Exploring Three-Dimensional Measurements in Research
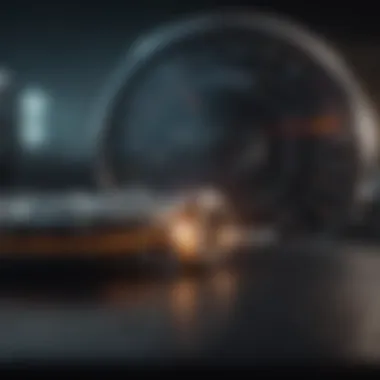
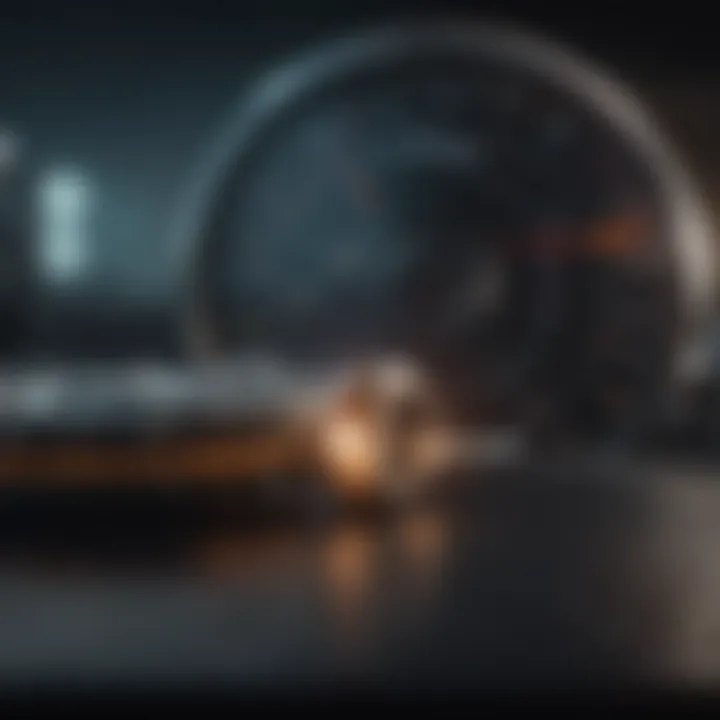
Intro
The exploration of three-dimensional measurements is critical in contemporary scientific research. These measurements have wide-ranging implications across various disciplines, including biology, physics, and engineering. In an era where data drives innovation, understanding the nuances of 3D measurement becomes increasingly vital. This article seeks to outline the foundational concepts, methodologies, and applications associated with three-dimensional measurements, spotlighting their significance in scientific inquiry.
Research Overview
Summary of Key Findings
Three-dimensional measurements enhance our ability to analyze complex structures and phenomena. Key findings show that these measurements:
- Improve accuracy in research outputs.
- Facilitate advanced visualization and modeling of scientific data.
- Drive the development of new technologies and applications in various fields.
The precision provided by 3D measurements can directly affect experimental results and interpretations. Understanding spatial relationships enhances data analysis and can prompt new research questions.
Relevance to Current Scientific Discussions
The relevance of three-dimensional measurements in today’s scientific discussions cannot be overstated. As various fields expand, the demand for precise and reliable measurement techniques rises. Ongoing debates surrounding data accuracy and technology integration further anchor the importance of 3D measurements.
Accurate three-dimensional measurements are fundamental to the integrity of scientific research, influencing everything from data interpretation to technological advancement.
Methodology
Research Design and Approach
A structured approach to three-dimensional measurements is crucial in ensuring validity and reliability. Research design often includes qualitative and quantitative methods tailored to specific scientific needs. This design could involve:
- Selection of appropriate measurement techniques (e.g., laser scanning, photogrammetry).
- Definition of research parameters and objectives.
- Establishment of control mechanisms for data collection.
Data Collection and Analysis Techniques
Data collection in 3D measurements typically involves sophisticated tools and techniques. Commonly employed methods include:
- Laser Scanning: Capturing precise spatial data of objects or environments.
