Spectrum Scanners: Their Role and Applications Explained
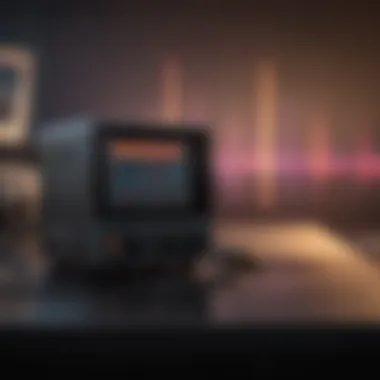
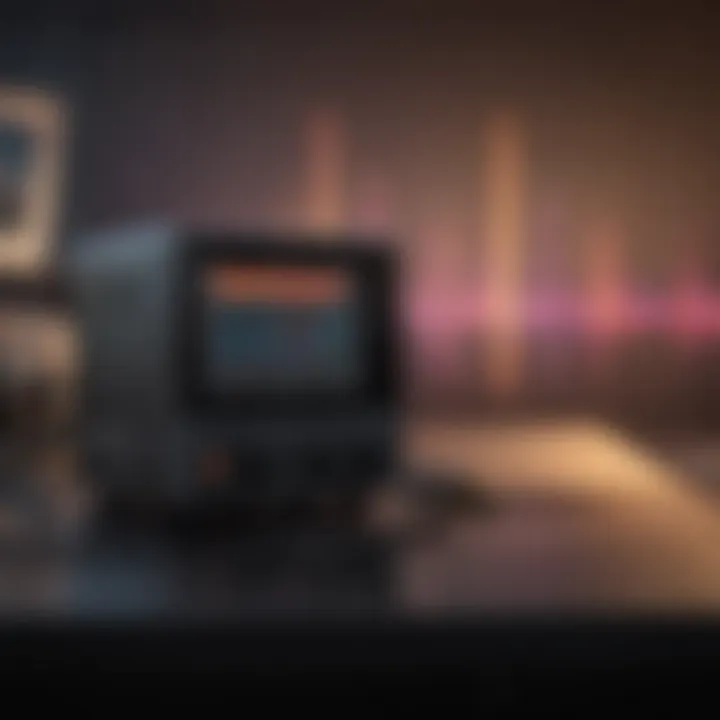
Intro
In the landscape of modern science, spectrum scanners play a pivotal role, bridging various disciplines and offering insights that have reshaped our understanding of the natural world. This technology is not just a fancy gadget; it’s a tool that captures a spectrum of electromagnetic radiation, from radio waves to gamma rays, and translates it into data that scientists can analyze and interpret.
Imagine trying to decode a symphony without being able to hear the orchestra; that’s how critical spectrum scanners are in fields like physics, chemistry, and telecommunications. They allow us to observe phenomena that are otherwise invisible to the naked eye. Whether it’s determining the composition of a distant star or analyzing the quality of a new material, the depth of information these scanners provide is staggering.
Given their versatility, spectrum scanners are woven into the fabric of research today. However, understanding their complexity requires us to first delve into their basic principles before exploring the various applications and implications for future scientific endeavors.
Research Overview
Summary of Key Findings
Through extensive research, it’s become clear that spectrum scanners can be customized for specific scientific explorations. Their applications vary widely,
- Astronomy: Gathering light from stars helps understand cosmic phenomena.
- Environmental Science: Monitoring air or water quality for harmful substances.
- Medical Applications: The analysis of biological samples for disease detection.
These scanners harness principles from spectral analysis, making them indispensable in laboratories and research institutions worldwide.
Relevance to Current Scientific Discussions
In many scientific circles, the discourse surrounding spectrum scanners leans heavily toward their evolving capabilities and ethical usage. As spectrum scanning technology advances, discussions have also sprouted about the implications of its application, particularly concerning privacy issues, especially in communication fields. As we embrace this technology, the pressing question arises: how do we balance innovation with ethical considerations?
As society continues to embrace technologies that push the boundaries, understanding the delicate balance between progress and responsibility becomes crucial for future generations.
