Exploring Thin Film Thickness Measurement Techniques
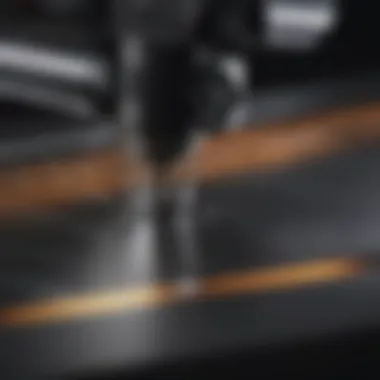
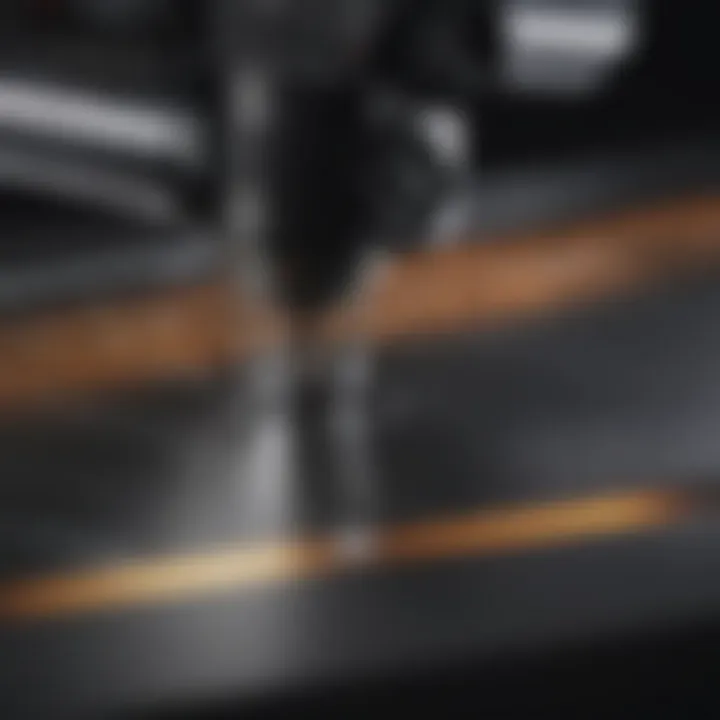
Intro
The measurement of thin film thickness is pivotal in a range of scientific and engineering fields. Thin films are utilized in numerous applications, from semiconductor manufacturing to optics and coatings. As the demand for precision increases, understanding the various techniques for measuring thin film thickness becomes essential. This article will explore the intricacies of different measurement techniques, each with unique principles, benefits, and constraints. By thoroughly analyzing optical, mechanical, and electrical methods, practitioners and researchers will gain insight into the most effective approaches for accurate thin film characterization.
Research Overview
Summary of Key Findings
Relevance to Current Scientific Discussions
Understanding thin film thickness measurement is increasingly relevant in today’s technology-driven world. As industries push for thinner films in electronic devices, optimizing measurement techniques can lead to more innovative applications. The advancements in these measurement technologies often inform material science discussions regarding the properties and potential uses of compounds at the nanoscale.
Methodology
Research Design and Approach
This article synthesizes information from academic literature, technical reports, and industry practices. The emphasis is on comparative analysis of different techniques, assessing how each can be implemented in real-world scenarios. Evaluation criteria include accuracy, convenience, and potential for automation.
Data Collection and Analysis Techniques
Data was collected through a comprehensive review of peer-reviewed articles and case studies available in databases such as JSTOR and IEEE Xplore. Multiple sources were analyzed to ensure a balanced understanding of each measurement method's performance. This methodology aids in presenting an objective overview of available techniques, making it valuable for researchers who seek the best practices in thin film measurement.