- Photogrammetry: Utilizing photographs to measure dimensions of objects.
- Computed Tomography: A non-destructive method for obtaining 3D representations of objects.
Once collected, data analysis techniques include statistical analysis, modeling, and visualization, which assist in interpreting complex data sets effectively.
Prologue to Three-Dimensional Measurements
Three-dimensional measurements play a crucial role in various scientific fields. They provide a framework for quantifying physical objects in three-dimensional space. From healthcare to engineering, the need for accurate measures is vital for successful research and development.
Understanding three-dimensional measurements helps researchers accurately represent and analyze physical phenomena. For example, accurate 3D modeling assists medical professionals in planning surgeries or creating prosthetics. In engineering, these measurements guide the manufacturing of components with precision.
Additionally, advancements in measurement technologies have transformed how data is collected and analyzed. Researchers must be aware of these evolving tools to reap their benefits. Understanding 3D measurement opens the door to enhanced innovation and improved outcomes in practical applications.
Defining Three-Dimensional Measurements
Three-dimensional measurements refer to quantifying the spatial properties of an object within three distinct dimensions: length, width, and height. This concept extends beyond simple linear measurements and incorporates relationships between various points in space.
In technical terms, 3D measurements may involve techniques such as coordinate measurement systems or surface modeling. The importance of these measurements lies in their ability to provide a more comprehensive view of an object.
Without them, numerous fields would struggle to achieve precision in their work. For instance, architects rely on 3D measurements to create detailed blueprints, while engineers use them to ensure parts fit together correctly in manufacturing.
Historical Context and Evolution
The history of three-dimensional measurements traces back to ancient civilizations that utilized basic geometry for construction and art. The Egyptians and Greeks contributed significantly to the understanding of spatial dimensions. However, it wasn’t until the advent of sophisticated technologies, like laser scanning and photogrammetry, that 3D measurement achieved significant accuracy.
In the late 20th century, the integration of computer technology revolutionized the field. Software advancements allowed for more straightforward manipulation and analysis of three-dimensional data. As a result, three-dimensional measurements became indispensable across various disciplines.
Today, with innovations like 3D printing and virtual reality, the importance of accurate three-dimensional measurements continues to grow. This historical evolution highlights the necessity of adapting measurement practices to meet modern demands.
Theoretical Framework
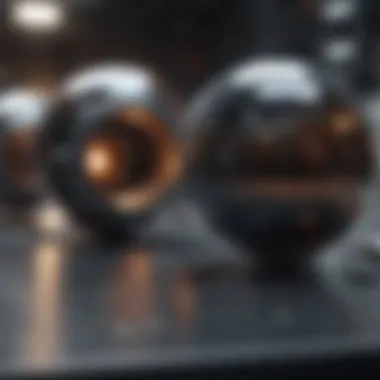
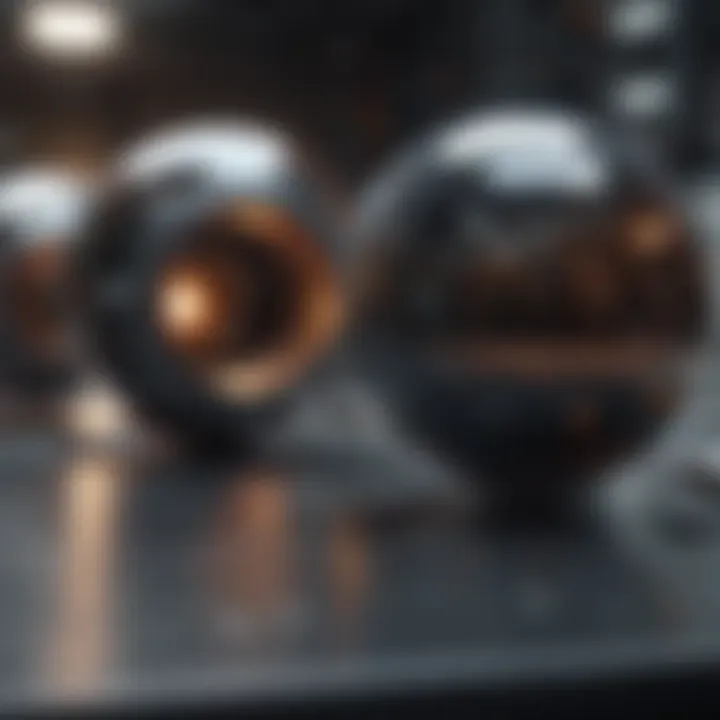
The theoretical framework serves as the backbone for understanding three-dimensional measurements. It is crucial in establishing a foundation on which various methodologies can be built. This framework encompasses mathematical principles and geometric concepts that are necessary for accurately capturing and analyzing three-dimensional data.
