other biological macromolecules, such as proteins. Understanding bulges can lead to insights into how RNA achieves its diverse range of functionalities.
Relationship Between RNA Structure and Function
RNA structure is an essential aspect of its functionality in various biological processes. The shape and conformation of RNA molecules are directly linked to their roles in gene expression, regulation, and cellular activity. The secondary structure of RNA, formed by intramolecular base pairing, allows it to adopt specific shapes that are critical for its function. This relationship suggests that understanding RNA's structure can unlock insights into its complex roles in biology.
One significant area where RNA structure plays a crucial role is gene regulation. RNA molecules can interact with proteins and other nucleic acids, which is directly influenced by their shape. For instance, some regulatory RNAs, including microRNAs and long non-coding RNAs, can bind to messenger RNA (mRNA) and modulate its translation process. This binding is often dictated by the secondary structure of the RNA, showcasing how structure informs function.
In the realm of protein synthesis, RNA structures also hold substantial importance. Ribosomal RNA is a central player in the translation process. Its structural features are not just supportive; they are essential for the correct assembly and function of ribosomes. The mRNA's secondary structure can also influence the efficiency of translation by affecting how ribosomes interact with the mRNA molecule.
Understanding the relationship between RNA structure and function has profound implications. It enables researchers to predict how changes in RNA sequences might affect biological outcomes. This insight can be particularly useful in developing RNA-targeted therapies for various diseases. The interactions within this structure-function paradigm are not only fascinating but are also a cornerstone in biochemistry and molecular biology.
Understanding RNA structure is key for unlocking its biological roles, especially in regulation and synthesis.
In summary, the connection between RNA structure and function establishes a foundation for further exploration of RNA molecules in both health and disease. A thorough investigation of this relationship opens avenues for innovative research and potential therapeutic strategies.
RNA Secondary Structure in Disease Mechanisms
The understanding of RNA secondary structure plays a significant role in elucidating various disease mechanisms. This section aims to delve into how RNA structure is intertwined with pathogenesis, especially in viral infections and genetic disorders. The unique spatial arrangements of RNA facilitate critical cellular processes, and abnormalities in these structures can lead to detrimental effects on health. The implications are far-reaching, with potential for therapeutic interventions.
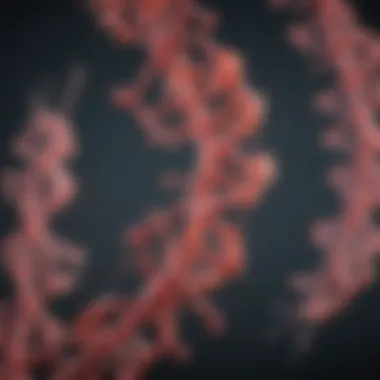
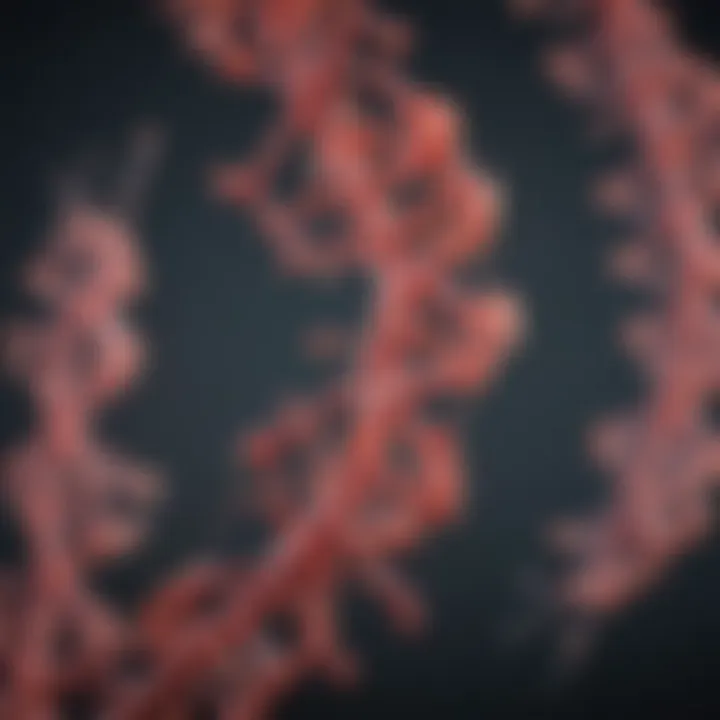
Implications in Viral Infections
Viruses often hijack the hostβs cellular machinery, and their RNA plays a pivotal role in this process. Adhering to their own functional requirements, viral RNAs exhibit diverse secondary structures that can influence virulence and replication. For instance, in viruses like HIV and influenza, specific RNA structures are crucial for interactions with host proteins, aiding in the evasion of immune responses.
- Viral RNA structures can serve as targets for antiviral therapies.
- Structural motifs present in the viral genome can be recognized by host defenses, such as pattern recognition receptors. Their modification can result in escaping detection.
- Understanding these structures provides insight into how viruses can mutate and adapt, posing challenges for treatment.
A pertinent example lies with the Zika virus, where studying the RNA secondary structure has led to revelations about its replication and interactions with the host's cellular proteins. This knowledge enhances our ability to develop targeted therapies. Thus, recognizing the relevance of RNA structure in viral infections is crucial for advancing antiviral drug design.
Role in Genetic Disorders
In the context of genetic disorders, RNA secondary structure can also significantly impact gene expression and stability. Mutations that alter RNA folding may lead to the production of malfunctioning proteins, giving rise to various conditions. There are numerous instances where specific RNA structural changes correlate with genetic pathologies.