Prelims to Spectrum Scanners
Understanding spectrum scanners is crucial for anyone engaged in scientific fields, whether it’s chemistry, biology, or materials science. These devices are instrumental in analyzing and interpreting data from various sources, allowing researchers and professionals to draw insights that could lead to breakthroughs in their respective disciplines. The depth of knowledge one can gain from mastering the principles of spectrum scanners—ranging from their operational mechanisms to their broad applications—can significantly influence the outcomes of complex scientific inquiries.
Importantly, the analysis of spectra sheds light on material composition, molecular interactions, and the characteristics of substances under examination. In this increasingly data-driven scientific environment, the ability to accurately gather and interpret spectral data has become invaluable.
Defining Spectrum Scanners
Spectrum scanners are essentially sophisticated devices designed to measure, analyze, and visualize the spectral properties of light and other forms of electromagnetic radiation. At their core, these scanners take advantage of the principles of spectroscopy, which is based on the interaction of light with matter. Spectra can manifest in various formats, providing insight into the physical and chemical properties of materials.
More technically, spectrum scanners convert light signals into data that can be analyzed and interpreted. For instance, when light is shone on a material, it may absorb specific wavelengths due to the unique electronic structure of its molecules. This results in a spectrum that represents the material's unique identifier. In simple words, just as a fingerprint denotes individuality in humans, the spectrum denotes the individual characteristics of a material.
Historical Background
Jumping back into the pages of history, the development of spectrum scanning technologies has been nothing short of remarkable. The origins trace back to the early 19th century with Joseph von Fraunhofer, who made critical observations regarding the absorption lines in sunlight. His insightful work laid the groundwork for spectroscopy as a scientific discipline.
In the subsequent decades, innovations in optics and instrumentation propelled spectral analysis into a wide range of applications. The advent of more advanced spectrum scanning technology, such as the development of the spectrometer and later the spectrophotometer, expanded analytical capabilities beyond mere observation to quantification and detailed analysis. This revolution transformed diverse fields like astronomy, chemistry, and even healthcare, positioning spectrum scanners as crucial instruments in modern science.
As technology continued to evolve, the integration of computers into spectrum analysis allowed for real-time data processing and visualization. This leap in computational technology raised the bar in how scientists and researchers operated, rendering data interpretation more streamlined and efficient. Looking toward the future, ongoing advancements hint at even broader applications and improved performance in spectrum scanning technologies.
Fundamental Principles of Spectrum Scanning
Understanding the fundamental principles of spectrum scanning is crucial for anyone looking to grasp the wider applications and significance of this technology in various scientific disciplines. At its core, spectrum scanning revolves around the analysis of light and matter interactions. This topic lays the groundwork for all subsequent discussions related to optical phenomena, detection mechanisms, and data interpretation.
Through a blend of physics, chemistry, and even biology, spectrum scanning provides insights that are invaluable in research and development. It opens doors to understanding matter at an atomic level and plays a foundational role in qualitative and quantitative analysis in laboratories worldwide.
Basic Concepts of Spectroscopy
Spectroscopy, in its most basic sense, involves the study of the interaction between matter and electromagnetic radiation. This interaction can unravel a wealth of information about material composition, structure, and environmental conditions. When light strikes a substance, it may either be absorbed or emitted at specific wavelengths, which can be measured and analyzed. The principle is embodied in methods like mass spectrometry, infrared spectroscopy, and ultraviolet-visible spectroscopy. Each of these reveals different aspects of the materials being studied, making spectroscopy a psychological tool for researchers.
Key considerations when diving into spectroscopy include:
- Wavelength: The specific part of the electromagnetic spectrum being analyzed.
- Resolution: The ability to differentiate between close wavelengths.
- Calibration: Ensuring that instruments provide accurate readings.