Through this framework, researchers and practitioners can understand the underlying theories that govern measurement techniques. Mathematical models provide the tools to quantify dimensions and relations in three-dimensional space. This is important not just for academic studies, but also for practical applications in fields like engineering, biology, and environmental science.
Mathematical Foundations
The mathematical foundations of three-dimensional measurements revolve around several key concepts. Coordinate systems are one of the fundamental elements. The Cartesian coordinate system, for example, allows us to represent points in 3D space using three-dimensional coordinates (x, y, z). This system is essential for translating physical space into mathematical values.
Vector calculus also plays a significant role. Vectors are used to define both direction and magnitude in three dimensions. Understanding how to manipulate vectors allows for deeper insights in applications such as force analysis in engineering or growth patterns in biology.
Another important aspect is the concept of tensors, which extend the idea of vectors. Tensors are crucial for dealing with different types of data in multiple dimensions. This is especially relevant in fields like fluid dynamics, where measurements might not only be three-dimensional but also change over time.
Geometric Principles
Geometric principles are central to interpreting three-dimensional measurements correctly. Basic geometric shapes, such as points, lines, and surfaces, form the basis for building more complex models. Understanding the relationships between these shapes is key for accurate measurements. For example, recognizing how a cylinder's volume can be calculated using its radius and height impacts various practical applications in manufacturing and engineering.
Moreover, similarity and congruence in geometry are vital. These concepts allow researchers to compare measurements across different objects and contexts, improving the robustness of their analyses. 3D shape recognition also taps into geometric principles for applications ranging from computer graphics to medical imaging.
Measurement Techniques
Measurement techniques play a critical role in the accuracy and reliability of three-dimensional measurements in scientific research. Selecting the appropriate technique directly influences the outcomes of experiments and applications. This section reviews manual measurement approaches, digital measurement tools, and comparative techniques. Each technique offers unique benefits and considerations, ultimately contributing to the broader field of 3D measurement.
Manual Measurement Approaches
Manual measurement approaches often involve traditional tools such as calipers and rulers. These techniques have been used for decades and are fundamental in many scientific disciplines. The primary advantage of manual methods is their accessibility. Most of these tools are simple, intuitive, and require minimal training for effective use. However, the accuracy of manual measurements can be impacted by the skill of the operator. Small errors can occur due to parallax or improper reading of scales.
In precise research environments, this approach may introduce variability in data, necessitating careful calibration. Some key points to consider with manual measurement include:
- User Skill: The competency of the individual measuring plays a significant role.
- Calibration: Regular checks are essential to maintain accuracy.
- Environmental Factors: Conditions such as temperature and humidity can affect readings.
Overall, while manual approaches can suffice for basic tasks, they may fall short when high precision is required.
Digital Measurement Tools
Digital measurement tools have revolutionized three-dimensional measurement practices. Instruments like laser scanners and coordinate measuring machines offer advantages that manual methods can rarely match. Digital tools provide enhanced accuracy, repeatability, and often speed up the measurement process. Key benefits include:
- Precision: These tools can produce measurements with a high degree of accuracy.