Prologue to Thin Film Measurements
Thin film thickness measurement is a fundamental component across various fields, including materials science, optics, and engineering. Understanding how to accurately measure the thickness of thin films is essential for improving product performance, ensuring quality control, and advancing technological development. As films become increasingly integral in devices, processes, and applications, the need for precise measurements grows ever more pressing.
Definition of Thin Films
Thin films are layers of material that typically range from fractions of a nanometer to several micrometers in thickness. They can be made from a variety of materials, including metals, semiconductors, and insulators. The unique properties of thin films arise from their reduced dimensionality, which can lead to different mechanical, electrical, and optical characteristics when compared to bulk materials. This altered behavior makes them particularly useful in applications such as coatings, microelectronics, and sensors.
Importance of Thin Film Thickness Measurement
The accurate measurement of thin film thickness is crucial for several reasons:
- Quality Assurance: Precise thickness ensures that materials will perform as expected in their intended applications. For instance, in semiconductor manufacturing, incorrect thickness can lead to device failure.
- Performance Optimization: Thin films can exhibit varying properties when their thickness changes. Understanding these variations helps in optimizing device performance, such as in photovoltaic cells or optical coatings.
- Research and Development: In scientific studies, reliable thickness measurements facilitate the development of new materials and technologies. For academic researchers, accurate data is necessary to validate experiments and produce repeatable results.
Accurate measurement of thin film thickness helps researchers to precisely tailor the material properties for specific applications, directly impacting performance and durability.
Fundamental Principles of Measurement Techniques
The measurement of thin film thickness is guided by several fundamental principles that form the backbone of various techniques. Understanding these principles is essential for selecting the appropriate measurement method. These considerations directly impact the accuracy, repeatability, and applicability of measurements in practical scenarios. Each method's performance is influenced by its unique combination of geometric configurations and material characteristics.
Geometric Considerations
Geometric factors play a vital role in the effectiveness of thin film thickness measurement. The angle of incidence, light path, and detection configuration can significantly affect the measurement results.
- Angle of Incidence: The incidence angle can alter the intensity of reflected light and its phase change.
- Light Path Length: A longer light path can lead to greater dispersion effects, which may influence accuracy.
- Measurement Configuration: The choice of measurement setup must align with the sample’s geometry. A flat surface will respond differently compared to a curved one. These elements collectively determine how well the measurement technique can resolve thickness variations in thin film structures.
Developing a nuanced understanding of these factors allows for better optimization of measurement techniques to suit specific applications. In addition, designers of measurement systems must consider these geometric aspects to mitigate potential sources of error.
Material Properties and Their Impact
The inherent properties of the materials being measured are crucial in assessing the effectiveness of any measurement technique. Thin films can be made from various materials, each with distinct attributes that influence the measurement process.
- Refractive Index: Knowledge of the film’s refractive index is critical for techniques like ellipsometry and reflectometry. Variations in refractive index lead to differing interference patterns which must be accounted for.
- Absorption Characteristics: Some materials may absorb specific wavelengths of light more than others. This absorption can affect the signal used for measurement, leading to less accurate results.
- Mechanical Properties: The stress and structural integrity of the film can alter its effective thickness when subjected to various environmental conditions.
Understanding how these properties interact with measurement techniques informs the choice of method and enhances accuracy in measurements. Consequently, practitioners must assess not only the thickness but also the underlying material characteristics to achieve reliable results.
Effective thickness measurements begin with a thorough understanding of geometric configurations and material properties. Without this knowledge, achieving accuracy remains a difficult endeavor.
Optical Measurement Techniques
In the realm of thin film thickness measurement, optical measurement techniques hold a pivotal position due to their non-invasive nature and ability to provide rapid results. These methods leverage principles of light behavior, making them ideal for a variety of applications, from semiconductor manufacturing to the study of complex coatings. The significance of optical techniques stems from their capacity to deliver high precision and accuracy in real-time, which is essential in research and industrial settings where material properties can directly influence performance and reliability.
