Understanding PCR: Key to Cancer Diagnosis and Research
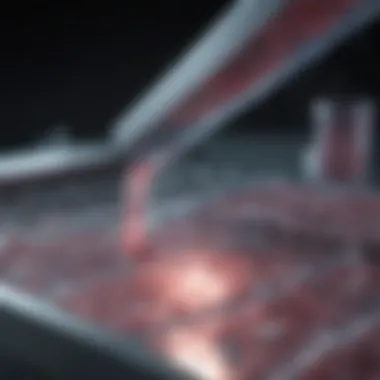
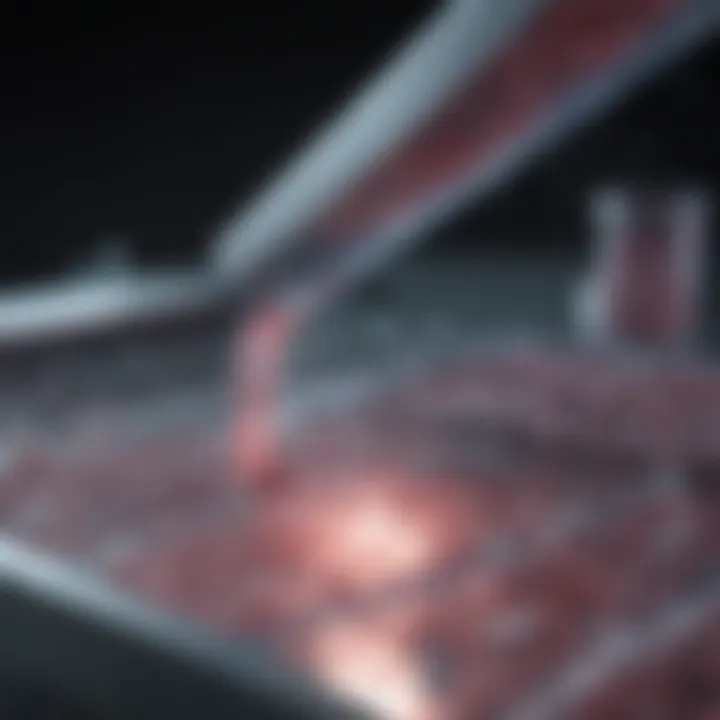
Research Overview
Polymerase Chain Reaction (PCR) has transformed the landscape of cancer diagnosis and research. Its ability to amplify specific DNA sequences makes it an invaluable tool in molecular biology. The technology allows for the detection of genetic mutations associated with various cancers, providing crucial insights for both diagnosis and treatment. As we delve into this article, we will explore significant findings and the relevance of PCR technology in current scientific discussions.
Summary of Key Findings
Recent advancements in PCR methodologies have yielded notable findings:
- Early Detection: PCR can identify cancer biomarkers at very low concentrations, aiding in earlier diagnosis compared to traditional methods.
- Personalized Medicine: The ability to examine specific genetic mutations allows for tailored treatment plans that target the unique characteristics of an individual’s cancer.
- Research Applications: PCR is not only vital in clinical settings but also in understanding the molecular pathways of cancer, which helps in developing new therapeutic strategies.
"The groundbreaking ability of PCR to detect minute quantities of DNA will change the approach to early cancer diagnosis and personalized treatment strategies."
Relevance to Current Scientific Discussions
The relevance of PCR in oncology cannot be overstated. Current scientific discussions often focus on integrating advanced PCR techniques with next-generation sequencing. This integration enhances the understanding of the genetic landscape of tumors, facilitating more effective therapeutic interventions.
Many researchers are investigating the potential of PCR in liquid biopsies, which allow for non-invasive cancer detection through blood samples. This development indicates a significant shift towards more patient-friendly diagnostic methods.
Methodology
Understanding how PCR is applied in cancer diagnosis and research involves examining the methodologies used in studies.
Research Design and Approach
Research often employs a mixed-methods approach, combining quantitative and qualitative analyses. Typically, studies might involve:
- Clinical Trials: Testing the efficacy of PCR-based diagnostics in real-world settings.
- Laboratory Studies: Focusing on optimizing PCR techniques and evaluating their sensitivity and specificity.