- Data Storage: Digital measurements can be easily saved and processed for further analysis.
- Ease of Use: Many advanced tools come with user-friendly interfaces and software integration.
However, potential downsides include the initial cost and the need for training to operate more complex equipment effectively. As such, while digital measurement tools can significantly enhance the quality of research, users must approach their integration with consideration for cost and training.
Comparative Techniques
Comparative techniques involve using two or more methodologies to verify results. This approach can highlight discrepancies and reinforce the validity of measurements. For example, a researcher may compare results from a laser scanner with those obtained through manual calipers to establish a confidence level in the data accuracy. This bifurcated approach provides crucial benefits:
- Validation: Confirmation of results through different methods ensures robustness.
- Error Detection: Contrasting results allows for the identification of consistent errors.
- Broader Insight: Leveraging various techniques can yield deeper insights into measurement issues.
In light of these points, adopting comparative techniques fosters a more comprehensive understanding of measurement results. This methodological diversity ultimately enhances the scientific validity of research outcomes.
Applications of Three-Dimensional Measurements
Understanding the applications of three-dimensional measurements is crucial in various fields of research and industry. Three-dimensional measurements provide valuable data that can enhance accuracy, inform decision-making, and foster innovation. The significance of these measurements extends across multiple disciplines, including biology, engineering, and environmental science. By applying 3D measurement techniques, researchers and professionals can better visualize, analyze, and interpret complex data, leading to improved outcomes and advancements.
In Biology and Healthcare
In biology and healthcare, three-dimensional measurements have revolutionized the way we understand and treat various conditions. Medical imaging techniques such as MRI and CT scans utilize 3D measurement principles to create detailed images of internal structures and organs. This level of detail is essential for accurate diagnosis and treatment planning. Here are some key points to consider:
- Precision in Treatment: Accurate 3D measurements enable healthcare practitioners to visualize tumors or other conditions in their precise locations. This improves surgical planning and reduces risks during procedures.
- Personalized Medicine: 3D models derived from patient data help in creating tailored treatment plans, leading to better patient outcomes.
- Research and Development: In drug development, biological models can be 3D printed to study the effects of new drugs, allowing for more efficient testing processes.
In Engineering and Manufacturing
Three-dimensional measurements in engineering and manufacturing play a pivotal role in ensuring quality and efficiency. Techniques such as laser scanning and computer-aided design (CAD) allow for precise measurements that are critical in producing complex structures and components. The following elements highlight the importance:
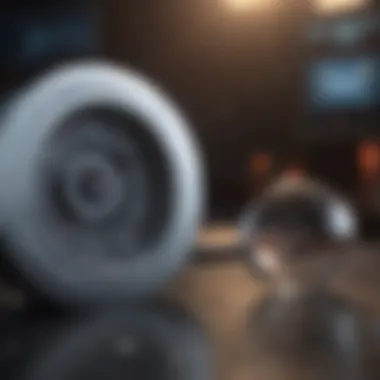
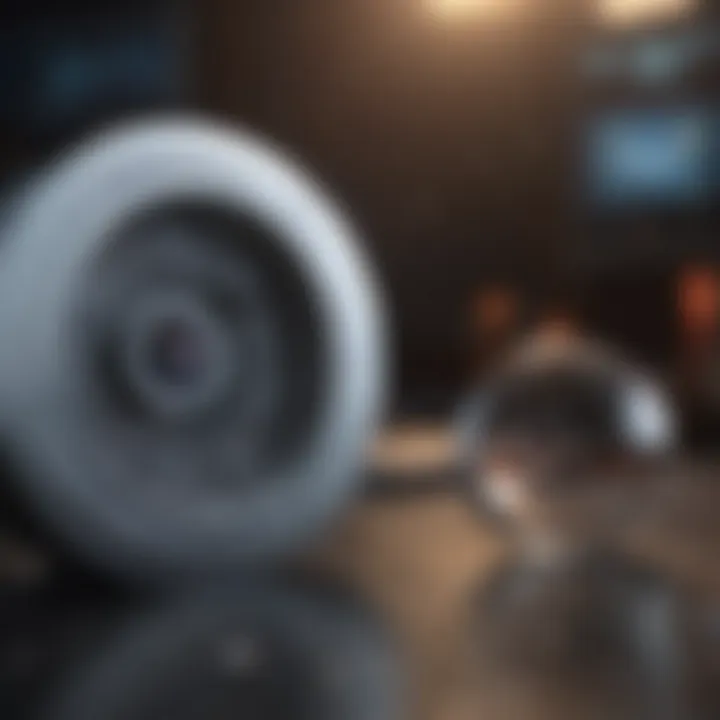
- Quality Control: Engineers can monitor products against strict specifications through 3D measurements, ensuring that every dimension adheres to design requirements.