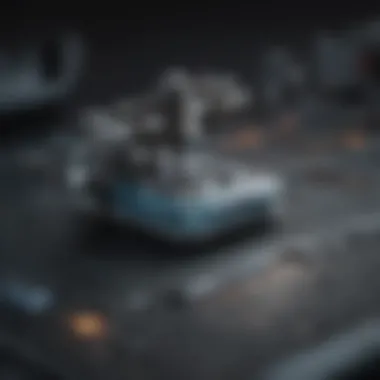
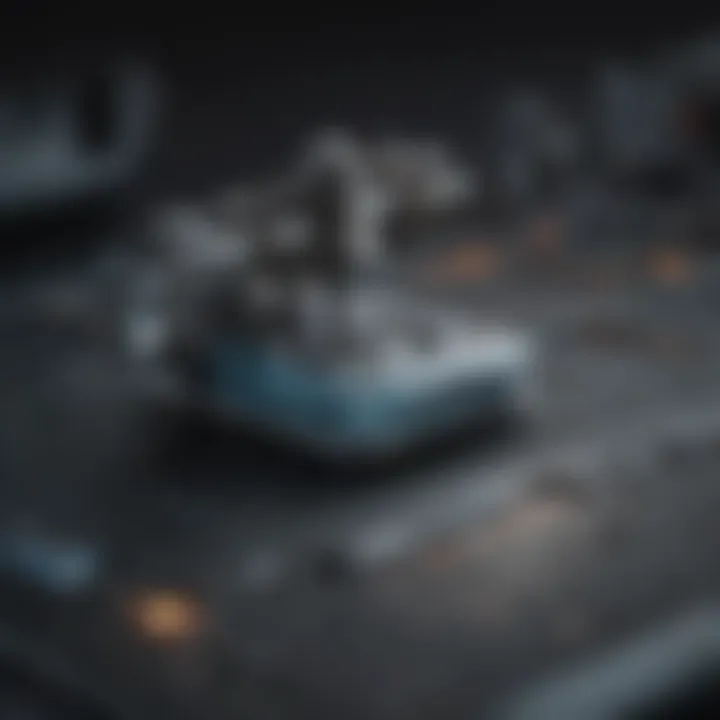
Interference Methods
Interference methods are among the most established optical techniques for measuring thin film thickness. These methods exploit the phenomenon where light waves combine, producing an interference pattern. When light reflects off the top and bottom interfaces of a thin film, the resultant waves can constructively or destructively interfere based on the film's thickness and refractive index.
One commonly used interference method is white light interferometry. In this approach, a broad spectrum of wavelengths is used to determine the film thickness with high accuracy. The major advantages include:
- High Resolution: Can measure thickness with nanometer precision.
- Non-Destructive Testing: Maintains the integrity of the sample being measured.
However, there are considerations to take into account with these methods. The surface roughness of the film can affect results. Moreover, films with very different refractive indices may complicate the interpretation of interference patterns.
Ellipsometry
Ellipsometry stands out as a powerful optical measurement technique, renowned for its capability to measure film thickness and optical properties simultaneously. It evaluates the change in polarization as light reflects off a thin film. Through this analysis, ellipsometers can extract detailed information about the film's thickness and refractive index.
Key benefits of ellipsometry include:
- Sensitivity: Offers remarkable sensitivity to thickness variations, as small as a few angstroms.
- Wide Applicability: Useful for various materials, including semiconductors, polymers, and metals.
Nevertheless, the technique demands precise calibration and knowledge of the optical properties of the film to provide accurate results. This can pose a challenge when dealing with complex layered structures or heterogeneous films.
Reflectometry
Reflectometry is another significant optical technique for thin film measurement. This method analyzes the reflected light intensity as a function of wavelength or angle. Time-domain and frequency-domain reflectometry are two notable variations of this method.
