Understanding Chip Sonication: Principles and Applications
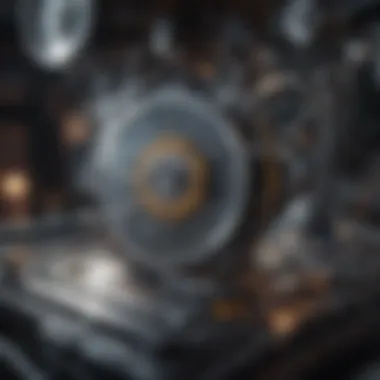
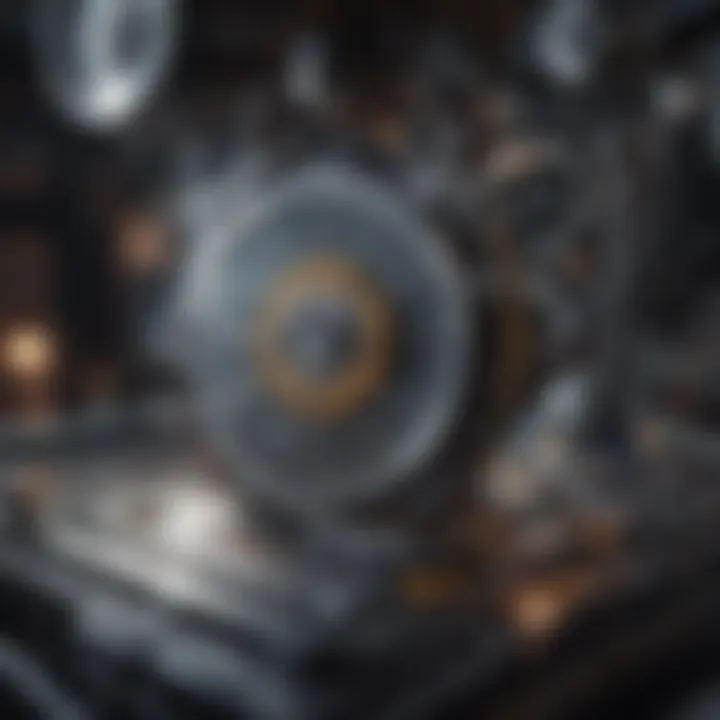
Intro
Chip sonication plays an essential role in enhancing material properties. This technique utilizes ultrasonic waves to achieve desired outcomes in various scientific fields, from biology to materials science. The intricacies of how chip sonication operates present significant insight into its applications and effects.
Effective understanding of this methodology is crucial for students, researchers, educators, and professionals. Through diving into the mechanics of chip sonication, we shall elucidate its principles and offer a comprehensive exploration of its multiple applications.
Research Overview
Summary of Key Findings
Chip sonication employs high-frequency sound waves to disrupt molecular structures in materials. This technique leads to superior mixing, dispersion, and extraction processes. Numerous studies show that it improves the solubility of compounds and increases reaction rates when facilitating chemical processes.
It has been notably beneficial in biological studies, where cell lysis is required for analysis of samples. Chip sonication can also enhance the surface properties of polymers and optimize the performance of nanomaterials.
Relevance to Current Scientific Discussions
Current scientific discussions focus on the efficiency and impact of sonication in innovative research. The advent of new ultrasonic technologies enhances precision and control over the sonication process. Increasingly, interdisciplinary approaches leverage chip sonication, thereby expanding potential applications.
Understanding chip sonication is timely; it converges with modern needs for sustainable practices and efficient material production.
Methodology
Research Design and Approach
Research into chip sonication involves experimental methodologies that assess its effectiveness across various applications. This includes designing experiments that incorporate variables such as sonic frequency, duration, and intensity. The approaches vary according to fields, highlighting sonication's versatility in research contexts.
Data Collection and Analysis Techniques
Data collection utilizes both quantitative and qualitative methods, depending on the application. The use of controls helps ensure reproducibility of results. Commonly employed analysis techniques include spectroscopic methods and microscopy to evaluate results post-sonication. Collaboration between different scientific disciplines often brings about novel insights into data interpretation.
