Understanding Multimode Wavelengths: Insights & Applications
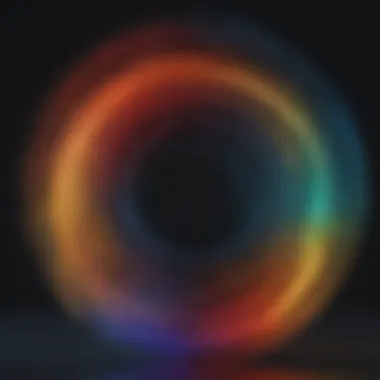
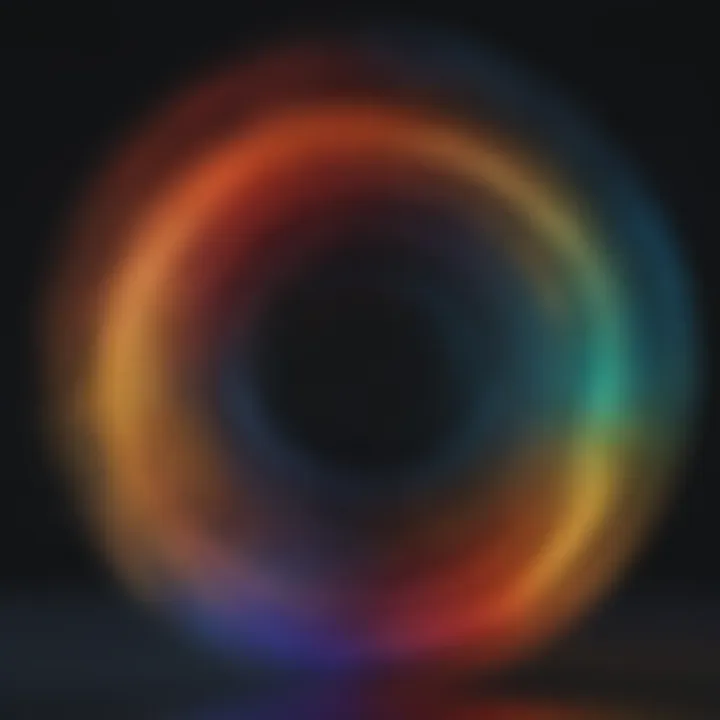
Intro
Multimode wavelengths play a crucial role in the realm of optical communication and various scientific fields. This article aims to dissect the complexities surrounding multimode wavelengths, providing clear insights into their mechanisms and uses. Understanding these wavelengths is essential for advancements in technology and research across multiple disciplines, including biology, chemistry, and physics.
Research Overview
Summary of Key Findings
Recent investigations into multimode wavelengths reveal important distinctions between multimode and single-mode systems. Multimode wavelengths allow multiple light paths within an optical fiber, enhancing data transmission capabilities. This divergence leads to a varied set of implications in terms of signal quality and bandwidth.
Key findings suggest that multimode wavelengths are especially advantageous in systems that require high data throughput but can tolerate some degree of signal distortion. The performance of multimode fibers often exceeds that of single-mode fibers in short-range communication applications, making them suitable for data centers and local area networks.
Relevance to Current Scientific Discussions
The conversation surrounding multimode wavelengths is increasingly significant in contemporary scientific discourse. With the growing demand for faster communication networks, multimode technology presents a viable path forward. Researchers are exploring innovative applications of this technology, leading to a broader impact on digital infrastructure and information technology.
Furthermore, the exploration of multimode wavelengths aligns with developments in quantum computing and photonics. This signifies their potential to revolutionize not just telecommunications but also areas such as biomedical applications and environmental monitoring.
Methodology
Research Design and Approach
The research conducted on multimode wavelengths incorporates a multifaceted approach. It integrates both theoretical and experimental components. Theoretical frameworks help in understanding the principles of light propagation in different fiber types. Experimental studies provide empirical data on performance metrics, such as bandwidth and dispersion.
Data Collection and Analysis Techniques
Data collection typically involves empirical testing of optical fibers under various conditions. This encompasses assessing signal integrity through methods like Optical Time Domain Reflectometry (OTDR). Analyzing the gathered data includes statistical techniques to determine performance trends. Insights derived from this analysis contribute significantly to our grasp of multimode wavelength behavior.
"The study of multimode wavelengths not only enhances our understanding of optical systems but also encourages future innovations in technology and communication."
In summary, a deep dive into multimode wavelengths reveals their critical role across diverse scientific disciplines. Future advancements will continue to shape their application, making this a relevant and intriguing area of study.
Intro to Multimode Wavelength
The concept of multimode wavelength is essential in various fields of science and technology. Understanding it helps researchers and professionals in fields ranging from telecommunications to biology employ these wavelengths effectively. Multimode wavelengths are characterized by the capacity to carry multiple modes or light rays. This feature enhances data transmission and broadens the scope of applications in fiber optics. In examining this subject, it becomes clear why multimode wavelengths are vital for modern advancements.
Defining Multimode Wavelength
A multimode wavelength refers to light waves that can travel through a medium, allowing multiple light paths or modes concurrently. This characteristic distinguishes multimode fibers from their single-mode counterparts, which restrict light to one path. Multimode wavelengths operate effectively over shorter distances, making them ideal for various applications in local area networks and data centers. For example, in fiber optic telecommunications, multimode wavelengths enable higher data throughput but with limits related to distance and signal quality.