- Time-Domain Reflectometry: Features as a rapid method, suitable for varying thicknesses across a surface. It utilizes pulsed light to evaluate reflections over time, making it effective for sophisticated layered systems.
- Frequency-Domain Reflectometry: Offers finer resolution in wavelength selection, enabling it to discern minute changes in film thickness.
The primary advantages of reflectometry include:
- Speed: Measurements can be completed quickly, which is crucial in production environments.
- Versatility: Applicable to both homogeneous and inhomogeneous films.
However, it can also suffer from limitations related to the angular dependence of reflections and potential interference effects in multilayer structures. Careful analysis and modeling are essential for accurate data interpretation.
Mechanical Measurement Techniques
Mechanical measurement techniques play a vital role in thin film thickness measurement. These methods are instrumental in providing precise measurements required in various applications, such as microelectronics and material science. Mechanical techniques operate by physically interacting with the thin film surface, allowing precise quantification of its thickness. Understanding these techniques is crucial for scientists and engineers because they can directly affect the quality and performance of devices.
One of the key benefits of mechanical measurement techniques lies in their ability to deliver high-resolution data. When employed correctly, these methods can obtain accuracy levels that may outperform some other techniques. This precision is particularly important in fields where even slight variations in film thickness can impact performance. However, users must also consider the trade-offs in terms of time and complexity involved in setting up these measurements.
These techniques generally fall into two categories: profilometry and contact/non-contact methods. Each approach holds distinct benefits and applications, ensuring that various industry needs are met.
Profilometry
Profilometry is one of the primary mechanical measurement techniques utilized for quantifying thin film thickness. It involves scanning the surface of the thin film to generate a topographic profile. The technique applies a stylus that moves across the film surface, recording height variations. This data is then processed to calculate the film thickness accurately.
The principal advantage of profilometry is its ability to provide detailed surface information, not just thickness. Moreover, it is particularly effective for films with complex topographies. The resolution achieved can be very high, making it suitable for research and applications demanding precision. However, the contact nature of the stylus may introduce some issues, such as damage to delicate films or substrate materials. Therefore, careful consideration must be given to the choice of tips and measurement points.
Profilometry also needs calibration against known standards to ensure the precision of measurements. This requirement means additional steps in the measurement process, which can be a drawback in some scenarios. Yet, it remains a popular choice among many researchers dealing with thin films.
Contact and Non-contact Methods
Mechanical measurement methods can further be categorized into contact and non-contact techniques.
Contact methods involve physically touching the surface of the thin film, providing measurements based on mechanical interactions. Typical contact methods include stylus profilometry and mechanical calipers. They offer high accuracy and are often easy to implement. However, the downside is the potential to damage sensitive films or alter their properties during measurement. Sometimes, the presence of contaminants on the surface can also impact accuracy.
In contrast, non-contact methods employ techniques that do not physically touch the film surface. These can include laser scanning and optical methods. Non-contact measurement is especially advantageous for fragile films, minimizing the risk of damage. Additionally, these methods often have faster measurement cycles, which enhances productivity in a laboratory or production environment. However, non-contact techniques may have limitations in resolution or may require careful alignment to ensure accuracy.
Choosing between contact and non-contact methods hinges on the specific application and the required level of precision. Determining the nature of the thin film and the environment also influence this decision. The balance between risk, speed, and accuracy must be carefully evaluated to achieve the best measurement outcomes.
Electrical Measurement Techniques
Electrical measurement techniques play a significant role in the measurement of thin film thickness. These methods are particularly useful because they often provide non-destructive ways to obtain data about material properties. The implementation of electrical techniques can simplify the measurement process and enhance accuracy in specific applications. Notably, both capacitance and resistance measurements offer valuable insights into the characteristics of thin films, making them essential for various industries including electronics.