- Rapid Prototyping: Additive manufacturing technologies rely on accurate 3D measurements to create prototypes quickly, reducing development times and costs.
- Design Validation: Before manufacturing, engineers can use 3D measurements to validate designs, identifying potential issues before production starts.
In Environmental Science
Environmental science benefits greatly from three-dimensional measurements in various applications such as ecological modeling, climate research, and resource management. 3D models of ecosystems or landscapes help researchers understand spatial relationships and dynamics in nature. Consider these aspects:
- Spatial Analysis: Environmental scientists use 3D measurements to analyze terrain and vegetation spatially, providing insights into biodiversity and ecosystem health.
- Climate Change Studies: Modeling temperature, precipitation, and other factors in three dimensions allows better predictions and understanding of climate change impacts.
- Conservation Efforts: Accurate 3D mapping of habitats contributes to conservation efforts by identifying critical areas for protection and restoration.
Three-dimensional measurements are vital in advancing knowledge and technology across diverse fields, shaping our approaches to complex challenges.
Through the insights gained from three-dimensional measurements, researchers and professionals can achieve better outcomes and drive innovation in their respective fields.
Technological Innovations
Technological innovations are pivotal in the realm of three-dimensional measurements, enhancing accuracy and expanding possibilities across various fields. These advancements not only streamline measurement processes but also contribute to the integration of complex data sets, which is essential for modern scientific research. The focus on improved tools and software has led to heightened measurements at microscopic and macroscopic levels, influencing everything from medical imaging to architectural design.
Advances in Imaging Techniques
The development and refinement of imaging techniques represent a major transformation in how three-dimensional measurements are captured and analyzed. Techniques such as computed tomography, magnetic resonance imaging, and laser scanning are at the forefront of these advances. Each method offers distinct benefits depending on the context of its use.
- Computed Tomography (CT): This technique provides high-resolution images that help in diagnosing ailments in medical fields. The resolution levels allow for precise internal views, aiding surgeons and doctors in planning.
- Magnetic Resonance Imaging (MRI): While primarily used in healthcare, MRIs also contribute significantly to understanding biological structures in research settings. The ability to visualize soft tissues in detail is invaluable.
- Laser Scanning: This method has applications in fields like architecture and civil engineering. It enables the creation of detailed 3D models of environments, which aids in preservation, construction planning, and urban development.
These techniques integrate seamlessly into research methodologies, producing not just data, but actionable insights that drive innovation.
Software Integration
Software integration plays a critical role in harnessing the power of 3D measurements. As various data collection methods proliferate, the need for robust software systems that can analyze this data has become more pressing. Creativity in analysis is just as important as data collection.
Some key considerations include:
- Data Processing: Advanced software enables the processing of large data volumes, which traditionally presented significant challenges. Software such as Autodesk and SolidWorks can efficiently handle raw data from imaging techniques to create visualizations and models.
- Interactivity and Collaboration: Many platforms allow multiple users to work simultaneously on 3D models. This is particularly important in collaborative research environments. Real-time updates and corrections can reduce time and enhance accuracy.