To comprehend this definition, it is crucial to recognize the context of wavelengths within electromagnetic theory. The wavelengths interact with the fiber medium, determining the spectrum of light utilized in various technologies. Incorporating multiple light paths can assure better performance in specific situations, leading to the desired efficiency gains in data transmission and imaging technologies.
Historical Context
The historical evolution of multimode wavelengths dates back to initial experiments in fiber optics. In the 1970s, researchers began to recognize the significant potential of multimode fibers, resulting in rapid technological advancements. Early work focused on understanding light transmission through glass fibers, culminating in the development of multimode fibers capable of supporting high data rates. This era saw a surge in interest, leading to improved designs and materials for fiber optics.
Key milestones in this history include:
- The invention of the first optical fiber by W. Keck and D. P. W. K. Turner in 1965.
- The establishment of multimode fibers in the 1970s, enhancing telecommunications.
- Advancements in laser technology enabling multimode fiber to carry dense data packets in the 1980s.
These developments encapsulate the journey toward optimizing multimode wavelengths. From rudimentary experiments to practical applications, the evolution reflects how understanding multimode wavelength has shaped contemporary communication technologies. Today, ongoing research focuses on expanding the capabilities of these wavelengths, ensuring they remain relevant in an ever-evolving landscape.
The Science Behind Wavelengths
Understanding the principles of wavelengths is essential for grasping the nuances of optical communication and various scientific applications. Wavelengths form the backbone of many technologies used in both industrial and research settings. The science behind wavelengths also aids in the comprehension of how light interacts with matter, combined with the relevant implications for fields such as telecommunications, biology, and environmental monitoring.
Wave Properties
Wavelength is a fundamental property of waves. All electromagnetic radiation, including light, can be characterized by its wavelength. In the spectrum of light, wavelengths determine not only the color of the light we perceive but also its energy and potential applications. The wave properties include amplitude, frequency, and speed, each significantly influencing how light behaves.
- Amplitude refers to the height of the wave. Higher amplitude means a brighter light, which can be important in imaging technologies.
- Frequency indicates how many wave cycles pass a given point in one second. Higher frequency light waves carry more energy, impacting applications like laser cutting.
- Speed is constant in a vacuum but varies in different media, affecting how signals propagate in optical fibers.
These properties are intimately connected to various phenomena such as reflection, refraction, and diffraction. Understanding these principles is vital for developing advanced technologies and improving existing systems in areas like telecommunications and medical imaging.
Frequency and Wavelength Relationship
The relationship between frequency and wavelength is crucial in the study of light and optics. This relationship can be described by the formula:
[ c = \lambda f ]
Where:
- ( c ) is the speed of light in a vacuum,
- ( \lambda ) is the wavelength,
- ( f ) is its frequency.
From this formula, it becomes clear that as the wavelength increases, the frequency decreases, and vice versa. This relationship has far-reaching implications:
- In telecommunications, shorter wavelengths are used for higher data transmission speeds.
- In spectroscopy, different frequencies help identify materials by examining how they absorb or emit light.
Understanding this relationship enhances our capacity to design systems for better performance in various applications, including remote sensing and biological imaging.
"The fundamental connection between frequency and wavelength is a cornerstone in advancing optical technologies and scientific research."
Overall, the science behind wavelengths serves as a foundation for exploring, understanding, and applying multimode wavelengths across various domains. This knowledge fosters innovation and improves the efficiency of technologies that rely heavily on the manipulation of light.
Multimode vs. Single-Mode Wavelengths
The comparison between multimode and single-mode wavelengths is fundamental in understanding optical communication systems. Each type has distinct properties that suit different applications and environments. Multimode wavelengths are characterized by multiple light paths through the fiber, which can lead to modal dispersion. This can limit their effective distance for signal propagation. In contrast, single-mode wavelengths, with only one path through the fiber, allow for longer distances with reduced dispersion. Thus, knowing these differences is crucial for selecting the right fiber type for specific applications.
Key Differences
Key differences between multimode and single-mode wavelengths can be summarized as follows:
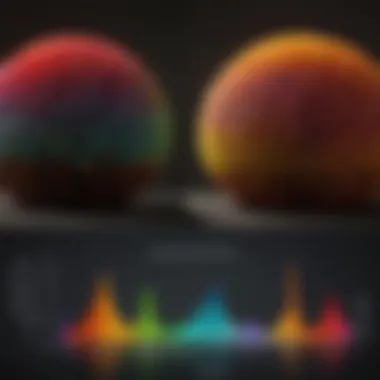
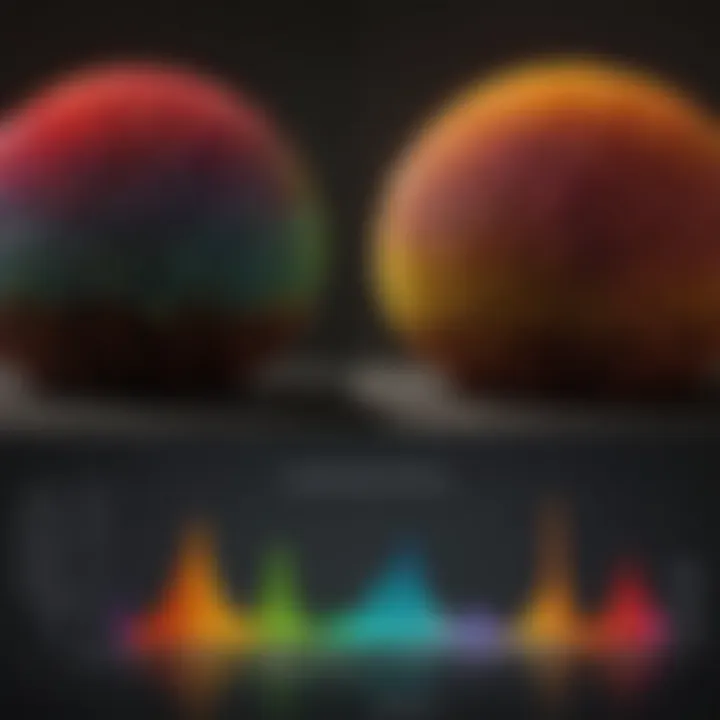
- Structure of Fiber: Multimode fibers have a larger core diameter than single-mode fibers. Typically, multimode fibers range from 50 to 62.5 microns, whereas single-mode fibers usually have a core diameter of about 8 to 10 microns.