Capacitance Measurements
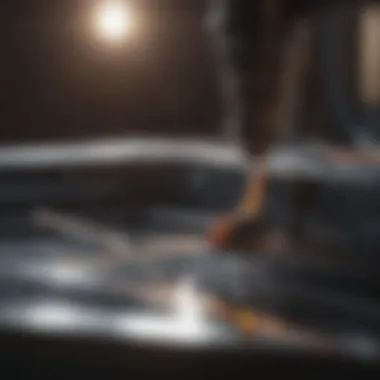
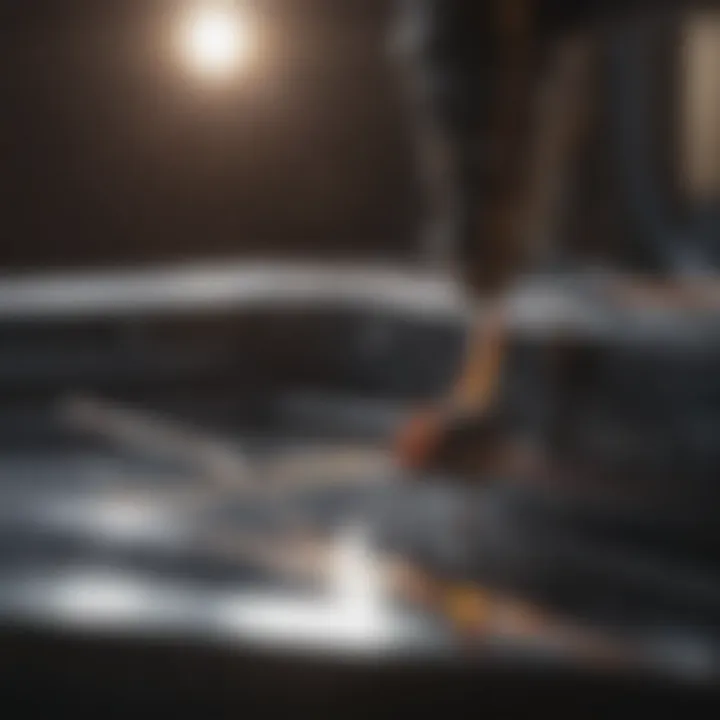
Capacitance measurement is a powerful technique used for determining the thickness of thin films. This method involves the measurement of electrical capacitance between two conductive plates that are separated by a dielectric layer, in this case, the thin film.
The basic principle relies on the relationship between capacitance, area, dielectric constant, and thickness:
[ C = \frac\varepsilon Ad ]
Where:
- ( C ) is the capacitance
- ( \varepsilon ) is the dielectric constant of the material
- ( A ) is the area of the conductive plates
- ( d ) is the thickness of the dielectric layer (thin film)
By measuring the capacitance and knowing the dielectric properties of the film, it is possible to calculate the thickness. The method is not only straightforward, but it also offers high precision.
Some benefits of capacitance measurements include:
- Non-destructive nature, preserving the material integrity
- Capability to measure in-situ, allowing for real-time monitoring
- High sensitivity to thickness changes, especially useful in thin films
However, certain considerations must be taken into account:
- Variations in dielectric constant with temperature can influence results.
- Surface contaminants may affect the measurements, necessitating clean environments.
Resistance Measurements
Resistance measurement is another fundamental technique for assessing thin film thickness. This method operates on the principle that the electrical resistance of a material is related to its dimensions and resistivity.
The relationship can be expressed by the formula:
[ R = \frac\rho LA ]
Where:
- ( R ) is the resistance
- ( \rho ) is the material's resistivity
- ( L ) is the length of the conductive path
- ( A ) is the cross-sectional area
In the context of thin films, we can relate the thickness with resistance, as resistance can change significantly with variations in film thickness. This relationship allows researchers and engineers to infer the thickness of a thin film by measuring its resistance.
Advantages of resistance measurements include:
- Simplicity in setup and operation, making it accessible in many labs.
- Ability to characterise multiple layers of films by examining different resistive properties.