Data Collection and Analysis Techniques
Data collection revolves around:
- Sample Collection: Tissue samples, blood, or other bodily fluids are collected from patients suspected to have cancer.
- PCR Techniques: Standard PCR, quantitative PCR (qPCR), and digital PCR are employed to analyze the samples, followed by data analysis to assess amplification results.
Researchers often publish their findings in peer-reviewed journals, contributing to the ongoing development of PCR applications in oncology. These methodologies ensure that new techniques are reliable and reproducible, advancing scientific knowledge in cancer research.
Prelude to PCR Technology
Polymerase Chain Reaction (PCR) is a cornerstone technology in molecular biology, particularly relevant in cancer diagnosis and research. The significance of PCR extends beyond mere scientific interest; it serves as a pivotal tool that revolutionizes our understanding of genetic material linked to cancer. This section will explore the definition, historical background, and the foundational aspects of PCR that have made it an indispensable method in oncology.
Definition of PCR
Polymerase Chain Reaction is a technique used to amplify specific segments of DNA, enabling researchers to create millions of copies of a particular DNA sequence. This process enhances the ability to analyze genetic material in detail. The amplification occurs in a series of cycles that involve temperature changes, which is essential for separating the DNA strands, annealing primers, and extending new DNA strands. This allows for even minute amounts of DNA to be evaluated with precision, playing a crucial role in diagnostics, research, and therapeutic development.
Historical Background
The development of PCR can be traced back to the late 1980s, primarily attributed to Kary Mullis, who conceptualized the method while working for Cetus Corporation. Initially, the idea faced skepticism, yet Mullis's innovative approach gained traction. In 1985, the first paper detailing PCR was published, marking a turning point in genetic research.
Over the years, the technology has been refined, with significant improvements to its efficiency and accuracy. The first commercial PCR machines became available in the early 1990s, paving the way for widespread adoption in laboratories around the world. Today, PCR stands as a foundation not only for cancer research but also in genetics, forensics, and even virology, showcasing its essential role in advancing scientific inquiry and healthcare.
Mechanisms of Polymerase Chain Reaction
The Polymerase Chain Reaction (PCR) serves as a critical process in cancer research and diagnosis. Understanding its mechanisms lays a foundation for comprehending how PCR aids in the early detection and treatment of cancers. The precise orchestration of components and steps involved in PCR allows researchers and clinicians to amplify specific DNA sequences, thereby enabling detailed genomic analysis. In oncology, these mechanisms can discern cancer-related mutations or the presence of specific oncogenes, which are vital for targeting therapies.
Components of PCR
The success of the PCR technique depends on several key components:
- DNA Template: This is the segment of DNA that will be copied. In cancer testing, it may come from tumor cells or blood samples.
- Primers: Short DNA sequences that provide a starting point for DNA synthesis. They bind to the template DNA at specific locations.
- DNA Polymerase: An enzyme responsible for synthesizing new DNA strands. Taq polymerase, for example, is often used in PCR due to its heat resistance.
- Nucleotides: The building blocks of DNA, consisting of adenine, thymine, cytosine, and guanine. A balanced mix of these is necessary for creating new DNA strands.
- Buffer Solutions: These maintain optimal pH and ionic conditions for the reaction to proceed effectively.
These components work together to produce a significant amount of DNA, which can then be analyzed for cancerous changes.