By examining chip sonication through these lenses, a clearer understanding of its fundamental principles and applications emerges. This opens avenues for future research and practical implementations.
Prelims to Chip Sonication
Chip sonication represents a pivotal technique in the realm of scientific research, impacting various disciplines by facilitating a range of processes from material modification to biological applications. Understanding this technique is crucial not only for its operational principles but also for recognizing its adaptability and effectiveness across diverse fields. By delving into the core principles and applications, this section sets the stage for a deeper understanding of how chip sonication enhances research methodologies.
Definition and Overview
Chip sonication can be defined as the application of ultrasound energy to a sample using a specialized device known as a sonicator or ultrasonic bath. The primary purpose of this technique is to induce cavitation within fluids, a process that leads to localized high pressures and temperatures, ultimately facilitating various transformations of the sample. The application of intense ultrasound generates waves that traverse through the medium causing molecular agitation. This process proves beneficial in fields such as molecular biology, material science, and chemical engineering. By breaking down complex materials into finer components, chip sonication has become crucial for sample preparation, systematizing biochemical extractions, and influencing material characteristics.
Historical Context
The practice of sonication in scientific research dates back several decades, evolving through significant technological advancements. Initially, ultrasonic waves were utilized for cleaning purposes in industrial settings. However, researchers soon discovered their potential in facilitating more complex interactions at a molecular level. The transition from mere cleaning to scientific applications began in the late 20th century. Innovators recognized that ultrasound could induce chemical reactions, promote dispersion, and enhance extraction efficacy. Over the years, various types of sonicators and ultrasound generators have been developed, contributing to specialized methods for materials and biological samples alike. Today, chip sonication stands at the forefront of many advanced research methodologies, combining its historical significance with modern innovations to yield profound impacts in contemporary science.
"Chip sonication is not just a tool; it is a transformative force in research, bridging old practices with new technological frontiers."
Through this exploration of chip sonication, we aim to underscore the importance of understanding its principles, operational mechanisms, and wide-ranging applications. Subsequent sections will dissect these dimensions further, offering a holistic view of both the advantages and limitations inherent in this technique.
Fundamental Principles of Sonication
Understanding chip sonication requires knowledge of its fundamental principles. These principles not only govern the functionality of the technique but also shape its applications across various scientific disciplines. The significance of grasping these principles lies in their direct impact on the effectiveness and outcomes of sonication processes.
Acoustic Waves and Their Properties
Acoustic waves are at the core of sonication technology. These waves are essentially pressure variations traveling through a medium, which is typically a liquid in the context of chip sonication. When ultrasound technology is employed, the frequency of these waves typically exceeds 20 kHz, putting them beyond human hearing.
The properties of acoustic waves include:
- Frequency: Higher frequencies penetrate materials more effectively, often resulting in improved disruption of cellular structures.
- Amplitude: This determines the intensity of the energy delivered to the sample. Greater amplitude can enhance the efficacy of the sonication process.
- Wavelength: Shorter wavelengths can interact more directly with smaller structures, essential in applications like nanoparticle synthesis.
Essentially, the effectiveness of chip sonication hinges on optimizing these properties. Adequate control of frequency and amplitude is crucial for achieving desired outcomes in various applications, including research and industrial processing.