- Conditions such as myotonic dystrophy and cystic fibrosis highlight how aberrant RNA secondary structures can affect protein synthesis and function.
- Misfolded RNAs can undergo accelerated degradation, leading to insufficient protein levels needed for normal cellular function.
- In some disorders, expanded repeat sequences within RNA lead to toxic gain-of-function phenotypes due to altered secondary structures.
The recognition that RNA structure is a key player in genetic diseases enhances our understanding of the mechanisms behind these conditions. Genetic and therapeutic strategies are being developed to target these faulty structures, aiming to restore normal function.
Understanding the role of RNA secondary structure in disease mechanisms provides a foundation for therapeutic developments. By targeting these crucial elements, we can potentially manage the expression and activity of genes involved in disease pathology.
In summary, RNA secondary structures are not merely functional components but are essential in understanding disease mechanisms. The implications in viral infections and genetic disorders highlight the necessity for continued research in this field. Identifying and manipulating these structures pave the way for innovative treatments.
Advancements in RNA Research
Research in RNA secondary structure has seen substantial growth in recent years. This advancement is crucial, as RNA's ability to perform various biological functions is influenced heavily by its secondary structure. The development of novel methodologies provides fresh insights into RNA biology, allowing scientists to explore RNA's versatility as a biomolecule. These findings have implications that extend beyond fundamental biology, influencing areas such as medicine, agriculture, and biotechnology.
Emerging Technologies
CRISPR
CRISPR technology has risen as a significant tool in molecular biology and genetics. This technique offers a precise way to edit genes, which can be particularly useful when investigating RNA functions. One key characteristic of CRISPR is its ability to target specific RNA sequences for modification or interference. This capability positions it as a beneficial asset in research aiming to dissect RNA functionalities and interactions.
A unique aspect of CRISPR is the use of guide RNA, which directs the Cas9 protein to the desired RNA target. This feature allows researchers to manipulate RNA secondary structures with high specificity. The advantages of CRISPR include its cost-effectiveness and the efficiency with which it can be utilized in various experimental settings. However, it also has potential disadvantages, such as the off-target effects that could arise from unintended modifications.
RNA Therapeutics
RNA therapeutics represent a burgeoning field that focuses on using RNA molecules to treat diseases. These treatments can be designed to modulate gene expression or target specific pathways disrupted in diseases, making them incredibly valuable in medical research and application. A defining characteristic of RNA therapeutics is their adaptability, enabling them to be tailored to the specific needs of patients or to address various targets.
One unique feature of RNA therapeutics is the ability to utilize synthetic RNA molecules. These can be engineered to enhance stability and delivery to target cells. Advantages of RNA therapeutics include their potential for rapid development and application, particularly in response to emerging health threats. Nonetheless, challenges such as delivery mechanisms and ensuring the stability of RNA in biological systems remain hurdles that researchers continue to navigate.
Future Directions in Research
As the understanding of RNA secondary structure deepens, researchers are exploring innovative avenues to leverage RNAβs capabilities in scientific and medical realms. Future research may focus on integrating advanced computational tools and experimental techniques to unveil previously unknown functions of RNA structures. The interplay between RNA structure and function will be a vital area for discovering new therapeutic targets.
Additionally, the expansion of RNA-based technologies could lead to novel applications in gene therapies and precision medicine, further demonstrating the importance of ongoing RNA research. Addressing the current limitations and enhancing the methodologies used for RNA analysis will play a crucial role in shaping the future landscape of biotechnology and molecular biology.
Concluding Insights
As we examine the landscape of RNA secondary structure, it becomes evident that understanding this aspect is indispensable for unraveling diverse biological processes. The insights drawn from the previous sections emphasize the intricate relationship between RNA structure and its functional repertoire. By analyzing the organization of RNA into stems, loops, and bulges, we gain fundamental knowledge about how these shapes facilitate interactions at the molecular level. The biochemical versatility that RNA exhibits is a direct consequence of its structural dynamics. Thus, diving deeper into these elements not only elucidates intrinsic cellular mechanisms but also enhances our grasp of broader biological implications.
The Role of RNA Secondary Structure in Biology
The core role of RNA secondary structure in biology cannot be overstated. It shapes the interactions RNA has with various proteins, molecules, and itself, ultimately influencing gene expression. In the realm of gene regulation, these structural motifs serve as critical determinants for the binding of regulatory proteins, impacting transcriptional and translational control.
Furthermore, RNA secondary structures function as active participants in protein synthesis. Ribosomes, the molecular machines of translation, rely intricately on RNA's folding to ensure the precise construction of proteins, essential for cellular function. These structures do not merely provide a scaffold; they are essential for the catalytic actions performed during RNA processing and protein synthesis.
In addition, emerging studies indicate that the variations in RNA secondary structures can influence disease mechanisms. For example, the misfolding of RNA has been associated with several genetic disorders and viral pathogenesis. This highlights the necessity of understanding RNA structure comprehensively, as it may offer clues to therapeutic approaches.
"Understanding RNA's secondary structure is crucial for both basic science and applied health research."
In summary, the exploration of RNA secondary structure opens a multitude of avenues for research and application. Its role extends from fundamental biology to potential therapeutic targets, underscoring the interconnectedness of molecular structures and biological functions. As we move forward, the emphasis on RNA structure within molecular biology will likely intensify, guiding future studies aiming to unlock its full potential.