Types of Spectra
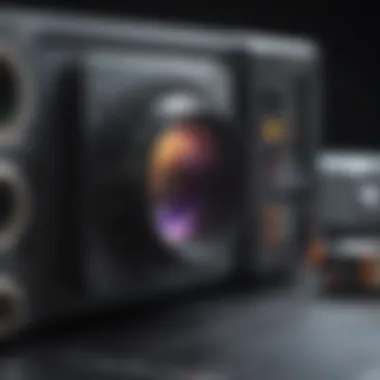
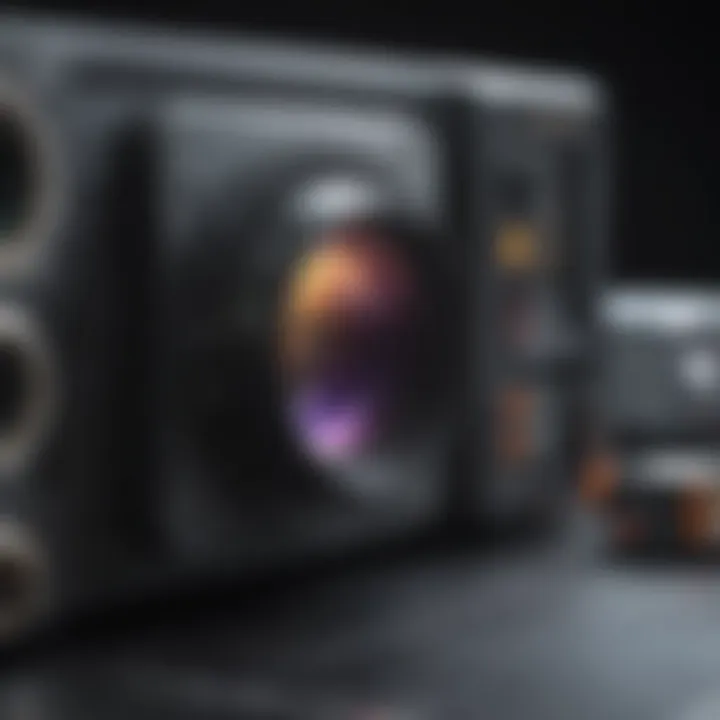
Understanding the different types of spectra is essential for leveraging spectrum scanning effectively. Each spectrum has unique characteristics that provide insights into the matter's physical properties and can lead to varying applications in scientific fields.
Continuous Spectrum
A continuous spectrum showcases a seamless blend of colors, much like a rainbow, representing all wavelengths of light without gaps. This type of spectrum emerges when light is emitted from a solid, liquid, or densely packed gas. It is instrumental in applications such as black body radiation, where it demonstrates how different materials emit light based on their temperature.
The key characteristic of the continuous spectrum is its ability to provide a complete range of visible wavelengths, making it a popular choice in studies focused on thermal emissions. It uniquely allows researchers to discern temperature-related phenomena and has advantages such as the clarity of data representation, though its broadness can complicate isolation of specific spectral lines.
Line Spectrum
In contrast, a line spectrum is characterized by distinct lines at specific wavelengths, each representing emitted or absorbed light by individual elements. Line spectra emerge from gases or atoms at lower densities, where the electron transitions result in quantized energy states.
This type of spectrum stands out due to its sharpness and clarity, making it especially beneficial for identifying elemental composition. For instance, chemists often rely on line spectra for qualitative analysis of components in a mixture, while not being without its drawbacks. The lines can sometimes be influenced by external factors, like pressure or temperature, leading to potential misinterpretations.
Band Spectrum
Finally, a band spectrum arises from molecules, appearing as bands rather than discrete lines due to the vibrations of molecular bonds. This spectrum is prevalent in complex molecules, where the energy levels involved can produce multiple adjacent lines that form broad bands.
The key feature of the band spectrum is its complex multi-transition representation. It serves as an invaluable tool in applications like infrared spectroscopy, as it provides insights into molecular structure and bonding. The advantage here lies in the detailed information regarding molecular interactions, though interpreting band spectra can sometimes prove challenging due to overlapping bands.