Transducer Functionality
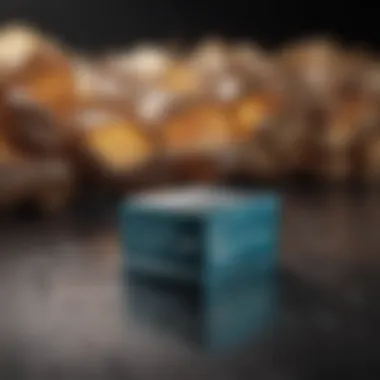
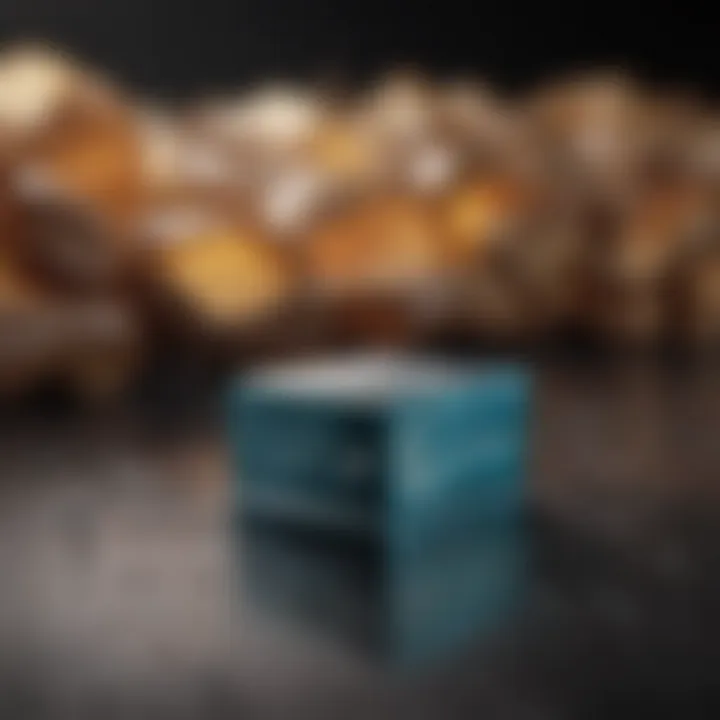
The transducer serves as a critical component in sonication processes. It converts electrical energy into acoustic energy, enabling the generation of ultrasound waves. Understanding its functionality is key for effective implementation of the technique.
The transducer operates based on piezoelectric principles, which involve:
- Electromechanical Conversion: When an alternating current is applied to the piezoelectric material, it vibrates and generates ultrasound waves.
- Efficiency: The efficiency of this conversion directly affects the energy transferred to the medium. Higher efficiency translates to more effective sonication.
- Design Variability: Transducers come in various designs, each suited for specific applications. Choosing the appropriate type is vital for achieving optimal sonication outcomes.
In the context of chip sonication, understanding how transducers work helps in selecting the right equipment and settings for precise and effective applications.
"Knowing the fundamental principles of chip sonication allows researchers and professionals to maximize the potential of this technology in achieving desired results across various fields of study."
By exploring the foundational elements of acoustic waves and transducer functionality, one can better appreciate the mechanics of chip sonication and its implications in areas such as biological research, material science, and beyond.
Mechanism of Chip Sonication
The mechanism of chip sonication is pivotal to understanding its efficacy and application across various scientific fields. This section elucidates the fundamental processes involved in chip sonication—specifically the generation of ultrasound waves and the resulting cavitation phenomena. These elements not only define the operational principles of the technology but also underscore its advantages and limitations.
Generation of Ultrasound Waves
Ultrasound waves, which are sound waves at frequencies higher than the audible range, play a critical role in chip sonication. The generation of these waves occurs through transducers, which convert electrical energy into mechanical energy. This conversion technique enables precise control over the amplitude and frequency of the ultrasound waves produced.
When ultrasound waves propagate through a medium, they create regions of high and low pressure. This dynamic pressure variation is what facilitates the disruption of cellular structures and the enhancement of material properties. For instance, in biological applications, ultrasound waves can effectively penetrate cells, leading to cell disintegration. This method is often preferred over traditional methods due to its rapidity and efficiency.
The advantages of ultrasound wave generation include:
- Precision: Able to target specific frequencies for distinct applications.
- Scalability: Flexible design allows for both small-scale lab setups and large-scale industrial applications.
- Adaptability: Can be adjusted based on the specific requirements of the material or biological sample being processed.
Cavitation Phenomenon
Cavitation is a significant effect resulting from the propagation of ultrasound waves. When ultrasound waves create areas of low pressure, tiny vapor-filled bubbles form within the liquid. As the pressure returns to normal, these bubbles collapse violently, generating localized high temperatures and shockwaves. This collapsing process causes significant disruption to surrounding materials, which can be harnessed for a variety of applications.