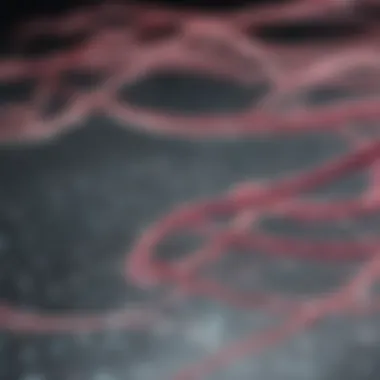
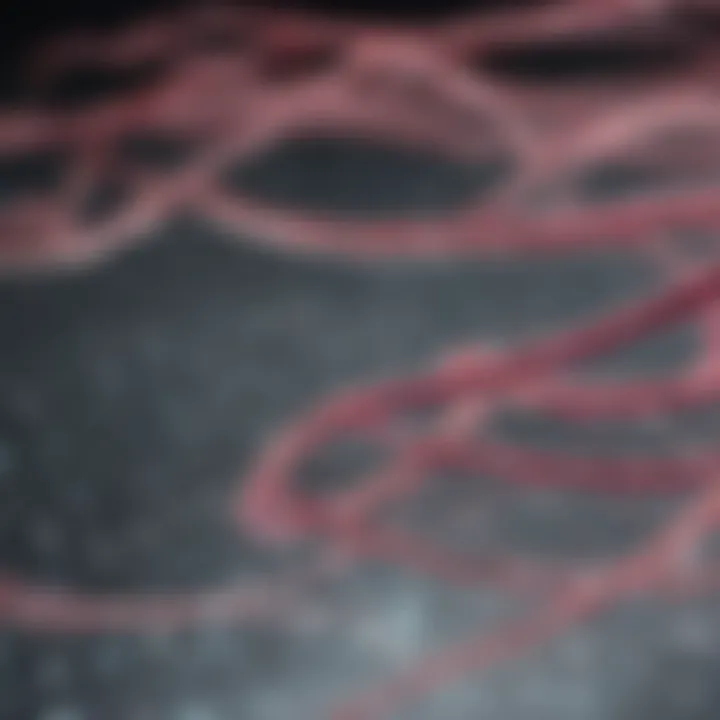
Steps in PCR Process
The PCR process unfolds in three main steps:
- Denaturation: The double-stranded DNA is heated to around 94-98°C, causing it to separate into two strands.
- Annealing: The temperature is lowered to 50-65°C, allowing the primers to bind to their complementary sequences on the template strands.
- Extension: The temperature is raised to approximately 72°C, which is optimal for the DNA polymerase to synthesize new DNA strands by adding nucleotides.
These steps are repeated for 20 to 40 cycles, resulting in the exponential amplification of the target DNA segment. This cycle yields millions of copies in a matter of hours, making it a powerful tool for cancer diagnostics.
Types of PCR Techniques
The variations of PCR techniques enhance its utility in oncology:
Quantitative PCR
Quantitative PCR, or qPCR, measures the amount of DNA produced in real-time during the amplification process. This technique allows researchers to quantify gene expression levels or detect minimal residual disease, essential in tracking cancer progression. A key characteristic of qPCR is its ability to deliver results in a shorter time compared to traditional methods. Its unique feature lies in the use of fluorescent dyes, which provide a visual representation of PCR progress. However, it may require careful optimization to avoid amplification of non-target sequences.
Reverse Transcription PCR
Reverse Transcription PCR (RT-PCR) is critical for analyzing gene expression by converting RNA into DNA before amplification. This approach allows for the examination of specific RNA molecules, which can lead to insights into cancer biomarkers. RT-PCR stands out due to its ability to assess the functionality of genes, making it a valuable tool in cancer research. However, it can be more complex than other methods, particularly in the steps of RNA extraction and cDNA synthesis.
Nested PCR
Nested PCR improves specificity by using two sets of primers in two sequential reactions. The first round amplifies a larger segment, while the second focuses on a specific region. This technique is particularly useful when the starting DNA is limited or degraded, which is often the case in clinical samples. The key characteristic of Nested PCR is its heightened sensitivity and specificity. Yet, it may increase the risk of contamination, requiring extra diligence in handling samples.
PCR Applications in Cancer Diagnosis
Polymerase Chain Reaction (PCR) is a revolutionary technique that fundamentally changed the landscape of cancer diagnostics. The ability to amplify specific DNA sequences allows for the detection of cancer at very early stages. PCR's applications in cancer diagnosis offer not only enhanced reliability but also a significant potential for personalized treatment strategies, making it an invaluable tool in the oncological field.
Early Detection of Cancer
Early detection of cancer is critical for improving patient outcomes. PCR enables the identification of genetic alterations associated with cancer, even when tumors are still small and asymptomatic. By amplifying specific regions of DNA, PCR helps in detecting mutations that can indicate the presence of malignancies. This permits healthcare professionals to initiate treatment sooner, which is often correlated with higher survival rates.