In the ever-evolving landscape of scientific research, grasping these fundamental concepts of spectroscopy not only enhances our understanding of the material world but widens the horizon for innovative applications.
In wrapping up, the exploration of these core principles offers a rich foundation for understanding how spectrum scanning serves as a pillar in advanced scientific research, bridging the gap between theory and practice.
Technical Aspects of Spectrum Scanners
Understanding the technical aspects of spectrum scanners is crucial in grasping how they function and are applied in various scientific fields. The efficiency and effectiveness of spectrum scanning largely depend on its components, technologies, and their integrations. Let’s dig into primary elements contributing to the functionality of these scanners.
Components of Spectrum Scanners
Components are the building blocks that define a spectrum scanner’s overall performance. Each part plays a significant role in gathering and interpreting spectral data. The main components include light sources, detectors, and optical instruments.
Light Sources
Light sources are pivotal in a spectrum scanner. They provide the energy necessary for the scanning process. Typically, these sources can be categorized into continuous and discontinuous. Continuous sources, such as incandescent and halogen lamps, provide a broad spectrum of light. They are often favored because they produce stable and high-intensity illumination, ensuring reliable results.
The unique feature of these light sources lies in their ability to cover a wide range of wavelengths, which is essential for many applications. However, one needs to consider that continuous sources can generate excess heat, which may interfere with sensitive measurements.
Detectors
The detector's role is to convert light signals into electrical signals for analysis. The performance of a detector can significantly influence the quality of the spectral data obtained. Different types of detectors, such as photomultiplier tubes and CCD sensors, have their strengths and weaknesses.
Among the detectors, CCDs are particularly valued for their high sensitivity and broad spectral response. They are widely employed in many spectrum scanning applications because of their capability to deliver accurate and reproducible results. However, the complexity and cost associated with CCDs can be limiting factors for some users.
Optical Instruments
Optical instruments are the tools that manage the light pathways within the spectrum scanner. This includes lenses, prisms, and gratings. Each of these components plays a specific role in dispersing and focusing light onto the detectors. For instance, prisms are often used for their ability to separate light into its constituent wavelengths, which is useful in a variety of analytical techniques.
A distinct attribute of these instruments is that they can be fine-tuned to optimize the performance based on the specific spectral analysis being performed. However, improper alignment or inadequate calibration may lead to errors in the collected data.
Applications of Different Technologies
Different technologies employed in spectrum scanning bring distinct benefits and applications to the table, catering to diverse scientific fields. The core technologies span across FTIR, Raman Spectroscopy, and UV-Vis Spectroscopy. Each one harnesses specific principles and techniques to unravel complexities in various materials.
FTIR (Fourier Transform Infrared Spectroscopy)
FTIR is central in molecular identification and quantitative analysis. By measuring the absorption of infrared light, it provides insights into molecular vibrations and rotations. The key characteristic of FTIR lies in its speed and efficiency, requiring only a few seconds for data acquisition compared to older techniques.
One unique feature of FTIR is its ability to analyze samples in various states, including solids, liquids, and gases. However, potential drawbacks include challenges in analyzing complex mixtures, which may result in overlapping spectral peaks that complicate interpretation.