The implications of cavitation extend into multiple fields. In biological research, it can enhance protein extraction and DNA shearing by breaking down cellular membranes effectively. In material science, it aids in creating nanoemulsions and improves polymer processing efficiency through enhanced dispersion of materials.
Key characteristics of cavitation include:
- Localized Energy Release: The energy released during bubble collapse can exceed thousands of degrees Celsius, providing a potent means for physical disruption.
- Non-Thermal Effects: While cavitation generates heat, the process can be controlled to minimize damage to thermally sensitive samples.
- Wide Applicability: Useful in fields like chemical engineering, food science, and bioengineering.
Cavitation driven by ultrasound waves is a cornerstone in many contemporary research methodologies, shaping how we approach material modifications and cellular manipulations.
Applications of Chip Sonication
Chip sonication proves to be a pivotal tool across several scientific disciplines. Its applicability cuts through biological research as well as material science, making a profound impact on how researchers manipulate materials and study biological systems. The technique leverages ultrasonic energy to produce precise and efficient outcomes, thereby enhancing both sample preparation and analysis efficiency. These applications extend beyond academic interest; they are vital to innovations in biotechnology, pharmaceuticals, and material engineering. Understanding these applications is essential for professionals who rely on cutting-edge methods to achieve accurate results and drive research forward.
Use in Biological Research
Cell Disruption
Cell disruption is one of the primary uses of chip sonication in biological research. This process is crucial for releasing cellular contents and facilitating the study of biological molecules, such as proteins and nucleic acids. The main characteristic of cell disruption through sonication is its efficiency; it can rapidly break down cell membranes without excessive damage to the molecules of interest.
A significant benefit of using chip sonication is the ability to precisely control the energy delivered, minimizing unwanted interactions. However, a disadvantage might be that excessive energy can lead to the shearing of sensitive biomolecules, making careful optimization necessary for different cell types.
DNA Shearing
DNA shearing is another critical application wherein chip sonication is employed to obtain fragmented DNA suitable for various sequencing techniques. This process is essential for methods like next-generation sequencing, where high-quality, sheared DNA is necessary. The key characteristic here is the controllability; researchers can adjust sonication parameters like pulse duration and power to achieve the desired fragment sizes.
Despite its benefits, such as uniformity in fragment size, the downside lies in the potential irreversibility of certain damages to the DNA backbone if not properly optimized. This can lead to reduced yields during downstream applications, thus requiring optimal conditions for effective results.
Protein Extraction
In protein extraction, chip sonication provides a robust method to free proteins from cellular and tissue matrices. The primary advantage of this technique is speed; it allows for rapid extraction, which is crucial when dealing with labile proteins that may degrade if extraction is delayed. The unique feature of this method is its ability to coexist with harsh solvents, ensuring compatibility across various extraction protocols.
Nevertheless, the challenge here is that high frequency and power levels may denature sensitive proteins, altering their functionality. Therefore, the application of chip sonication in protein extraction requires careful parameter selection for successful outcomes.