The process begins with the collection of samples, which may include blood, tissue, or other bodily fluids. Next, using targeted primers, the PCR selectively amplifies sequences of interest. For instance, detecting mutations in genes such as KRAS or TP53 can raise suspicion of cancer development. The high sensitivity of PCR means it can often identify trace levels of these genetic markers, providing insights that traditional imaging or biopsy methods may miss.
Tumor Marker Identification
Another significant application of PCR is in the identification of tumor markers. Tumor markers are substances produced by cancer cells or by normal cells in response to cancer. Examples include Carcinoembryonic antigen (CEA) and Alpha-fetoprotein (AFP). PCR can be employed to quantify these markers in patient samples, offering insights into cancer presence and progression.
The process of utilizing PCR for tumor marker identification often involves establishing a baseline to measure against during follow-up. Changes in the levels of these markers can correlate with tumor growth or response to therapy. Consequently, healthcare teams can make informed decisions on treatment adjustments or further evaluations depending on the PCR results.
Monitoring Minimal Residual Disease
Monitoring minimal residual disease (MRD) is crucial for understanding long-term treatment responses in cancer patients. MRD refers to the small number of cancer cells that may remain in the body after treatment, which can lead to relapse. PCR is particularly effective in identifying these residual cells at very low levels, providing a powerful tool for monitoring the effectiveness of treatment.
By using PCR to monitor MRD, clinicians can detect the presence of tumor DNA even after apparent remission. Results from PCR can guide further treatment decisions, such as whether to proceed with additional chemotherapy or implement other therapeutic strategies. This role of PCR enhances a clinician's ability to provide tailored care based on the patient’s unique cancer profile.
"The precision of PCR in detecting genetic contradictions makes it a cornerstone in modern oncology practices."
In summary, the applications of PCR in cancer diagnosis encompass a vital spectrum from early detection to ongoing monitoring. Each application not only enhances the accuracy of cancer diagnostics but also informs personalized treatments, making it a cornerstone in oncological research and clinical practice.
Advantages of PCR in Oncology
Polymerase Chain Reaction (PCR) has profoundly impacted the field of oncology by facilitating cancer diagnosis and management. Understanding the advantages of PCR reveals its vital role in not only identifying cancer but also in guiding treatment decisions. This section will address the specific elements that underline the importance of PCR in cancer care and research.
Sensitivity and Specificity
One of the foremost advantages of PCR is its high sensitivity and specificity. Sensitivity refers to PCR’s ability to detect even small amounts of nucleic acids, enabling the identification of cancerous cells that may be present in low quantities. This capability is crucial in early detection, as cancer often begins with a minimal number of aberrant cells that are difficult to identify through traditional methods.
Specificity, on the other hand, signifies how precisely PCR can differentiate between target sequences and non-target sequences. This reduces the chances of false positives, which is particularly essential in cancer diagnostics, where misdiagnosis can lead to inappropriate treatment. The combination of high sensitivity and specificity helps ensure that clinicians can make informed decisions based on accurate test results.
Rapid Results
Another compelling advantage of PCR in oncology is the speed at which results are obtained. Traditional diagnostic methods often require longer timeframes for analysis due to their multi-step processes. In contrast, PCR can provide results within a few hours, allowing for quicker diagnostic decisions. This promptness is vital in a clinical setting where timely diagnosis may significantly impact prognosis and treatment planning.