- Propagation Modes: Multimode fibers support multiple light modes to travel simultaneously, while single-mode fibers allow only one mode. This results in less data throughput in multimode compared to single-mode under certain conditions.
- Signal Loss and Dispersion: Multimode fibers experience higher modal dispersion due to the geometry of their design. This means that over longer distances, the signal can degrade more quickly compared to single-mode fibers, which are more efficient over longer runs.
- Cost Factors: Generally, multimode fibers are less expensive to manufacture and install. This makes them a popular choice for short-distance communication, such as within buildings. Single-mode fibers, while more costly, are essential for longer distances, including telecommunications and internet backbones.
Applications of Each Type
The application of multimode and single-mode wavelengths spans various sectors. The selection criteria often focus on distance, bandwidth requirements, and specific use cases:
- Multimode Fiber Applications:
- Single-Mode Fiber Applications:
- Local Area Networks (LANs): Multimode fibers are commonly used in LANs where distances do not exceed 2 kilometers. Their ability to handle multiple light paths suits high-bandwidth applications like video conferencing and data transfers within a campus or building.
- Data Centers: In data centers, multimode fibers provide efficient connections between servers due to their lower cost and ease of installation compared to single-mode fibers.
- Broadcast Links: Used in environments such as studios or auditoriums where fiber runs are short and high bandwidth is needed without extreme optical distance losses.
- Telecommunications: Single-mode fibers are ideal for telecommunications where long-distance signaling is necessary. They enable higher data rates and can transmit over distances exceeding hundreds of kilometers.
- Internet Services: Providing backbone connections for Internet service providers, single-mode fibers support high-speed internet needs in urban and rural areas alike.
- High-Speed Data Transmission: They are increasingly used in applications requiring high-speed data transfer, such as fiber-to-the-home installations, where the demand for bandwidth is crucial.
In summary, the choice between multimode and single-mode wavelengths should be driven by the specific needs of a project or application. Understanding these differences can help in optimizing performance and cost-effectiveness in optical networking solutions.
Technical Aspects of Multimode Wavelengths
Understanding the technical aspects of multimode wavelengths is crucial for comprehending their broad applications. The unique characteristics of multimode wavelengths stem from their interaction with various media, particularly in optical fibers. This section addresses specific phenomena relevant to these wavelengths while highlighting their advantages and challenges in practical scenarios.
Understanding Modal Dispersion
Modal dispersion refers to the spreading of signal pulses that occurs when light travels through a multimode fiber. This phenomenon arises because different light modes travel at different speeds. As a result, there can be delays in the arrival times of these modes, causing a distortion of the signal. This aspect is essential in determining how distances can affect the clarity and efficiency of communication systems.
Several factors contribute to modal dispersion.
- Core Size: Multimode fibers typically have larger core diameters compared to single-mode fibers, which allows multiple light paths. However, this design is precisely what causes greater dispersion due to the varying distances traveled by each mode.
- Wavelength: The wavelength of the light used can impact the degree of dispersion. Shorter wavelengths may experience less modal dispersion than longer ones.
- Temperature and Material: The physical properties of the fiber, such as the material it is made from and environmental conditions, can influence the speed and behavior of light modes.
Minimizing modal dispersion is critical for improving the performance of multimode systems, especially in long-distance communications or high-bandwidth data transmission. This can be achieved by selecting appropriate wavelengths or employing specialized waveguide designs.
"Understanding modal dispersion allows for better design decisions in optical systems, impacting performance metrics greatly."
Role of Refractive Index
The refractive index plays a vital role in how multimode wavelengths propagate through optical fibers. It measures how much light bends when it enters a material. Different refractive index profiles can lead to distinct propagation characteristics, influencing the properties of the modes within a fiber.
In multimode fibers, there are typically two primary profiles:
- Step-index Profile: In this design, the core has a uniform refractive index that sharply changes to a lower index in the cladding. This type allows for easy analysis and is basic in structure. However, it can cause greater levels of modal dispersion.
- Graded-index Profile: Here, the refractive index gradually decreases from the core to the cladding. This design reduces modal dispersion since light modes are guided more efficiently towards the core center.
The choice of refractive index has important implications for bandwidth and signal quality in multimode fiber optics. Choosing the right refractive index can lead to enhanced performance in communication systems, offering advantages in transmission efficiency and minimizing signal loss.