Role in Material Science
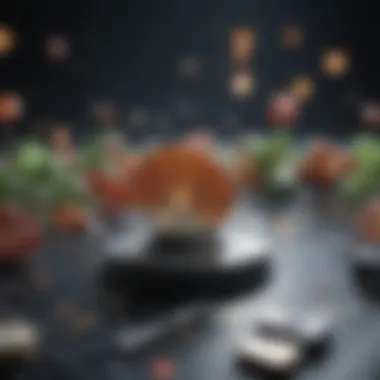
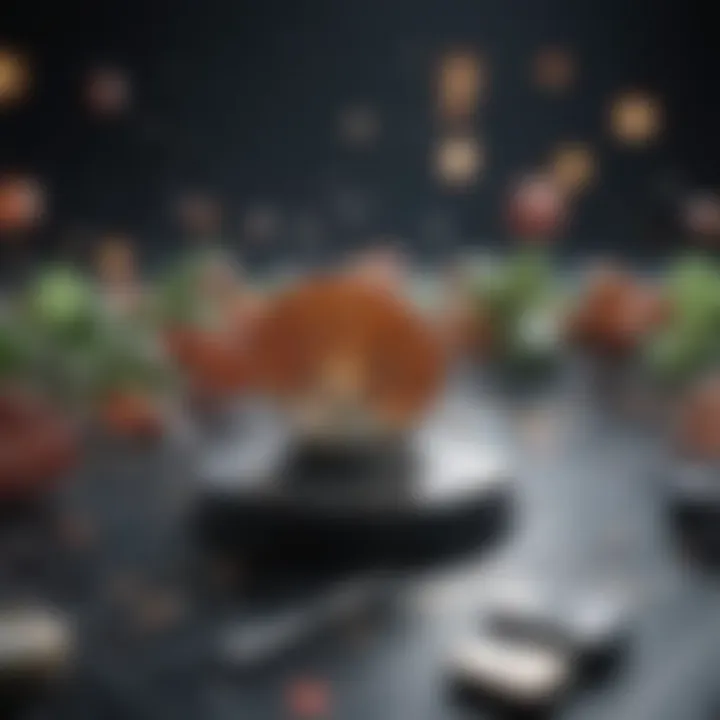
Nanoemulsions and Nanoparticles
In material science, chip sonication plays a vital role in the creation of nanoemulsions and nanoparticles. These materials are significant for their broad applications, from drug delivery systems to cosmetic formulations. The essential characteristic of using sonication in this context is its capability to produce fine dispersions effectively. By employing ultrasonic waves, researchers can achieve a high degree of uniformity in particle sizes, enhancing stability and performance in applications.
However, one may consider that the process requires strict control over conditions to avoid clustering or instability of the formed structures, which can diminish their intended functionality. Proper implementation proves paramount in attaining desired properties and efficiency.
Polymer Processing
The integration of chip sonication in polymer processing showcases the technique's versatility. Sonication facilitates the homogeneous mixing and dispersion of various components within polymers, resulting in superior material properties. This method stands out for its energy efficiency and ability to achieve robust mechanical properties in composite materials.
Yet, while the benefits are considerable, certain limitations exist. The sonication process can introduce thermal effects that may alter polymer characteristics negatively. Calibration of power and duration is essential to ensure the integrity of the polymers during processing.
By comprehensively examining the applications of chip sonication, we recognize the often-overlooked nuances that impact its effectiveness in various fields. Its continued development and integration can lead to advanced methodologies paving the way for future innovations.
Advantages of Chip Sonication
Chip sonication has numerous advantages that contribute significantly to scientific research and material development. The technique stands out in its ability to produce reliable outcomes while drastically improving workflows across various domains. These advantages stem from the core attributes of the sonication process, making it an indispensable tool in both biological and material sciences.
Increased Efficiency in Sample Preparation
One primary advantage of chip sonication lies in its capacity to enhance efficiency during sample preparation. Traditional methods of cell disruption and extraction can be time-consuming and often yield inconsistent results. In contrast, chip sonication minimizes preparation time significantly. This efficiency is not only vital for research timelines but also for experimental accuracy.
When utilizing chip sonication, the application of ultrasound waves allows for rapid disruption of cells and other structures, leading to effective extraction of biomolecules or materials in a fraction of the time required by conventional methodologies. For instance, in biological research, sonication can achieve cell lysis and recovery of nucleic acids much quicker than mechanical or chemical methods.
Moreover, the precision of chip sonication allows researchers to fine-tune parameters such as amplitude and duration, leading to optimized extraction protocols. This adaptability ensures reproducible sample preparations, which is essential for valid experimental comparisons.
Minimized Contamination Risks
Another essential advantage of chip sonication is the reduction of contamination risks during sample processing. In research settings, maintaining sample integrity is crucial, especially when dealing with sensitive biological materials.
By employing chip sonication, the chances of cross-contamination are significantly diminished, as the technique often requires fewer handling steps compared to traditional approaches. With less manual intervention, the likelihood of introducing external contaminants reduces markedly.