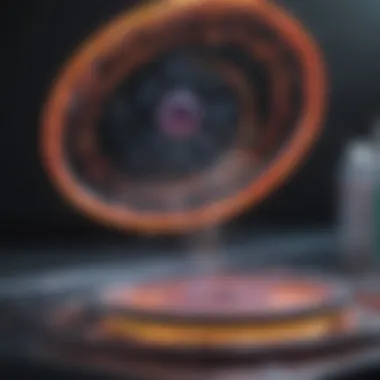
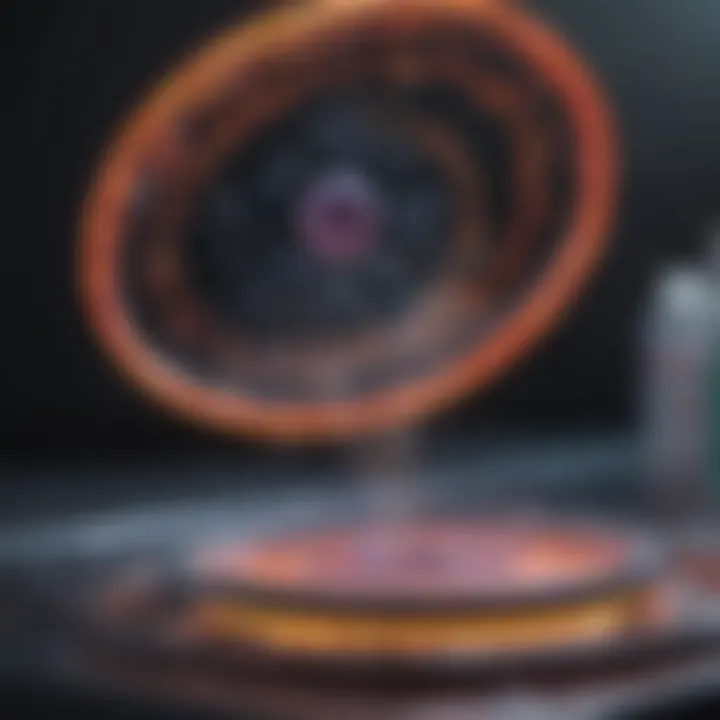
Rapid results enable clinicians to initiate therapy sooner, particularly for aggressive cancers that require immediate intervention. Furthermore, the efficiency of PCR technology helps streamline workflows in laboratories, optimizing resources and improving patient throughput.
In summary, the advantages of PCR lie in its remarkable sensitivity, specificity, and the ability to yield rapid results. These elements enhance the reliability and efficiency of cancer diagnostics, thereby solidifying PCR's essential role in oncology.
Limitations and Challenges of PCR
The Polymerase Chain Reaction (PCR) has significantly advanced molecular biology, particularly in cancer diagnosis. However, despite its numerous advantages, several limitations and challenges must be addressed. Understanding these obstacles is essential for researchers, clinicians, and anyone involved in cancer diagnostics. These limitations impact the accuracy, reliability, and overall effectiveness of PCR in clinical settings.
Potential for Contamination
One of the most significant challenges in PCR is the potential for contamination. Contamination can arise from various sources, including airborne particles, laboratory equipment, or even operator error. Once contamination occurs, it can lead to false positives, which can misguide treatment decisions. To mitigate this risk, laboratories must adopt stringent protocols, including the use of separate areas for pre- and post-PCR processes. These measures help ensure that samples do not come into contact with potential contaminants after amplification.
"Contamination is a serious concern that can compromise the integrity of PCR results. Awareness and preventive strategies are vital for maintaining a reliable testing environment."
Another effective approach is to utilize positive controls in all PCR experiments. Controls help confirm whether the PCR reaction is working correctly, thus allowing researchers to identify issues in results promptly. Such practices can enhance the reliability of PCR, especially in sensitive cancer detection cases.
Interpretation of Results
The interpretation of PCR results presents its challenges. PCR yields data that may not always correlate directly with clinical outcomes. Different variables can influence the results, including the quality of DNA extraction, the efficiency of amplification, and the specificity of the primers used. As a result, inaccurate interpretation can occur if expected outcomes are overly simplified.
Moreover, the quantitative aspect of PCR, particularly in quantitative PCR (qPCR), requires careful consideration. The difference in expression levels can be misleading without an appropriate reference gene or control. This need for normalization can complicate results when comparisons are drawn between different samples.
To improve the interpretation process, professionals should employ standardized reporting guidelines and robust statistical analyses. Continuous training for laboratory personnel in result interpretation is also beneficial to reduce the risk of misunderstanding the data generated from PCR assays.
Regulatory and Compliance Issues
Regulatory and compliance issues further complicate the practical application of PCR in oncology. As PCR technology evolves, so do the regulations surrounding its use in diagnostic settings. Principles of Good Laboratory Practice (GLP) and compliance with local and international standards are crucial for labs dealing with cancer diagnostics. Failure to comply can lead to legal ramifications and hinder access to essential healthcare.