Multimode Optical Fibers
Multimode optical fibers are critical components in the field of telecommunications and beyond. They facilitate the transmission of data over short distances using multiple light paths or modes. Compared to single-mode fibers, multimode fibers offer distinct advantages and applications. Understanding their structure, functionality, and various applications gains significance when considering their role in modern communication and technology.
Structure and Functionality
The design of multimode optical fibers consists of a core that is significantly larger than that found in single-mode fibers. Typically, the core diameter of multimode fibers ranges from 50 to 62.5 micrometers. The larger core allows multiple light modes to propagate simultaneously. This structure impacts the fiber's performance, particularly in terms of light propagation and dispersion.
The core material usually involves glass or plastic. These materials help in guiding light effectively within the fiber. The refractive index of the core is higher than that of the cladding, which keeps the light confined to the core, allowing for efficient transmission.
Multimode fibers also rely on modal dispersion, which occurs when different light modes travel at varying speeds. Although this phenomenon can limit bandwidth and affect signal integrity over long distances, it is less pronounced in short-range applications.
However, it's important to note that modal dispersion leads to signal degradation as distance increases. This is a crucial design consideration when implementing multimode fibers in specific applications.
Applications in Telecommunications
In telecommunications, multimode optical fibers play a pivotal role. They are commonly used for intrabuilding connections, local area networks (LANs), and data centers. The benefit of using multimode fibers in these scenarios lies in their capacity for high data transfer rates while maintaining affordability compared to single-mode counterparts.
Some key applications include:
- Local Area Networks (LANs): Due to their ability to transmit large amounts of data over short distances, multimode fibers are frequently utilized in LANs, connecting devices within a confined area.
- Data Centers: The rapid growth of online data storage requires robust intra-data center connections. Multimode fibers are ideal for linking equipment within data centers due to their high bandwidth capability.
- Short-Range Communication: These fibers excel in short-range communication systems, such as video conferencing and streaming applications, where high data rates and low latency are crucial.
The ongoing development in fiber technology continues to enhance the capabilities and applications of multimode fibers. Their importance in telecommunications cannot be overstated, as they provide vital infrastructure that supports modern communication systems.
"In the evolving landscape of technology, multimode fibers have proven indispensable for meeting the increasing demands of data transmission."
In summary, multimode optical fibers represent an essential element in telecommunications, offering unique structures and functionalities that support a variety of applications. Their growing significance mirrors the advancements in communication technologies, ensuring that they will remain relevant in future developments.
Biological Applications of Multimode Wavelengths
The utilization of multilmode wavelengths within the biological field encompasses a broad range of applications, primarily centered on advancements in diagnostic and imaging techniques. This section highlights the importance of these wavelengths in medical and research settings. By understanding their implications, professionals can enhance the accuracy and efficiency of various procedures. Multimode wavelengths facilitate deeper insights into biological systems, which is essential for both basic and applied research.
Medical Imaging Techniques
Multimode wavelengths play a critical role in modern medical imaging. Techniques such as optical coherence tomography (OCT) utilize these wavelengths to provide high-resolution images of tissues. The ability to capture details at a micro-level supports the detection of abnormalities, such as tumors, at earlier stages. These advantages contribute to better patient outcomes through improved diagnosis and timely interventions.
Another application is in fluorescence imaging, wherein multimode wavelengths are effective for observing cellular and molecular processes. Fluorescent markers are used in various scenarios, including cancer research and the study of infectious diseases. The specificity offered by these wavelengths helps in visualizing interactions and dynamics in real time, a key factor in understanding disease mechanisms.
Moreover, in techniques like three-dimensional (3D) imaging of biological samples, the use of multimode wavelengths allows for the reconstruction of complex structures. This is particularly useful in understanding spatial relationships within cells or tissues, leading to enhanced biological insights and research outcomes.
Diagnostic Tools
In diagnostics, multimode wavelengths aid in the development of sensors and assays that are vital for detecting diseases. Techniques like surface plasmon resonance (SPR) benefit from the properties of these wavelengths, providing high sensitivity for biomarker detection. This is crucial in conditions where early detection can significantly impact treatment.
Additionally, biosensors employing multimode wavelengths can offer real-time monitoring of physiological or pathological changes. These tools are instrumental in various applications, such as monitoring glucose levels in diabetes management or detecting cardiac markers during emergencies. Their versatility promotes a shift towards more personalized healthcare solutions, making diagnostics more patient-centric.
"The integration of multimode wavelengths in biological applications enhances the sensitivity and specificity of imaging and diagnostic techniques, facilitating crucial advancements in healthcare."
Overall, the significance of multimode wavelengths in biological applications cannot be overstated. They enhance existing methodologies and foster new avenues for research and diagnostics. As technology continues to evolve, the focus on these wavelengths will likely expand, leading to innovative solutions in understanding and treating diseases.
Chemical Applications of Multimode Wavelengths
Multimode wavelengths play a crucial role in the field of chemistry, specifically in analytical techniques. Their applications are extensive, ranging from spectroscopy to sensing, both of which are vital for studying chemical compositions and reactions. This section highlights these applications, exploring how multimode wavelengths enhance our understanding of chemical phenomena.