Nonetheless, this approach has limitations as well:
- It requires careful calibration and knowledge of the material’s resistivity.
- Environmental factors, such as temperature and humidity, can significantly impact device performance.
"Electrical measurement techniques offer unique insights into material properties that are essential for scientific advancement and industrial application."
Recent Advancements in Measurement Technology
Advancements in measurement technology play a crucial role in enhancing our understanding of thin film thickness and its implications across various fields. Historically, traditional measurement techniques have served well, but as the complexity and precision demanded by modern applications increase, so does the need for innovative solutions. This section will delve into how recent technologies, particularly nanotechnology and sensor integration, are shaping the future of thin film thickness measurement.
Nanotechnology Influence
Nanotechnology is revolutionizing the way we approach thin film thickness measurement. At the nanoscale, films exhibit unique properties that differ significantly from their bulk counterparts. The precise control at this scale necessitates new measurement methods that can accurately characterize these materials.
One significant advancement includes the development of atomic force microscopy (AFM), which provides high-resolution measurements down to a few nanometers. The capability of AFM to map the topography of thin films enables researchers to observe structural changes that occur at microscopic levels.
"Nanotechnology not only enhances measurement precision but also opens avenues for new applications in fields like electronics and photonics."
Another notable impact of nanotechnology is the advancement of optical measurement methods. For instance, techniques like scanning near-field optical microscopy (SNOM) allow for thickness measurements that are less invasive and more accurate than traditional optical methods.
Integration with Sensor Technologies
The integration of sensor technologies with measurement techniques marks another significant breakthrough. Sensors can provide real-time data, improving the accuracy and efficiency of thin film thickness measurements. Modern sensor technologies, such as piezoresistive and piezoelectric sensors, enable the detection of minute changes in thickness, offering precise feedback during the film deposition process.
These integrated systems can automate measurement processes, leading to enhanced reproducibility and faster data collection. For instance, systems utilizing optical sensors combined with machine learning algorithms can predict thickness variations, allowing for dynamic adjustments during production.
Furthermore, the use of Internet of Things (IoT) technology in measurements allows for remote monitoring and data analysis. This capability facilitates constant optimization and refinement of thin film applications across sectors like semiconductor manufacturing and coatings.
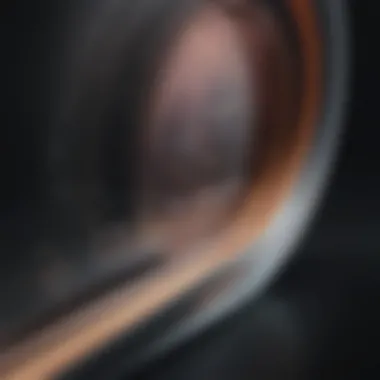
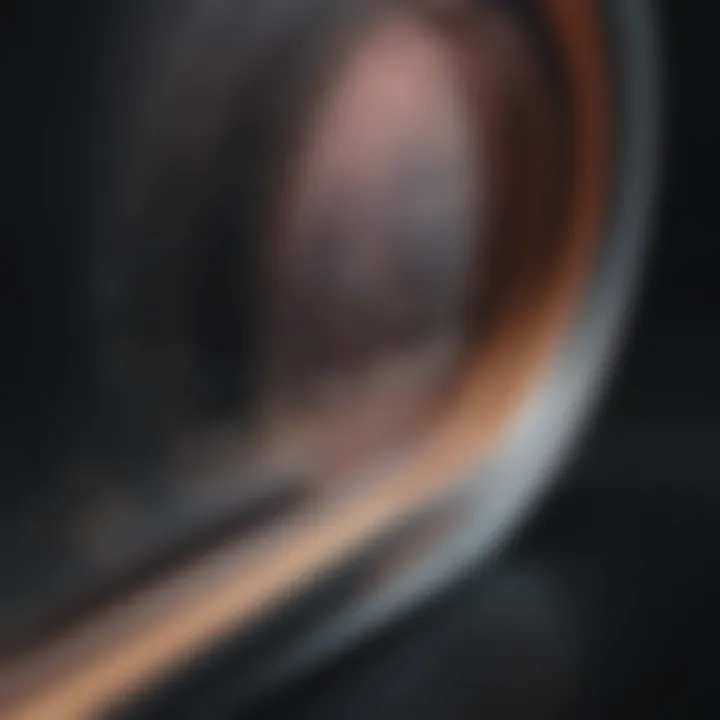
Limitations of Current Techniques
Measurement Uncertainty
Measurement uncertainty is one of the most significant limitations faced in thin film thickness measurement. It refers to the doubt that exists about the result of any measurement. Factors contributing to measurement uncertainty can be numerous and may include:
- Instrumental Errors: Calibration issues or inherent inaccuracies in measurement devices can lead to false readings.