Additionally, the rapid pace of technology development often outpaces regulatory guidelines. This discrepancy can create challenges in validating new PCR methods or modifications before their introduction into clinical practice. The need for rigorous testing and validation can delay the adoption of innovative techniques that could enhance diagnostic capabilities.
To address these issues, researchers and practitioners must stay informed about regulatory updates and compliance standards. Collaboration with regulatory bodies can help bridge the gap between technological advancements and the necessary protocols for clinical implementation.
Recent Advancements in PCR Technology
Advancements in PCR technology are crucial for enhancing cancer diagnosis and research. These innovations not only increase the accuracy and efficiency of PCR but also expand its applications in personalized medicine. As the field progresses, understanding these developments allows researchers and healthcare professionals to harness the full potential of PCR in oncology.
Next-Generation Sequencing
Next-generation sequencing (NGS) is a significant advancement in PCR technology. NGS allows for the simultaneous sequencing of millions of DNA fragments, significantly reducing the time and cost associated with traditional sequencing methods. This capability enables a more detailed analysis of genetic mutations linked to various cancers.
The integration of NGS with PCR techniques improves the detection of rare mutations. This is vital, as certain tumors may carry specific mutations that can inform treatment decisions.
Some key benefits of NGS include:
- Higher throughput: NGS can process many samples at once, facilitating large-scale genomic studies.
- Enhanced accuracy: This method minimizes errors during the sequencing process, leading to more reliable results.
- Comprehensive profiling: NGS provides detailed insights into tumor heterogeneity, allowing for better understanding and treatment planning.
The transformative potential of NGS makes it a cornerstone of modern cancer genomics.
Digital PCR Innovations
Digital PCR (dPCR) represents another remarkable advancement in PCR technology. Unlike traditional PCR, which provides a qualitative measure of target DNA, dPCR quantifies DNA with high precision. It partitions the PCR reactions into thousands of individual chambers, allowing for more accurate enumeration of DNA molecules.
Some advantages of digital PCR include:
- Absolute quantification: dPCR offers exact counts of DNA copies, making it especially useful in detecting low-abundance targets, such as circulating tumor DNA (ctDNA).
- Improved sensitivity: This technology enhances the detection of genetic alterations, even in samples with minimal residual disease.
- Minimized variability: The partitioning approach reduces the effects of inhibitors and variability among reactions.
Digital PCR is particularly relevant for monitoring treatment responses and identifying resistance mutations, providing critical information that can guide therapeutic strategies.
"The integration of digital PCR and next-generation sequencing is reshaping the landscape of cancer diagnostics, paving the way for more personalized and effective treatments."
Role of PCR in Personalized Medicine
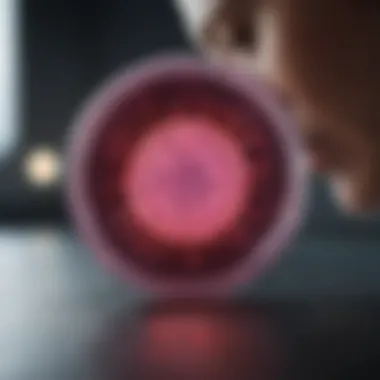
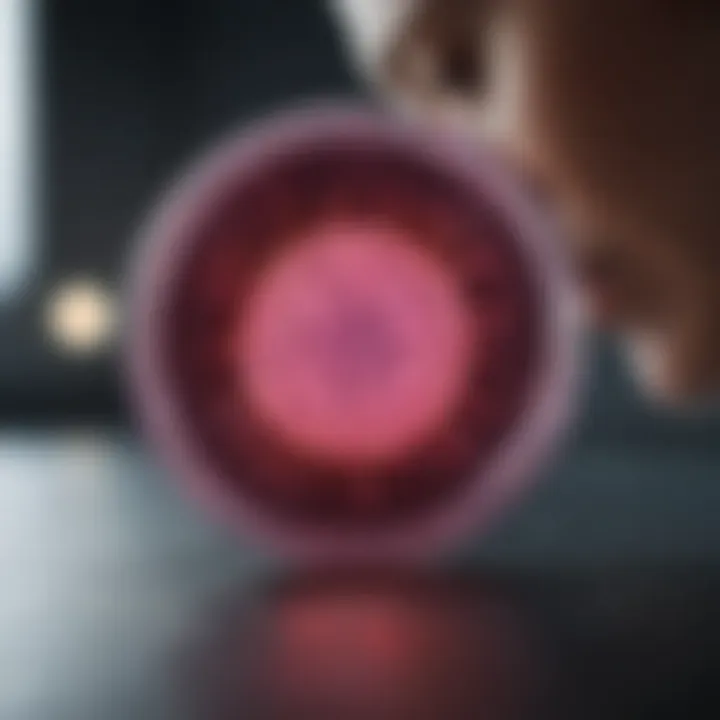
Polymerase Chain Reaction (PCR) technology plays a crucial role in the realm of personalized medicine. This field aims to customize healthcare treatments based on individual patient characteristics. The significance of PCR becomes apparent when we explore its applications in treatment planning and patient care.