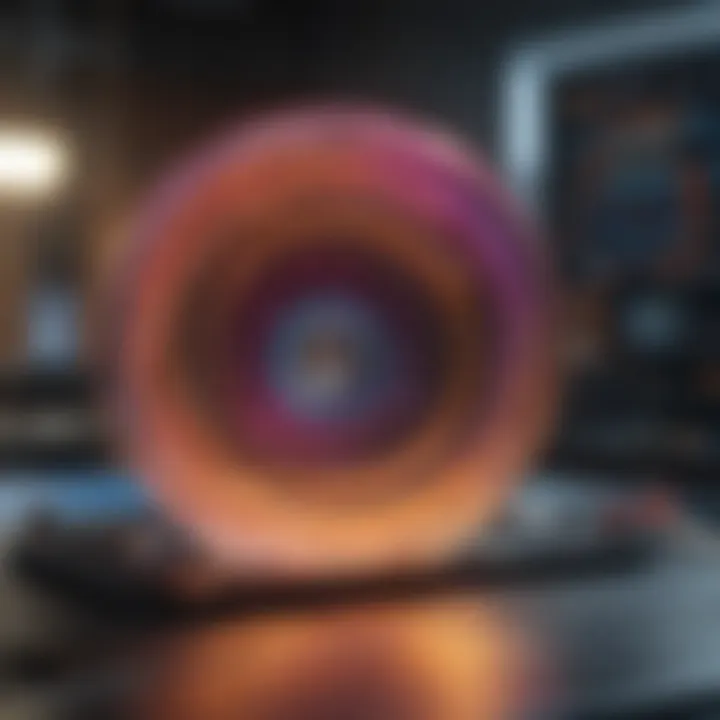
Spectroscopy Techniques
Spectroscopy is a foundational tool in chemistry, enabling scientists to analyze materials based on light interaction. The use of multimode wavelengths in spectroscopy allows for various techniques, including UV-Vis, infrared, and Raman spectroscopy. Each method utilizes the unique properties of light at differing wavelengths to gain insights into molecular structure and behavior.
In UV-Vis spectroscopy, for instance, multimode wavelengths aid in monitoring electronic transitions in molecules. This capability is essential for determining concentration levels of chemical compounds in solutions. Some specific benefits related to this technique include:
- Enhanced sensitivity: Multimode wavelengths improve detection limits, allowing for the analysis of low-concentration samples.
- Broader range of applicability: Multiple wavelengths enable the identification of a wider variety of chemical species.
- Rapid analysis: The ability to collect data at various wavelengths simultaneously results in faster analysis processes.
Raman spectroscopy also benefits from multimode wavelengths, facilitating the study of molecular vibrations. This technique helps identify different chemical species and their operational states. In many instances, multimode wavelengths enhance spectral resolution, allowing chemists to distinguish closely related compounds.
Sensing Applications
Sensing is another critical application of multimode wavelengths in chemical analysis. Chemical sensors utilize these wavelengths for detecting and quantifying substances present in various environments. Multimode wavelengths can enhance the sensitivity and specificity of sensors, making them essential in fields such as environmental monitoring and food safety.
Some notable aspects of sensing applications include:
- Real-time monitoring: Sensors equipped with multimode wavelengths provide data instantaneously. This characteristic is essential for applications requiring continuous monitoring, such as pollutant detection.
- Selective detection: Multimode sensors can be designed to respond selectively to particular analytes, reducing interference from other substances.
- Versatility: These sensors are suitable for diverse applications, including gases, liquids, and solid samples.
Multimode wavelengths significantly add to the reliability and performance of chemical sensors, enhancing their capacity to deliver meaningful data in real-time scenarios.
In summary, the applications of multimode wavelengths in chemical spectroscopy and sensing are indispensable. They enhance analytical techniques, making them more efficient and effective. As technology continues to advance, we can expect further innovations in these areas, ultimately refining our analytical capabilities in chemistry.
Physical Science Applications
The study of multimode wavelengths has significant implications in physical sciences. This section provides an analysis of how these wavelengths find application in various fields, particularly in laser physics and optical communication. Understanding the practical applications and implications of multimode wavelengths is crucial for advancing research and technology.
Laser Physics
Laser physics utilizes the principles of multimode wavelengths extensively. Lasers emitting multimode wavelengths allow for various applications due to their ability to generate multiple light pathways simultaneously. This characteristic enables the use of multimode lasers in applications such as laser scanning, imaging, and material processing.
- Multimode Laser Behavior: multimode lasers can operate in different modes, which influences the shape and quality of the laser beam. This flexibility is often beneficial in applications requiring high power and fast response times.
- Efficiency: Lasers operating on multimode wavelengths often exhibit higher output power across a range of wavelengths, making them suitable for applications requiring strong intensity. Applications in manufacturing benefit significantly from this feature.
- Broadband Applications: Lasers that utilize multimode wavelengths can cover a broader spectrum, which is advantageous in spectroscopy. Their ability to generate multiple wavelengths simultaneously aids in characterizing materials effectively.
"The flexibility and higher output capacity of multimode lasers present numerous opportunities for innovation in various technical fields."
Optical Communication
Optical communication systems deploy multimode wavelengths to enhance data transmission capabilities. This technology mainly utilizes optical fibers, which are designed to transmit signals through multiple paths.
- Increased Bandwidth: Using multimode wavelengths increases bandwidth, allowing a greater amount of data to be transferred simultaneously. This is essential for modern communication systems that require high-speed data exchange.
- Cost-Effectiveness: Multimode fibers are often less expensive to produce compared to single-mode alternatives. This cost advantage makes multimode optical communication a viable option for many organizations.
- Reduced Complexity in Network Design: The ability to connect multiple paths in a multimode optical system reduces the complexity involved in designing network layouts. This aspect facilitates easier installation and maintenance for network administrators.