Furthermore, chip sonication can be conducted in closed systems or specialized containers, providing an environment that shields samples from potential contaminants in the laboratory atmosphere. This aspect is particularly important in clinical and research labs where purity is paramount.
Effective control of contamination can lead to reliable data, significantly impacting research outcomes.
As a result, chip sonication emerges as a preferred method in studies requiring high-quality samples, such as genomic analyses or proteomic evaluations. By streamlining preparation processes and ensuring minimal contamination, chip sonication supports rigorous scientific standards in research.
Limitations of Chip Sonication
In any scientific endeavor, it is crucial to understand not just the benefits but also the limitations of a technique. Chip sonication, while powerful, has certain drawbacks that researchers must consider. Awareness of these limitations helps in the design of experiments and the interpretation of results.
Potential for Heat Generation
One significant limitation of chip sonication is the potential for heat generation during the process. When ultrasound waves propagate through a medium, they can cause friction, leading to an increase in temperature. This is particularly concerning in biological applications where sensitive components, like proteins or nucleic acids, may be denatured if exposed to excessive heat. The temperature rise can vary based on parameters such as power output and duration of sonication. Researchers must, therefore, monitor the temperature carefully, employing cooling techniques or adjusting parameters to mitigate this issue. By controlling heat generation, the integrity of the sample can be preserved as desired results can be achieved.
Requirements for Optimization
Another aspect that needs attention is the requirement for optimization in chip sonication. Successful outcomes depend on the appropriate calibration of sonication parameters such as duration, frequency, and power. Each application may necessitate a different set of conditions for optimal performance. For instance, sonication for cell lysis may differ markedly from that required for DNA shearing. Not optimizing these parameters can lead to subpar results or complete failure of the experiment. An understanding of the sample properties and the specific goals of the experiment is essential. Continuous experimentation and protocol adjustments often become necessary to achieve the most effective sonication conditions. This iterative process can become a resource-intensive endeavor, highlighting the importance of sufficient planning and trial runs before commencing critical experiments.
"Optimization of parameters is key in achieving desired results and preventing biological damage during sonication."
By acknowledging the limitations of chip sonication, researchers can navigate potential pitfalls while capitalizing on its strengths. This understanding not only fosters better experimental design but also enhances the reliability of data obtained from sonication-based procedures.
Comparative Techniques to Chip Sonication
Comparing chip sonication to other techniques is essential for understanding its unique advantages and potential drawbacks. Each method has its place, depending on the specific requirements of a research or industrial application. In this section, we will discuss two prominent alternatives to chip sonication: mechanical disruption and enzymatic methods. Understanding these techniques informs decisions regarding sample preparation and processing.
Mechanical Disruption
Mechanical disruption involves the use of physical force to break down cells or materials into smaller components. This technique can include methods such as grinding, milling, or bead beating. It is often employed to achieve substantial cell lysis, allowing for the release of cellular contents like proteins and nucleic acids.