One of the most impactful contributions of PCR is in the realm of targeted therapies. Targeted therapies are designed to attack specific molecules involved in the growth and progression of cancer cells. By utilizing PCR, clinicians can identify mutations or alterations in a patient’s tumor DNA. Such identification enables a precise selection of targeted drugs, enhancing treatment efficacy. For instance, in breast cancer, the presence of the HER2 gene can guide the use of trastuzumab. This specificity minimizes unnecessary treatment and side effects, aligning with the core principles of personalized medicine.
Moreover, PCR is pivotal in pharmacogenomics, which is the study of how genes affect a person's response to drugs. Through pharmacogenomic testing, individuals can undergo PCR testing to analyze genetic variants that influence metabolism, efficacy, and safety of drugs. This information allows providers to tailor medication choices and dosages, thereby reducing adverse drug reactions and improving therapeutic outcomes.
"Tailoring treatments to genetic profiles can profoundly enhance the quality and effectiveness of patient care."
Incorporating PCR in personalized medicine leads to several advantages. The primary benefits include:
- Increased Effectiveness: Treatments become more targeted, leading to better patient outcomes.
- Reduced Side Effects: Patients avoid unnecessary treatments that might not be effective for their specific cancer type.
- Enhanced Monitoring: PCR can be used for monitoring responses through real-time assessments of genetic changes.
Nonetheless, the utilization of PCR in this field is not without challenges. Healthcare providers need to ensure informed consent, emphasizing the importance of understanding the implications of genetic testing. Additionally, there is a need for robust ethical guidelines addressing privacy concerns that arise with genetic data handling.
As personalized medicine evolves, the role of PCR will continue to expand, offering innovative testing methods that refine treatment pathways. By identifying specific molecular changes and personalizing treatment strategies, PCR contributes significantly to the advancement of precision oncology.
Ethical Considerations in PCR Usage
The use of Polymerase Chain Reaction (PCR) technology in cancer diagnosis and research opens a range of ethical issues. As PCR becomes more integral to medical practice, it is crucial to address the implications of its deployment. Understanding the ethical dimensions helps guide policies that enhance patient care while safeguarding individual rights.
Privacy Concerns with Genetic Testing
Genetic testing, facilitated by PCR, can reveal critical information about an individual’s predisposition to cancer. However, this information is highly sensitive. Privacy concerns arise when test results are stored and shared. Patients may worry about who has access to their genetic data and how it may be used.
- Data Security: Protecting genetic information from unauthorized access is a primary concern. Strong encryption and stringent data handling protocols are necessary to maintain confidentiality.
- Surveillance Risks: If genetic data is collected by commercial entities, there is a potential for misuse. Companies may use this data beyond original patient consent, leading to discrimination in employment or insurance.
- Long-term Storage: There is also concern about the long-term storage of genetic information. Patients often do not know how long their data will be kept or for what future purposes, raising ethical questions about consent and autonomy.
Informed Consent
Informed consent is a fundamental principle in medical ethics. It requires that patients understand the procedures, risks, and implications of genetic testing before agreeing to it. PCR applications necessitate a robust informed consent process, yet challenges persist.