Implications for Earth Sciences
The implications of multimode wavelengths for Earth sciences are substantial, influencing both the techniques used and the overall comprehension of various environmental processes. The ability to analyze data through different wavelengths allows scientists to observe the Earth in ways that were once challenging. Through multimode wavelengths, researchers gain insights into the physical, chemical, and biological aspects of our planet. This section will elaborate on key areas where multimode wavelengths are especially beneficial, focusing on remote sensing technologies and environmental monitoring.
Remote Sensing Technologies
Remote sensing utilizes various sensor technologies, many of which operate on multimode wavelengths. This method enables researchers to collect data from distant locations without direct contact. Sensors can detect electromagnetic waves reflected or emitted from the Earth’s surface.
- Diverse Applications: Remote sensing can monitor land use changes, urban growth, and deforestation. It allows scientists to gather large datasets for analysis.
- Improved Accuracy: The use of multimode wavelengths contributes to higher accuracy in data collection. Different wavelengths can penetrate materials and provide unique insights into the characteristics of the studied areas.
- Continuous Monitoring: These technologies enable continuous monitoring of ecosystems, which is crucial for understanding ongoing changes due to climate change or human activities.
"Remote sensing has revolutionized how we understand and manage Earth's resources, providing essential data that influences policy and conservation efforts."
Due to these advantages, remote sensing applications in Earth sciences have expanded rapidly, offering powerful tools for geologists, ecologists, and climate scientists.
Environmental Monitoring
Environmental monitoring has gained significance because of increasing environmental concerns. Multimode wavelengths play an essential role in this field by enabling detailed observations and assessments of various environmental factors.
- Pollution Detection: Sensors tailored for specific wavelengths can evaluate air and water quality. Early detection of pollutants is critical for environmental protection and human health.
- Climate Change Analysis: Multimode wavelengths are essential in tracking temperature changes, greenhouse gas emissions, and land surface temperatures. They assist in modeling and predicting climate patterns.
- Biodiversity Preservation: Knowledge gained from studying environmental changes through multimode wavelengths contributes to conservation strategies. Monitoring habitats and ecosystems helps protect endangered species.
By integrating multimode wavelengths into environmental monitoring, scientists can make informed decisions about necessary actions to protect the Earth’s resources. Overall, the implications of multimode wavelengths in Earth sciences reinforce the importance of advanced technologies in understanding and responding to environmental challenges.
Challenges in Multimode Wavelengths
The exploration of multimode wavelengths is not devoid of challenges. Various technical hindrances can significantly impact the performance, application, and feasibility of these wavelengths in different fields. Understanding these challenges is crucial for researchers, educators, and professionals as it helps in devising effective strategies to overcome them.
Signal Degradation
Signal degradation presents a notable challenge in the realm of multimode wavelengths. Unlike single-mode fibers that predominantly carry light in one path, multimode fibers allow multiple light paths. This diversification leads to modal dispersion, where different paths may cause signals to arrive at different times.
Such delays distort the integrity of the signal. In applications that require precise timing, like telecommunications, signal degradation can result in connectivity issues or data loss. Moreover, the cumulative effect of dispersion can limit the bandwidth over long distances, restricting the data transmission capabilities.
"Addressing signal degradation is fundamental to ensuring the efficiency of multimode wavelengths in various sectors."
Strategies to mitigate signal degradation involve careful design of fiber structures. Employing graded-index fibers can help minimize modal dispersion, enhancing signal clarity. Additionally, continuous monitoring and testing can help identify degradation early, allowing for timely interventions.
Cost and Efficiency Concerns
Cost and efficiency are persistent considerations in the deployment of multimode wavelengths. While initial investments in multimode optical systems may be lower when compared to single-mode systems, the operational costs can accumulate. Maintenance and replacements can escalate due to the challenges posed by signal degradation. In environments where high reliability is paramount, these costs become a significant concern.
Furthermore, efficiency losses due to modal dispersion create a cycle of increased operational demands. Users may find themselves compelled to invest in advanced equipment or technologies to maintain performance. This situation leads to further discussions around the overall cost-effectiveness of multimode fibers in various applications.
In summary, addressing the challenges related to signal degradation and cost is essential for enhancing the practicality and performance of multimode wavelengths. Researchers and industry practitioners must focus on ongoing improvements and innovations to overcome these hurdles.
Future Trends in Multimode Wavelength Research
In recent years, the landscape of multimode wavelength research has evolved significantly. Understanding these trends is essential for grasping how current advances shape various fields. The exploration of multimode wavelengths opens doors to innovations and presents challenges that demand attention. As technology progresses, it prompts ongoing evaluation of their implications and applications.
Advances in Fiber Technology
Fiber technology plays a central role in the utilization of multimode wavelengths. Recent developments have focused on enhancing performance, efficiency, and applicability across diverse fields. Key advancements include new materials and manufacturing techniques that improve signal integrity and minimize loss.