Key Considerations of Mechanical Disruption:
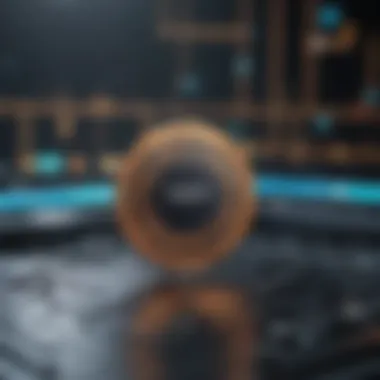
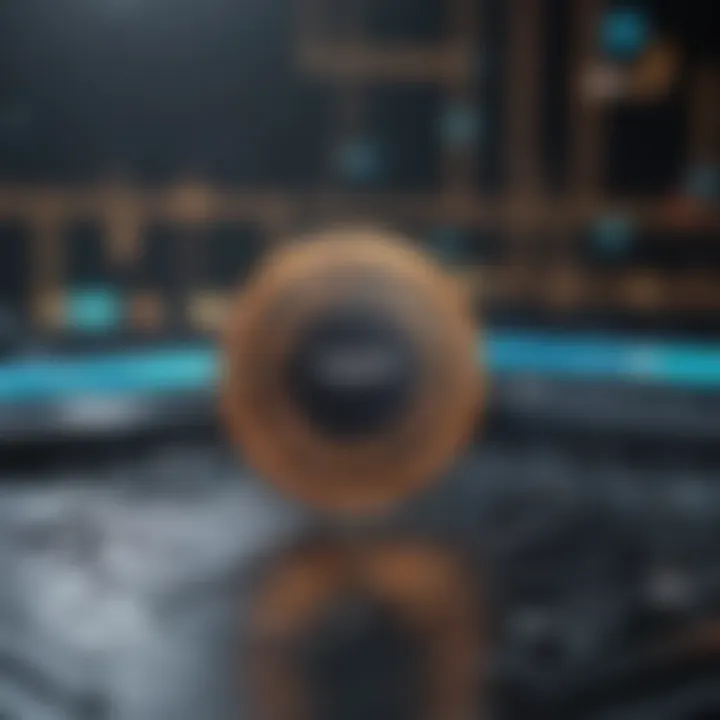
- Force Application: The effectiveness of mechanical disruption is highly dependent on the force and duration applied. Different materials may require tailored approaches.
- Sample Integrity: Overly aggressive mechanical methods can lead to extensive damage, resulting in lower yields of intact biomolecules.
- Equipment Costs: Equipment such as homogenizers or bead mills can be costly and may require specific maintenance.
This technique is useful in cases where chip sonication might induce unwanted heat or where chemical stability is critical.
Enzymatic Methods
Enzymatic methods leverage biological catalysts, such as lysozyme or proteases, to facilitate the breakdown of cellular structures. These enzymes cleave specific bonds in substrates, allowing for controlled and gentle lysis of cells or tissues.
Advantages of Enzymatic Methods:
- Specificity: Enzymatic action is highly specific, which reduces the risk of damaging other biomolecules in the sample.
- Mild Conditions: These methods tend to operate under milder conditions which can preserve sensitive structures that might otherwise degrade.
- Ease of Use: Many enzymatic protocols are straightforward and can often be performed simply by mixing components without complex setups.
However, enzymatic methods also come with constraints. For example, the effectiveness is influenced by factors such as temperature and pH, which need careful monitoring.
Innovations in Chip Sonication Technology
Innovations in chip sonication technology represent a significant evolution in how researchers approach sample preparation and analysis across various scientific disciplines. These advancements enhance the overall efficiency, precision, and applicability of the sonication process. By integrating modern technologies, researchers can achieve more controlled and reliable outcomes, thereby broadening the scope of potential scientific applications.
Automation and Real-time Monitoring
The incorporation of automation in chip sonication systems has transformed traditional methodologies. Automation reduces dependence on manual inputs, leading to consistent results and minimizing operator error. Automated systems can handle variables such as time, intensity, and temperature with precision. This is particularly important when processing biological samples, where every parameter can significantly affect the outcome.
Real-time monitoring is another critical feature of contemporary chip sonication technology. It allows researchers to observe changes in parameters during the sonication process. For example, sensors can track cavitation bubbles' formation and collapse, helping to optimize conditions instantaneously. This responsiveness not only enhances the quality of results but also aids in troubleshooting potential issues as they arise.
Being able to adjust parameters dynamically, based on monitored data, plays a crucial role in ensuring that sample integrity is maintained while allowing researchers the flexibility to tailor the sonication process to specific needs.
"Automation and real-time monitoring are game changers in sonication technology. The ability to fine-tune processes in real-time leads to greater reproducibility and data comparability across studies."
Integration with Other Analytical Techniques
Innovations in chip sonication also extend to its integration with other analytical techniques, which considerably improves the scope and depth of research. By merging sonication with methods such as mass spectrometry, chromatography, and electron microscopy, researchers can obtain a more comprehensive understanding of complex samples.
For instance, combining sonication with mass spectrometry can enhance the extraction of biomolecules, allowing for more effective downstream analysis. This synergy contributes to more informative data, revealing insights that may not be achievable through isolated techniques.