- Clarity of Information: Patients need clear explanations of what PCR entails, including what the testing might reveal about their health status and implications for relatives. Information should be devoid of jargon and comprehensible to all.
- Voluntariness: Consent must be freely given, without coercion. Patients should feel empowered to ask questions and must be allowed to withdraw consent at any point without facing repercussions.
- Awareness of Outcomes: Ethical considerations also include ensuring that patients are aware of potential outcomes. Not all findings from PCR tests are beneficial or clear-cut. There can be emotional and psychological impacts that patients need to be prepared for.
Future Directions in PCR Research
The future of Polymerase Chain Reaction (PCR) research holds significant promise for advancing cancer diagnostics and treatment. With increased innovation and a growing understanding of genetic material, PCR technology is expected to evolve and yield new methodologies. The journey ahead is not just an extension of current techniques but a transformative phase that integrates breakthrough technologies and concepts.
Emerging Technologies
Emerging technologies are critical to enhancing the power and scope of PCR. One notable area is the development of microfluidic devices. These tools allow for the manipulation of tiny fluid volumes, improving the speed and efficiency of PCR. This technology enables rapid diagnosis, which is crucial for timely cancer interventions.
Another promising innovation is the synergy between PCR and CRISPR, a genome-editing platform. This combination not only enhances the specificity of PCR but also opens doors to targeted treatments tailored to individual genetic profiles. Such advancements contribute to the evolving landscape of precision medicine in oncology.
Furthermore, the integration of artificial intelligence in interpreting PCR results is showing potential. AI can analyze vast datasets to discern patterns that might elude human researchers, ultimately facilitating earlier and more accurate cancer detection.
Integrative Approaches with Other Modalities
As PCR technology progresses, integrative approaches become essential for holistic cancer research. For instance, combining PCR with next-generation sequencing (NGS) enhances genetic profiling accuracy. This synergistic relationship allows researchers to look beyond just DNA amplification to understand the complexities of tumor biology.
Moreover, integrating PCR with imaging technologies like MRI and PET scans can provide a comprehensive view of cancer's molecular and structural characteristics. This approach enhances diagnostic capabilities and can guide personalized therapeutic strategies.
In summary, the future of PCR in cancer research is rich with potential. It demands ongoing adaptation and collaboration across fields to leverage emerging technologies and integrative strategies, emphasizing the core responsibility of researchers to innovate for better outcomes in cancer care.
Culmination
The conclusion encapsulates the essence of PCR's contributions to cancer diagnosis and research. It highlights how PCR technology, through its precision and efficacy, has transformed the landscape of oncology. The intersection of advanced techniques and clinical applications showcases PCR's role as a cornerstone in understanding and treating cancer.
Summary of Key Insights
In summary, this article has addressed multiple facets of PCR in the realm of oncology. Key insights include:
- Mechanisms of PCR: The clarity of PCR's processes establishes a firm groundwork for its usage in medical contexts.
- Applications in Diagnosis: Early detection and tumor marker identification demonstrate PCR's critical function in timely interventions.
- Advantages Over Other Techniques: High sensitivity and specificity make PCR a preferred method in cancer research.
- Challenges: A balanced understanding of limitations, such as contamination and data interpretation, is essential for effective application.
- Future Directions: Innovations like digital PCR and next-generation sequencing mark significant advancements that could reshape cancer treatment paradigms.
These insights not only provide a comprehensive view of PCR's capabilities but also set the stage for ongoing exploration and improvement within the field.
The Ongoing Role of PCR in Cancer Research
PCR remains integral to ongoing cancer research. The technology continuously evolves, adapting to new scientific discoveries and clinical needs. For instance, advancements in digital PCR have allowed for more precise quantification of nucleic acids, aiding in minimal residual disease monitoring. Similarly, the integration of PCR with genomic profiling offers avenues for personalized medicine.
Furthermore, as researchers delve deeper into the genetic underpinnings of cancer, PCR serves as a fundamental tool for exploring gene expression, mutation detection, and the development of targeted therapies. The collaborative efforts between PCR applications and other modalities will likely enhance diagnostic capabilities and therapeutic strategies in the future, underscoring the significance of this technology in the ongoing battle against cancer.