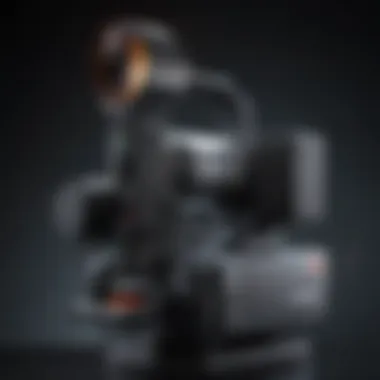
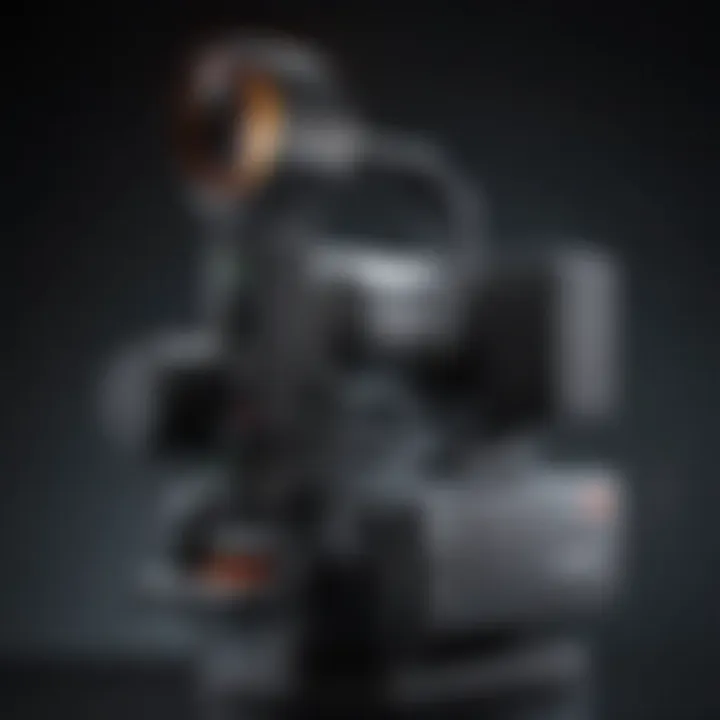
Raman Spectroscopy
Raman Spectroscopy leverages inelastic scattering to provide a unique fingerprint of materials. Unlike FTIR, it focuses on vibrational transitions caused by changes in polarizability, making it particularly useful for characterizing molecular structures. Its non-destructive nature and requirement for minimal sample preparation highlight its advantages.
However, Raman’s sensitivity to fluorescence can sometimes be a drawback, as certain samples may emit light upon excitation, leading to misinterpretation of results.
UV-Vis Spectroscopy
UV-Vis Spectroscopy is renowned for its ability to assess electronic transitions within molecules. It is widely employed in various fields from chemistry to environmental science. The distinguishing characteristic of UV-Vis is its straightforward operation and rapid results.
The unique feature of using UV-Vis lies in its discernment between light absorption at specific wavelengths, which can indicate concentration levels of different substances. Contrarily, it is limited to chromophoric compounds, hence, without such characteristics, the analysis becomes futile.
In summary, mastering the technical aspects of spectrum scanners empowers researchers and scientists to utilize these tools effectively, enhancing their capacity for discovery across diverse scientific domains.
Applications of Spectrum Scanners in Scientific Research
Spectrum scanners are invaluable tools in various scientific disciplines. Their ability to analyze and interpret spectral data allows researchers to understand complex phenomena at a molecular level. This section explores how spectrum scanners contribute to chemistry, biology, environmental sciences, and materials science. By highlighting specific applications and benefits in each field, we can appreciate the broader implications of these technologies.
Role in Chemistry
In chemistry, spectrum scanners play a pivotal role in characterizing substances. Techniques like FTIR and NMR spectroscopy are integral for examining molecular structure and interactions. For example, chemists can use FTIR to identify functional groups in organic compounds. Such analyses help in synthesizing new materials or understanding reaction mechanisms.
The precision of spectrum scanners allows for detailed investigations. Chemists often rely on this technology to monitor phase changes, reaction kinetics, and to confirm the purity of compounds. By obtaining precise spectroscopic data, researchers can tailor chemical processes more effectively.
Role in Biology
Biologists utilize spectrum scanners to delve deeper into cellular processes and biological molecules. Fluorescence spectroscopy, for instance, aids in studying protein interactions and dynamics. This technique, along with Raman spectroscopy, helps scientists quantify and analyze biomolecules with high sensitivity.
Moreover, spectrum scanning is essential in medical diagnostics. It enables rapid identification of pathogens or even cancerous cells through spectral fingerprints, paving the way for early intervention. With such capabilities, biological research and medical applications are closely intertwined, providing promising avenues for understanding life at a molecular level.