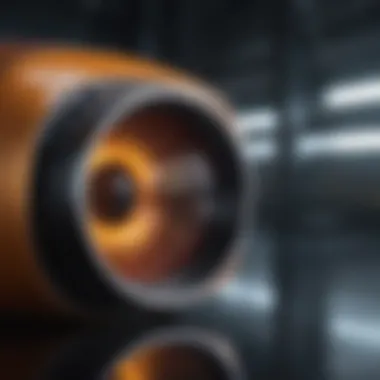
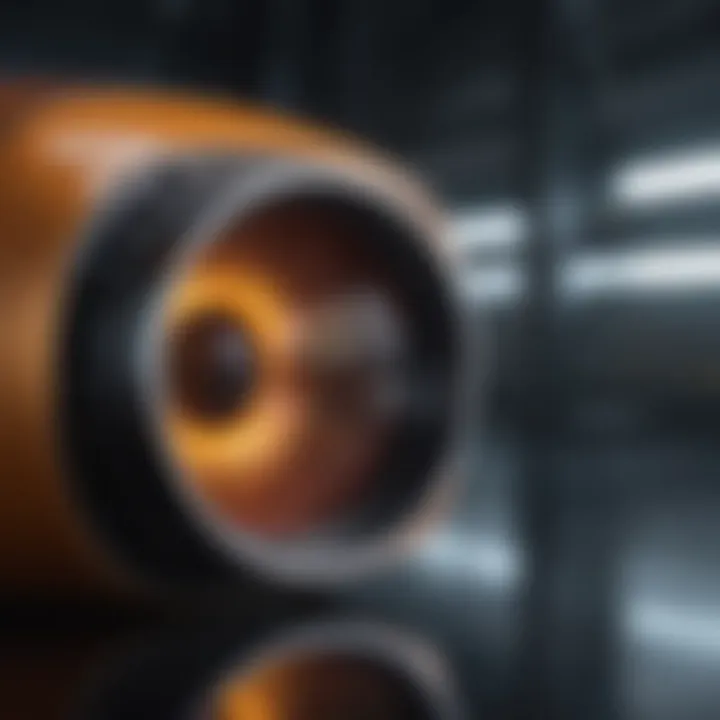
- Enhanced Core Design: Innovations in core design of multimode fibers can lead to better light transmission, thus reducing modal dispersion. This ensures that signals can travel longer distances while maintaining clarity.
- Multi-Core Fibers: Research into multi-core fibers allows for multiple signals to be transmitted simultaneously without interference. This is crucial for data-intensive applications like telecommunications and network infrastructures.
- Photonic Crystal Fibers: These fibers use sophisticated structures to manipulate light more effectively. They can support various multimode wavelength applications in sensing and imaging.
The integration of these technologies is vital for meeting the growing demands of sectors such as telecommunications, imaging, and sensing. Continuous improvement in fiber technology ensures a robust foundation for future research.
Potential Innovations
The potential for innovation in multimode wavelength applications is extensive. Current investigations into dynamic modulation techniques can redefine how we utilize these wavelengths in practical scenarios.
- Dynamic Wavelength Allocation: Using algorithms to dynamically adjust wavelengths can improve system efficiency and response time in networks, especially in high-traffic scenarios.
- Adaptive Optics: Incorporating adaptive optics can enhance image clarity in biomedical imaging, allowing for precise diagnostics and treatment planning.
- Quantum Dots in Fiber Technology: Research on integrating quantum dots into fiber optics could significantly impact the performance of multimode systems, especially in sensing applications, by enhancing sensitivity and selectivity.
"The future of multimode wavelengths is deeply intertwined with advances in technology. Innovations in fiber materials and designs will pave the way for more efficient, high-performance systems across various industries."
In summary, the future trends in multimode wavelengths reveal a path of ongoing development and exploration. As technology progresses, these advances not only alter current applications but also prompt new ones, highlighting the relevance of multimode wavelengths in both academia and industry.
Multimode Wavelengths in Educational Research
Understanding multimode wavelengths holds significant value in educational research. As scientific inquiry evolves, integrating core principles of optical technology into education becomes increasingly vital. This understanding can enhance various teaching methodologies and interdisciplinary approaches, ultimately enriching the educational landscape.
Teaching Methodologies
Incorporating multimode wavelengths into teaching methodologies fosters a comprehensive scientific perspective. Educators can leverage the principles of these wavelengths to create practical and theoretical frameworks tailored to students' learning needs. For instance, laboratory experiments that demonstrate light propagation through multimode fibers engage students actively. Such experiential learning promotes better retention of knowledge compared to traditional memorization methods.
Further, multimedia resources can illustrate complex concepts clearly. Videos and simulations showing how multimode wavelengths operate in different environments help demystify the technical content. This integration encourages students to explore and challenge assumptions about optical technologies. The initiative to use practical applications, such as telecommunications or medical imaging, can lead to a deeper appreciation of multimode wavelengths in real-world contexts.
Benefits of Teaching Multimode Wavelengths:
- Enhanced Engagement: Practical experiments make learning tangible.
- Critical Thinking: Students learn to analyze and apply concepts across different scenarios.
- Collaboration Opportunities: Group work in labs promotes teamwork and communication.
Interdisciplinary Approaches
Multimode wavelengths cross disciplinary boundaries, making them a rich topic for interdisciplinary approaches in education. By connecting principles from physics, biology, and chemistry, educators can demonstrate the broad impact of this field on various scientific domains. For instance, in a combined physics and biology course, students might explore how multimode wavelengths are used in medical imaging technologies. This application could link optical physics with practical healthcare solutions.
Encouraging collaboration between different subject areas results in a holistic educational experience. The integration of multimode wavelengths provides a platform for discussions on ethical considerations in technology deployment, scientific communication, and environmental sustainability.
Furthermore, addressing challenges faced in various fields through the lens of optical technology highlights the relevance of multimode wavelengths. Students gain a well-rounded understanding of how a foundational concept can influence research and development in multiple scientific domains.