- Environmental Conditions: Fluctuations in temperature, humidity, and light can affect optical measurements, leading to discrepancies.
- Film Homogeneity: Variability in the thin film's properties, such as thickness uniformity and refractive index, can alter the output of measurements, increasing uncertainty.
- Operator Variability: Different skills and techniques applied by various operators can introduce inconsistencies.
Quantifying this uncertainty is crucial as it can substantially impact the reliability of measurements. A deeper understanding of these factors would help in designing better experiments and interpreting results with a critical eye.
Material Constraints
Material constraints present another significant obstacle in thin film thickness measurement. Different materials exhibit unique properties that can affect the choice and effectiveness of measurement techniques. Key considerations include:
- Surface Roughness: The texture of the thin film surface can influence measurement accuracy. Rough surfaces may scatter light, complicating optical measurement methods.
- Refractive Index Variability: Changes in the refractive index due to composition or environmental factors can distort the results for techniques like ellipsometry.
- Chemical Stability: Chemicals used in measurement processes may react with the thin film materials, altering thickness and causing measurement errors.
- Thickness Range: Some methods are ineffective at measuring films that are either too thin or too thick, leading to a lack of suitable techniques for particular applications.
The selection of an appropriate measurement technique thus necessitates an understanding of the specific material properties involved. A comprehensive grasp of these constraints allows for more accurate measurements and better outcomes in applications where precision is crucial.
"As technology progresses, so does the complexity of materials and measurement techniques. A clear recognition of limits encourages innovation in measurement technologies, leading to more accurate results."
Applications in Various Fields
Microelectronics
In microelectronics, thin films are integral to the fabrication of devices like integrated circuits and sensors. Here, the precision of thickness measurement can significantly impact performance. For example, semiconductor layers must be measured accurately to ensure they meet specific electrical and thermal properties. Moreover, adherence to stringent tolerances is vital for device yield rates, which directly affect production costs. Additionally, the continual scaling down of devices has increased the demand for advanced measurement techniques, enabling the reliable control of materials at the nanoscale.
Factors like deposition methods and material properties must be considered because they influence film formation and stability. As devices become more complex, the interplay between various layers makes accurate measurements even more crucial. Effective thickness measurements can also assist in enhancing device efficiency and longevity, resulting in great benefits for the end-users.
Optics and Photonics
Optics and photonics applications require precise thin film characterization for optical components such as coatings on lenses, mirrors, and filters. The performance of devices like lasers, fiber optics, and optical sensors directly relies on film thickness. In this field, measurement demands are high, as even minor discrepancies can affect light transmission and reflection properties. For example, antireflective coatings must be precisely tuned to specific wavelengths of light to minimize losses while maximizing efficiency.
Furthermore, understanding the refractive index and its relation to the film thickness enhances the design and functionality of layered optical structures. Successful measurement techniques can lead to innovative solutions in imaging systems, telecommunications, and many cutting-edge technologies.