Role in Environmental Sciences
The environmental sciences benefit tremendously from spectrum scanners' capacity to detect pollutants in air, water, and soil. For instance, UV-Vis spectroscopy is employed to analyze water samples for trace contaminants. Such analyses are crucial for ensuring safe drinking water and monitoring ecological health.
Furthermore, remote sensing using spectral analysis aids in assessing land use changes and evaluating climate impact. Through satellite data that relies on spectral signatures, scientists can observe deforestation, urban development, and changes in biodiversity. The role of spectrum scanners in environmental research underscores their importance in sustainable practices and policy-making.
Role in Materials Science
In materials science, spectrum scanners are fundamental for characterizing new materials. Techniques like X-ray diffraction and electron microscopy often rely on spectroscopic data to understand material properties at atomic and molecular levels. The insights gained can lead to the development of advanced materials used in electronics, catalysis, and nanotechnology.
By comprehensively analyzing spectral data, researchers can identify defects, crystal structures, and composite materials’ responses to various conditions. This accelerates innovation cycles in material development, enhancing the quality and performance of products in industries ranging from aerospace to renewable energy.
In summary, spectrum scanners are indispensable across various scientific domains. They provide critical information that fuels research and drives advancements, making them essential tools for today’s scientists.
Impact of Spectrum Scanning on Modern Science
Spectrum scanning has proven to be a cornerstone in various scientific disciplines. Its applications stretch far and wide, bringing about advancements that shape our understanding of the physical world.
When looking at the impact of spectrum scanning, several noteworthy elements come to the forefront. Firstly, this technology enhances objectivity in analysis; it transforms qualitative observations into quantitative data. Such precision allows scientists to pinpoint chemical compositions with a level of accuracy that was previously unattainable. These scanners serve as the bridge between complex data sets and interpretable results, streamlining the path from investigation to insight.
Advancements in Diagnosing and Analyzing
In the realm of diagnostics, spectrum scanners contribute significantly to fields like medicine and environmental science. They allow researchers to analyze substances that may be hazardous to health or valuable for treatment. For instance, in disease diagnosis, a technique known as Raman spectroscopy enables non-invasive detection of cancerous cells or pathogens. Earlier methods sometimes had invasive procedures or required complex sample preparations.
Furthermore, these scanners assist environmental scientists in assessing pollutants in soil and water. By interpreting the spectral data, they can identify minute traces of harmful substances, ensuring quicker response times for health advisories and environmental policies.
"The ability to quickly and accurately analyze compounds in natural resources directly influences public health and policy decisions."
By enabling these advancements, spectrum scanning plays a vital role not only in the laboratory but also in society as a whole, as it molds our response to emergencies through timely information.
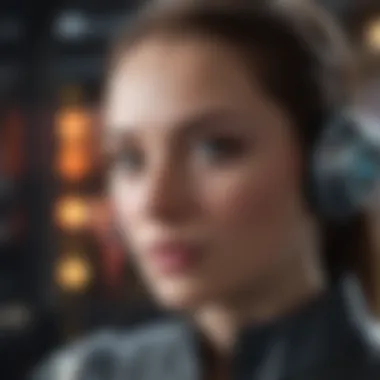
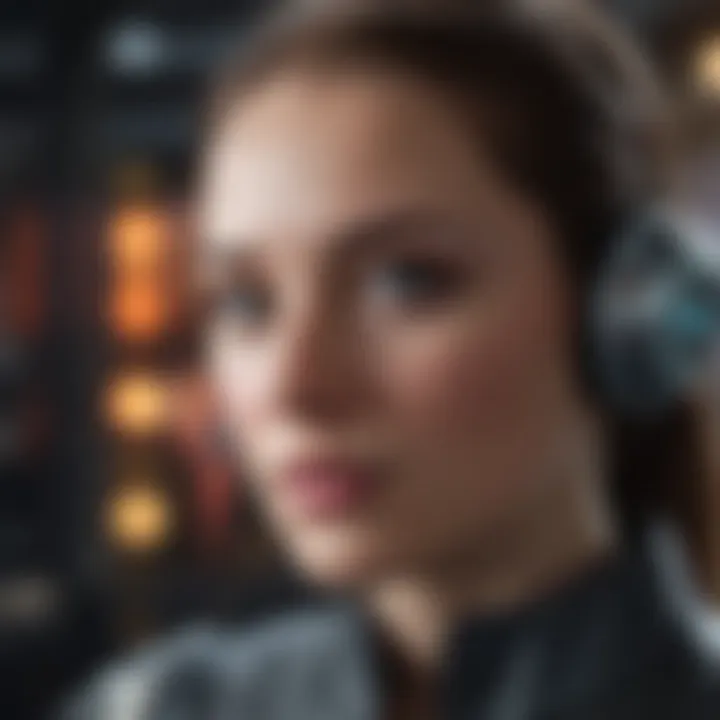
Enhancing Research Methodologies
The methodologies employed in scientific research have been remarkably enhanced through the application of spectrum scanning technologies. The integration of these scanners into research practices encourages a more data-driven approach, leading to more robust and reproducible results.