- Visualization Tools: Tools for data visualization are crucial for interpreting 3D measurement results. Software like Tableau and MATLAB provides features that turn complex datasets into digestible visual formats, allowing better decision-making and insights.
Technological innovations in imaging and software integration have reshaped the landscape of three-dimensional measurements, offering new capabilities and opportunities for researchers and professionals. As these technologies advance, one can expect to see further enhancements in precision and application across disciplines.
Data Analysis in Three-Dimensional Measurements
Data analysis plays a crucial role in understanding and interpreting three-dimensional measurements. It is not merely about collecting data; it is about transforming that data into actionable insights. In the realm of three-dimensional measurements, data analysis involves the synthesis of large datasets generated through various measurement techniques. Understanding how to process and analyze this data effectively is essential for researchers and professionals who rely on precise measurements in their work.
The importance of data analysis can be outlined through specific elements:
- Enhancing Accuracy: By applying correct statistical methods, researchers can minimize errors in measurements. This leads to more accurate results and valid conclusions, which is vital in scientific research.
- Interpreting Complex Data: Three-dimensional data can be complex. Data analysis techniques help researchers derive meaningful patterns and correlations from this complexity.
- Supporting Decision-Making: In fields like healthcare and engineering, the results of three-dimensional measurements inform critical decisions. Effective data analysis enables stakeholders to base their decisions on robust findings.
- Facilitating Communication: Data analysis also assists in visualizing measurements in a way that is comprehensible to various audiences, from scientists to stakeholders in industry.
Statistical Methods
Statistical methods are fundamental in the analysis of three-dimensional measurements. They provide the framework through which researchers can ensure that data is interpreted correctly and that findings are reliable.
By utilizing statistical techniques, researchers can perform tasks such as:
- Descriptive Statistics: These methods summarize the main characteristics of data through measures such as mean, median, and mode. They provide insights into the general trends and patterns in the measurements.
- Inferential Statistics: This branch helps researchers make predictions and generalizations about a population based on a sample. Techniques like regression analysis can identify relationships between different variables captured in the measurements.
- Hypothesis Testing: Statistical hypothesis testing can be used to validate or refute assumptions about the data. This is essential in determining the significance of the findings in scientific inquiries.
Familiarity with these statistical methods allows researchers to handle three-dimensional data with professionalism and rigor. Using software like R or SPSS can streamline the analysis, enabling quicker processing of complex datasets.
Visualization Techniques
Visualization techniques are paramount in transforming raw data into a format that is easy to comprehend and analyze. In three-dimensional measurements, effective visualization not only aids in the interpretation but enhances communication of findings.
There are several techniques commonly used in this domain:
- 3D Graphing: Tools such as MATLAB and Python libraries like Matplotlib can create three-dimensional graphs that allow researchers to observe relationships between multiple variables simultaneously.
- Heat Maps: These graphical representations show data values across two dimensions in color. In 3D measurements, they can be particularly useful for displaying variations in density or intensity.
- Volume Rendering: This technique is utilized in fields like medical imaging. It allows for the visualization of three-dimensional structures, enabling a more thorough analysis of spatial relationships.
"Effective visualization is crucial; it turns complex data into insightful narratives for stakeholders, enhancing clarity and understanding."