Coatings and Tribology
In coatings and tribology, thin films are applied to enhance surface properties of materials, such as wear resistance, corrosion resistance, and friction reduction. Accurate measurement of these films is crucial in applications involved in mechanical engineering and materials science. For instance, in the automotive industry, coatings on engine components can improve performance and longevity, reducing maintenance costs.
The examination of thickness is particularly relevant when evaluating the efficacy of lubrication systems and surface interactions. Furthermore, the tribological performance can be optimized by controlling the thickness of the applied coatings, ensuring that they meet specific requirements under varying operational conditions. This aspect emphasizes the link between accurate measurement techniques and the overall effectiveness of advanced coatings in real-world applications.
Accurate thin film thickness measurements are essential for ensuring functionality in technology and materials sciences. Miscalculations could lead to failures or inefficiencies in systems that rely on these measurements.
Future Directions in Thickness Measurement
Thin film thickness measurement plays a critical role in many fields, including semiconductor manufacturing, optics, and materials science. As technology advances, so does the need for more accurate and efficient measurement techniques. Future directions in this area are likely to focus on enhancing precision and reliability while addressing the limitations presented by current methodologies. This section explores emerging techniques and their implications for measurement accuracy.
Emerging Techniques
One area of growth is the development of novel measurement methods. Techniques such as atomic force microscopy (AFM) and scanning tunneling microscopy (STM) are gaining attention. These methods offer a high level of sensitivity, capable of measuring down to the atomic scale. They can provide detailed insights into film properties and profiles that were previously unattainable with standard techniques.
Another promising direction includes integrating machine learning into measurement processes. By analyzing vast datasets, algorithms can identify patterns and reduce noise in measurements. This integration can lead to improved accuracy and faster processing times, making measurements significantly more efficient.
Technological Impact on Measurement Accuracy
Advancements in sensor technologies are set to impact the accuracy of thickness measurements. The introduction of nanostructured sensors enhances measurement capabilities by providing better sensitivity and stability. These sensors can effectively respond to small changes in film thickness, capturing fluctuations that may impact functionality.
Moreover, the fusion of optical and electronic measurement techniques enhances data gathering. More accurate calibration processes are essential for maintaining precision in measurements. Utilizing multi-sensor approaches improves reliability and minimizes errors, thereby bolstering confidence in measurement data.
"The future of thin film thickness measurement lies in combining precision, efficiency, and innovative technologies to meet the evolving demands of various industries."
As we look to the future, it’s evident that adapting to technological advancements will be crucial. Continued research in emerging techniques and technologies will play a pivotal role in refining measurement accuracy, potentially transforming how thin films are characterized in practice.
Culmination
Understanding thin film thickness measurement is fundamental to the success of various scientific and industrial applications. Thin films are utilized in microelectronics, optics, coatings, and many other fields. Therefore, the precision in measuring their thickness directly impacts the performance and reliability of devices and systems using these films.
Accurate thickness measurements ensure the functionality of the components. For example, in microelectronics, the precise thickness of insulating layers affects the electrical properties of semiconductors. This can influence performance metrics and longevity of electronic devices. In optics and photonics, layer thickness can determine wavelength-dependent behaviors, vital for applications such as filters and mirrors. In coatings, incorrect thickness may lead to decreased durability and compromised performance in environment.
The variety of measurement techniques explored in this article—optical, mechanical, and electrical—each present unique benefits and limitations. Optical methods, such as spectroscopic reflectometry, offer non-destructive options but require careful calibration to account for environmental variables. Mechanical methods, such as profilometry, deliver reliable data but may not be suitable for very delicate films. Electrical techniques, including capacitance and resistance measurements, can provide valuable insights but can also be influenced by the materials used.
Research into emerging technologies continues to advance thickness measurement capabilities. By integrating nanotechnology and advanced sensors, future methods may provide even greater accuracy and efficiency. It’s important for practitioners to be aware of these advances, as they present opportunities for improvement in their respective fields.