“To understand the world around us, one must appreciate the intricate connections across scientific disciplines.”
Integrating knowledge of multimode wavelengths into educational practices is essential for nurturing informed, innovative thinkers.
Comparative Analysis with Other Technologies
In the realm of optical wavelengths, comparative analysis with other technologies is crucial. It allows for a better understanding of how multimode wavelengths fit into the broader landscape of optical communication, sensing, and other applications. This analysis highlights the strengths and weaknesses of different wavelength technologies, helping researchers and professionals make informed decisions.
Understanding how multimode wavelengths perform against alternatives not only clarifies their utility but also identifies potential areas for improvement. For example, multimode wavelengths typically offer advantages in terms of cost and ease of use in certain situations. However, they may lack the performance efficiency seen in single-mode wavelengths.
This contrast leads to several considerations:
- Efficiency: Single-mode fibers usually support higher bandwidths over longer distances. In comparison, multimode fibers excel in shorter, high-volume environments.
- Cost-effectiveness: Multimode systems, generally less expensive, are ideal for local area networks where budget constraints are significant.
- Complexity of installation: Multimode solutions are often simpler to install and require less precision in alignment compared to single-mode systems.
Analyzing these factors contributes to an understanding of when to opt for multimode solutions versus other technological options.
"Comparative analysis provides clarity in selecting the right wavelength technology based on specific needs."
Wavelengths in Industry Applications
The application of wavelengths within industry contexts illustrates the practical value of multimode technology. Industries such as telecommunications rely heavily on multimode fibers. These fibers facilitate high data transfer rates over short distances, commonly used in campus networks and data centers.
Applications also extend to biomedical fields. Multimode wavelengths are instrumental in diagnostics and imaging technologies. For example, they enhance the accuracy of techniques like optical coherence tomography, which is vital in medical imaging.
Cross-Disciplinary Applications
Multimode wavelengths find applications across various disciplines, fostering interdisciplinary collaboration. In environmental science, they contribute to sensing technologies that monitor climate variables.
In education, multimode wavelengths aid in developing hands-on learning experiences. By harnessing the principles of optics, students from diverse fields can engage with practical experiments. Furthermore, this cross-disciplinary approach encourages innovation and creativity among researchers.
Ultimately, the comparative analysis with other technologies emphasizes the importance of multimode wavelengths. This understanding fosters advancements in both theoretical and practical applications, enriching the body of knowledge in the field.
Culmination
The examination of multimode wavelengths reveals their significance across various scientific domains. Their multifaceted nature contributes distinct advantages, particularly in fields such as telecommunications, medicine, and environmental science. In this concluding section, we will underscore key findings, elaborate on their relevance, and propose avenues for further research.
Summary of Findings
Multimode wavelengths offer a unique range of applications stemming from their ability to accommodate multiple light paths within optical fibers. This property enables higher data transmission rates, which is crucial in modern communication networks. In the domain of medical imaging, technologies utilizing multimode wavelengths enhance diagnostic precision and outcomes. The discussion also highlighted several key distinctions between multimode and single-mode wavelengths, particularly in terms of signal fidelity and application scope. Key findings include:
- Increased Data Capacity: Multimode wavelengths effectively manage increased traffic in data networks.
- Medical Diagnostics: The use of multimode wavelengths in imaging augments diagnostic capabilities, providing clearer insights into biological tissues.
- Research Implications: Their applications in spectroscopy and remote sensing have opened new avenues in scientific exploration, impacting environmental monitoring and chemical analysis.
These findings illustrate that multimode wavelengths are not just a technical concept, but a pivotal element enhancing the functionality of various systems.
Recommendations for Future Study
While significant advances have been made concerning multimode wavelengths, there remain many areas ripe for exploration. Future studies could focus on the following aspects:
- Investigation of New Materials: Research into alternative fiber materials could enhance performance, reducing signal degradation.
- Integration with Emerging Technologies: Exploring the interplay between multimode wavelengths and quantum technologies might yield innovative solutions.
- Cost-Efficiency Analyses: Detailed studies on the cost versus performance dynamics could predict trends that support wider adoption in sensitive industrial sectors.
- Cross-Disciplinary Studies: Encouraging collaboration between engineers and researchers from medical and environmental fields to develop integrated solutions.
In summary, understanding and advancing the science of multimode wavelengths is crucial. Continued research and innovative applications are imperative to fully harness their potential in both practical settings and academic discourse.
Citing Relevant Literature
Citing relevant literature is not just a formality. It serves several purposes:
- Validation of Claims: References add credibility to the points made throughout this article. By acknowledging the work of previous scholars and practitioners, we validate the significance of multimode wavelengths in practical applications.
- Resource for Further Reading: Scholars, students, and professionals often seek to expand their understanding of a subject. References allow for a deeper exploration of multimode wavelengths, guiding readers to primary and secondary sources. This includes extensive studies, peer-reviewed articles, and reputable online databases such as Wikipedia and Britannica.
- Engagement with Ongoing Research: The field of optics and wavelength studies is evolving. Citing current research reveals advancements, trends, and challenges. This can encourage discussions and collaboration among researchers, leading to innovation.
Moreover, acknowledging diverse sources fosters an atmosphere of knowledge-sharing. A conscientious reference list might comprise:
- Peer-reviewed journals
- Academic books
- Conference papers
- Online resources from reputable organizations
"A well-structured reference list signifies rigorous research and respect for original thought."