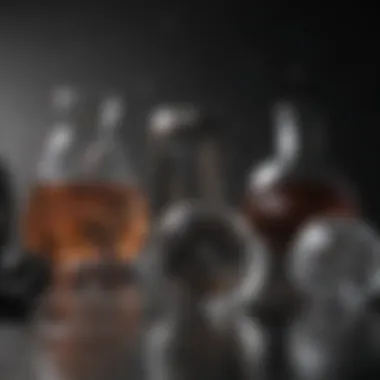
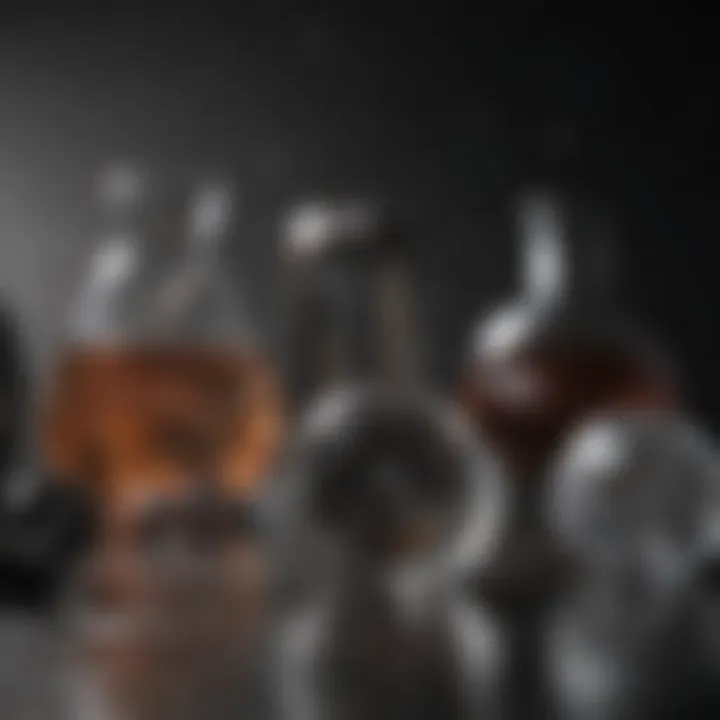
In summary, the combination of robust statistical methods with advanced visualization techniques equips researchers to delve deeper into their analyses of three-dimensional measurements, leading to informed decisions and new discoveries.
Challenges in Three-Dimensional Measurements
The area of three-dimensional measurements is not without its challenges. Identifying these challenges is crucial for researchers and practitioners who rely on these measurements for accurate data and innovation. Understanding the specific issues aids in better planning and implementation of measurement strategies, ultimately improving the quality of scientific research.
Accuracy and Precision Issues
Accuracy refers to how close a measurement is to the true value, while precision indicates the repeatability of measurements. In three-dimensional measurements, achieving both can be quite challenging. Variations in measurement tools and methods can lead to discrepancies, affecting the reliability of the data collected. For example, small errors in manual measurements can accumulate, producing significant impact on the analysis outcome.
Factors that contribute to accuracy and precision include:
- Instrument Calibration: Regular calibration of instruments is necessary to ensure that they provide correct data.
- Environmental Conditions: Changes in temperature, humidity, or pressure can alter measurements.
- Operator Error: Human factors, such as technique inconsistency, can introduce errors.
- Data Processing: Issues in data handling can also lead to inaccuracies.
Improving these aspects may involve employing advanced measurement techniques, such as laser scanning or photogrammetry, that can minimize errors and enhance both accuracy and precision.
Technological Limitations
While technology has advanced significantly, certain limitations still affect three-dimensional measurements. The instruments used may have inherent constraints that impact the measurement process. One primary issue is the depth resolution, which refers to the ability to differentiate between multiple layers in dense materials. This can be particularly challenging in fields such as geology and biology.
Other technological limitations include:
- Resolution Limits: Devices may struggle to capture fine details depending on their specific capabilities.
- Cost of Equipment: Advanced tools like 3D scanners can be prohibitively expensive, limiting accessibility for smaller labs and institutions.
- Software Compatibility: Not all measurement tools are compatible with analysis software, which can hinder workflow efficiency.
Future research may aim to bridge these technological gaps by developing more affordable and versatile tools, thus enhancing the reliability of three-dimensional measurements across various scientific disciplines.
The challenges faced in three-dimensional measurements highlight the need for ongoing research and improvements in both methodology and technology.
Ultimately, addressing these challenges is essential for ensuring that three-dimensional measurements in scientific research are both accurate and meaningful. By acknowledging these issues, researchers can develop strategies to mitigate them, leading to robust scientific outcomes.