For example, in materials science, researchers utilize FTIR spectroscopy to analyze the chemical bonds within different compounds. This method provides detailed insight into the molecular structure, paving the way for the development of new materials with specific attributes.
In addition, the continuous feedback from spectrum scanners reshapes experimental designs. This feature is particularly useful in iterative research processes, where ongoing adjustments can be made based on scanner outputs. The adaptability afforded by these technologies not only supports innovation but also fosters a collaborative environment where diverse scientific teams can converge their findings and methodologies.
To sum it up, spectrum scanning isn’t just a tool; it's a dynamic companion in modern scientific endeavors, pushing the boundaries of diagnostics and methodologies further than ever before.
Challenges and Limitations of Spectrum Scanners
Understanding the challenges and limitations inherent to spectrum scanners is pivotal, especially when considering their role in scientific research. These challenges can shape the efficacy with which spectrum scanners operate across different fields. Engaging with the intricacies of these limitations can illuminate pathways for improvement, as well as inform users to make intelligent choices when utilizing these devices.
Technical Limitations
Detector Sensitivity
When we discuss detector sensitivity, we delve into a core attribute that significantly affects the performance of spectrum scanners. High sensitivity detectors can identify minute changes in light intensity, allowing for more precise analysis. This sensitivity is essential in fields like chemistry and biology, where accurate substance identification might hinge on subtle differences in spectral data. However, this high sensitivity comes with a caveat.
A unique feature of sensitive detectors is their susceptibility to noise. This noise can arise from various sources, including electronic interference and environmental factors. Consequently, while sensitive detectors may offer fine resolution, they often require controlled settings to function ideally. Users must weigh the benefits of enhanced detection capabilities against the potential for misleading data due to noise. In doing so, they can maximize the scanner's effectiveness by understanding and mitigating these issues.
Interference Issues
Interference remains a significant hurdle when deploying spectrum scanners. This issue often manifests when external wavelengths disrupt the spectral data being captured. Factors contributing to this interference range from ambient light conditions to variations in sample composition.
The primary characteristic of interference is its unpredictable nature, making it difficult to account for in various applications. Researchers striving for reliable results must consider the unique features of their experimental setups. The presence of interference can lead to reduced signal clarity which is detrimental when precise analysis is critical. Even with advanced filtering techniques, residual interference can skew results, leading to misinterpretation or erroneous conclusions. Thus, the challenge lies in developing strategies to minimize interference while preserving the scanner’s functionality.
Cost-Effectiveness
Cost-effectiveness is a double-edged sword in the landscape of spectrum scanning technologies. On one hand, investing in high-quality spectrum scanners can yield substantial long-term benefits, including better precision and reliability. However, the initial costs of these advanced devices can be prohibitive, particularly for smaller labs or educational institutions. Researchers often find themselves in a balancing act, deciding whether to invest in top-tier technology or opt for basic models that may lack certain functionalities.