Moreover, this integration streamlines workflows, enabling scientists to analyze samples without extensive preparatory steps. In a fast-paced research environment, such efficiencies can lead to faster results and a more agile investigative process. It also encourages multidisciplinary approaches, fostering collaboration between fields such as biochemistry, materials science, and nanotechnology.
Future Directions in Chip Sonication Research
The future of chip sonication research holds significant promise and an array of opportunities across multiple sectors. Understanding these future directions is essential for researchers and professionals aiming to leverage this technology effectively. As scientific advancements continue to unfold, the ability to adapt and integrate chip sonication with emerging technologies will drive innovation and improve various applications.
Expanding Applications in Industry
Chip sonication has a growing impact on different industries beyond its traditional uses.
- Food Industry: In food technology, chip sonication is being explored for emulsifying and enhancing nutrient extraction. The capability to improve product stability can lead to healthier food products.
- Pharmaceutical Industry: The pharmaceutical sector benefits from sonication in drug formulation processes. The method helps in altering the physical and chemical properties of drugs, ensuring enhanced bioavailability and effectiveness.
- Biotechnology: Research in biotechnology is witnessing advancements due to chip sonication. This technique can facilitate faster cloning, modification, or synthesis of DNA.
- Cosmetic Products: Manufacturers are utilizing sonication to enhance the mixing of formulations, contributing to better texture and absorption of active ingredients in skin care products.
The successful integration of chip sonication into these industries will not only streamline processes but also enhance product quality, thereby meeting evolving consumer demands.
Potential in Personalized Medicine
The concept of personalized medicine focuses on tailoring medical treatment to the individual characteristics of each patient. Chip sonication could play a pivotal role in this field by enabling more precise and effective methodologies.
- Genetic Analysis: By facilitating the efficient shearing of DNA, chip sonication can improve genetic analysis processes crucial for personalized treatment plans. It allows for a better understanding of genetic variations that can guide therapy decisions.
- Cell Manipulation: Chip sonication technology can aid in manipulating cells for stem cell research or regenerative medicine. This manipulation can lead to novel treatment strategies designed specifically for individual patient needs.
- Drug Delivery Systems: The potential for developing customized drug delivery systems is significant. By combining chip sonication with nanotechnology, researchers can create more effective and targeted delivery methods for therapeutic agents.
"The evolution of chip sonication could redefine boundaries of research and application, opening doors to unprecedented methodologies and solutions."
As professionals and researchers recognize the potential impact of chip sonication, further investment in this technology will likely yield fruitful results, enhancing its relevance in both industry and healthcare.
Finale
In summarizing the significance of chip sonication, it is essential to recognize its pivotal role in modern scientific research. This technology harnesses the power of ultrasonic waves to enhance material properties and streamline various experimental procedures. Chip sonication is not merely a methodology; it represents a convergence of precision and efficiency that can lead to remarkable advancements in multiple disciplines.
A core element of this discussion is the vast range of applications that chip sonication encompasses. From biological fields such as cell disruption and DNA shearing to material science applications including polymer processing, the versatility of this technique cannot be overstated. Each application offers unique benefits, making chip sonication a go-to tool for researchers seeking reliable and reproducible results.
The exploration of advantages, including increased efficiency in sample preparation and minimized contamination risks, strengthens the case for adopting chip sonication in scientific practices. Moreover, when juxtaposed against the limitations identified, such as potential heat generation and the necessity for optimization, it becomes clear that thoughtful implementation is crucial.
Effective use of chip sonication requires careful consideration of factors like sample type, desired outcomes, and the operational parameters of equipment.
"Understanding chip sonication involves both acknowledging its myriad benefits and being mindful of its constraints."
This dual perspective ensures that researchers can maximize the potential of this technique while mitigating any downsides. Looking ahead, the future directions of chip sonication research appear promising, especially in expanding industry applications and personalized medicine potential. As technology evolves, new innovations will likely enhance the existing capabilities of sonication, further broadening its scope within scientific inquiry.