Future Directions
Understanding the future directions of three-dimensional measurements is crucial for enhancing scientific research. This section explores how advancements in technology and methodology can influence research practices across various disciplines. By recognizing emerging trends and potential research areas, scientists can adapt and improve their measurement techniques, ensuring that they remain relevant in a fast-evolving landscape.
Emerging Trends
One of the noticeable trends in three-dimensional measurements is the integration of artificial intelligence. AI algorithms are increasingly being used to analyze complex datasets, allowing researchers to uncover insights that were previously difficult to detect. For instance, machine learning models can improve the accuracy of measurements by identifying subtle patterns in the data. This trend not only increases precision but also reduces the time required for analysis.
Additionally, there is a move towards more automation in measurement processes. Automated systems can conduct measurements more quickly and consistently than manual methods, minimizing human error. The rise of drones in environmental research exemplifies this trend. Drones can capture 3D spatial data over large areas, enabling researchers to gather information efficiently.
Finally, collaboration across disciplines is becoming more common. Innovations in one area, such as robotics, can influence measurement techniques in another field, such as healthcare. Interdisciplinary collaborations can lead to the development of new tools and methodologies that enhance the quality of three-dimensional research.
Potential Research Areas
Several promising research areas are emerging within the realm of three-dimensional measurements. One significant area is the study of materials at the nanoscale. Investigating how materials behave in three dimensions at such small scales can lead to breakthroughs in nanotechnology and materials science. This requires the development of advanced measurement techniques to observe structures that are not visible through conventional methods.
Another area is the application of three-dimensional measurements in personalized medicine. With the increasing availability of 3D imaging technologies, researchers can develop tailored treatments based on the unique anatomical features of patients. This research aims to improve surgical outcomes and treatment efficacy.
Moreover, understanding the impacts of climate change through three-dimensional measurements of ecosystems is crucial. Researchers can utilize 3D modeling to simulate environmental changes and predict their effects on biodiversity. This could support the development of strategies for conservation and sustainable resource management.
Closure
The conclusion of this article encapsulates the critical role that three-dimensional measurements play in various scientific fields. Understanding these measurements enhances the quality and accuracy of research. This section will reflect on specific insights gathered throughout the article, emphasizing their relevance and implications for future endeavors in science and technology.
Summary of Key Insights
Several key insights emerge from our exploration of three-dimensional measurements:
- Importance of Precision: Accurate measurements are fundamental to scientific inquiry. They allow researchers to validate their hypotheses and enhance the reliability of their findings.
- Diverse Applications: The versatility of 3D measurements is evident in applications ranging from healthcare to engineering. Each discipline encounters unique challenges and leverages innovative tools tailored to their needs.
- Technological Integration: Advances in imaging and data analysis technologies are reshaping the landscape of 3D measurements. Software integration facilitates the seamless transition from data collection to interpretation, enhancing research outputs.
- Challenges Remain: Despite progress, issues such as accuracy, precision, and technological limitations persist. Continuous evaluation and adaptation are necessary to address these challenges.
The Importance of Continuous Learning
In a rapidly evolving scientific environment, the importance of continuous learning cannot be overstressed. The field of three-dimensional measurements is dynamic, with constant technological advancements and methodological shifts. This necessitates a commitment to ongoing education among researchers, practitioners, and educators.
- Keeping Knowledge Current: Workshops, seminars, and online courses can play a vital role in ensuring that scientists are familiar with the latest tools and techniques in their fields.
- Adaptability: Learning continuously cultivates a mindset ready to embrace change, whether it involves adopting new measurement instruments or utilizing advanced data analysis methods.
- Collaboration and Networking: Engaging with others in the field can lead to shared knowledge and experiences. These interactions underscore the importance of collaboration in enhancing research quality.
Continuous learning fosters the ability to adapt and innovate, which is essential in a competitive scientific landscape.
In summary, the conclusion of this article underscores the essential insights of three-dimensional measurements, emphasizing their significance across various domains. It advocates for a culture of continuous education to navigate the challenges and leverage the opportunities presented by this evolving field.