The choice of equipment can significantly influence research outcomes. Less expensive models may perform adequately for simple analyses but may fall short when complex data interpretation is necessary. It's vital to approach this decision with a clear understanding of both immediate needs and long-term goals.
Ethical Considerations
The ethical landscape surrounding spectrum scanning cannot be overlooked. The ability to analyze and identify substances accurately raises questions about data handling and privacy. Researchers must navigate the moral implications of their findings, especially when dealing with human tissues or environmental samples that may have far-reaching consequences.
A key consideration is ensuring the integrity of data. Misrepresentation, whether intentional or accidental, can have serious ramifications, influencing policies or even public health responses. Additionally, the deployment of spectrum scanning technology in areas such as forensics or pharmaceutical research necessitates a careful ethical examination. Researchers carry the responsibility of not only conducting their work with integrity but also considering the broader implications their findings may have.
"In the realm of science, understanding the contours of one's tools is as essential to inquiry as the questions we seek to answer."
Addressing these challenges and limitations will ultimately enhance the effectiveness and reliability of spectrum scanners. Through a considered and reflective approach to these issues, researchers can continue to leverage these powerful tools in their quest for knowledge.
The Future of Spectrum Scanning Technologies
As we gaze into the horizon of scientific innovation, the future of spectrum scanning technologies unfolds with both excitement and anticipation. Spectrum scanners have evolved into indispensable tools across various disciplines. Their potential is far from fully tapped, and ongoing research indicates promising advancements that might reshape how we analyze and interpret data.
Emerging Trends in Research
In recent years, several trends have begun to emerge in the realm of spectrum scanning. These trends reflect not just technological evolution but also a deeper integration with other areas of research. Some notable developments include:
- Increased Automation: With machine learning and artificial intelligence gaining ground, the integration of automated systems in spectrum scanning is proving beneficial. Instruments capable of self-calibration and adaptive settings are carving a more efficient path, reducing human error and improving accuracy.
- Miniaturization: Researchers are increasingly keen on compact devices, paving the way for portable spectrum scanners. These devices make it possible for field studies and ensure that spectrum analysis is not confined to laboratories.
- Open-Source Platforms: The open-source movement is permeating the field of spectroscopy. Collaborative platforms allow scientists from various backgrounds to develop and share software tools, pushing the boundaries of what’s achievable in spectrum analysis.
- Data Fusion: Combining data from various spectroscopic techniques can yield richer, multidimensional insights. This approach enhances our comprehension of materials and enables more comprehensive study of complex systems.
The benefits of these trends are manifold. They not only enhance the efficiency and accessibility of spectrum analysis but also catalyze interdisciplinary collaborations, thus fostering a broader understanding of various phenomena.
Potential Innovations
With the advancement of spectrum scanning technologies comes an array of potential innovations set to revolutionize the field. Here are a few concepts that are on the brink of revolutionizing how spectrum scanners function:
- Quantum Dots: The use of quantum dots in enhancing resolution in spectroscopy is gaining traction. Their unique optical properties can help achieve more precise measurements, which could be ground-breaking in fields like biomedicine and nanotechnology.
- Real-Time Spectrum Analysis: The future may also welcome instruments capable of real-time data processing. This capability could transform how we conduct experiments, allowing immediate feedback and adjustments, thereby facilitating dynamic research environments.
- Integration with Cloud Computing: As cloud technologies continue to evolve, the integration of spectrum databases with cloud computing can provide global researchers access to vast datasets, enabling collaborative analysis and shared resources.
- Enhanced User Interfaces: Imagine intuitive user interfaces driven by augmented reality that assist researchers in navigating complex spectral data. This would not only simplify data interpretation but also enhance educational opportunities in spectroscopy.
"As we look to the future, the continuous evolution of spectrum scanning technologies promises to open doors to new realms of scientific inquiry and discovery."
The innovations on the horizon underscore the adaptability and relevance of spectrum scanners within scientific contexts. As these capabilities develop, their applications can potentially stretch into uncharted territories, altering the landscape of scientific research today and well into the future.