Understanding Silicon Wafer Thermal Conductivity
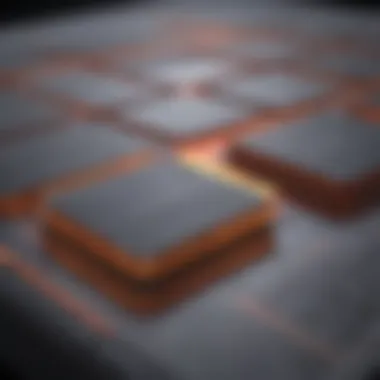
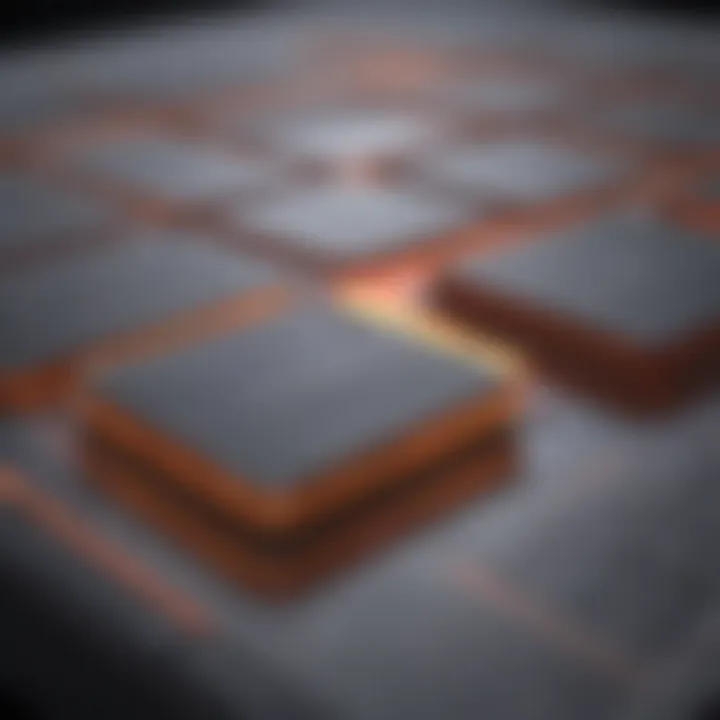
Intro
The thermal conductivity of silicon wafers plays a crucial role in modern semiconductor technology. Understanding how heat moves through these materials is not just an academic exercise; it has profound implications for performance, reliability, and innovation in various applications, from semiconductor devices to solar cells. This area of study is often overlooked, yet it forms the backbone of advancements in electronics and renewable energy technology.
In this exploration, we will navigate through the essential concepts, delve into measurement techniques, and outline the factors that influence thermal conductivity. Gaining insights into these elements is vital for anyone involved in material science or engineering disciplines.
We will also touch on the latest advancements within this domain, particularly how engineers and scientists are enhancing thermal management strategies to optimize device performance. Ultimately, this article invites you to appreciate the intricate dance between heat and technology, highlighting its significance in our rapidly evolving landscape.
Research Overview
Summary of Key Findings
The study of thermal conductivity in silicon wafers reveals several critical findings. Firstly, the inherent properties of silicon make it an ideal candidate for thermal management in electronic devices.
Here are some key takeaways from recent research:
- Thermal Conductivity Values: Silicon has notably high thermal conductivity, typically around 149 W/mK at room temperature. This characteristic allows for effective heat dissipation in high-performance applications.
- Temperature Dependence: Thermal conductivity in silicon wafers decreases at elevated temperatures due to increased phonon scattering, which can limit device efficiency.
- Doping Effects: The introduction of dopants can significantly alter thermal properties, affecting how heat travels within the material. Different doping elements can either enhance or reduce thermal conductivity.
These findings underscore the importance of understanding not only thermal conductivity itself but also the various factors influencing it to enhance the performance of semiconductor devices.
Relevance to Current Scientific Discussions
The subject of thermal conductivity in silicon wafers has relevance in several ongoing discussions in the scientific community. For instance, as the push towards miniaturization of electronic components continues, managing heat efficiently becomes ever more critical.
Additionally, with the growing interest in sustainable energy solutions, particularly solar energy, the need for optimal thermal management in photovoltaic systems cannot be overstated. Recent advancements in material science techniques could provide promising avenues for enhancing silicon’s thermal properties, thereby improving overall system efficiencies.
The challenge of thermal management in electronics has sparked lively debates among students and professionals alike, as they seek innovative solutions to overcome current limitations associated with silicon wafers.
Taking all this into account, one can appreciate how pivotal the study of thermal conductivity is to progress across multiple technological fronts.
Prelude to Silicon Wafer Technology
Understanding silicon wafer technology is paramount to grasping the nuances of many modern electronic devices. At the heart of semiconductor fabrication, silicon wafers serve as the substrate upon which many circuits are constructed. Their properties not only influence the performance of individual components but also impact overall device efficiency and reliability. Given the rapid evolution of technology, particularly in electronics and renewable energy, delving into the essentials of silicon wafers can yield significant insights.
Silicon wafers carry unique characteristics that underscore their significance in the semiconductor industry. They function as the building blocks for numerous applications in devices such as smartphones, laptops, and solar panels. Without a firm foundation like silicon, the staggering advancements in technology we enjoy today would not have been possible. Understanding how these wafers operate, their structure, and their thermal management capabilities can provide a deeper appreciation for their role in innovation.
What is a Silicon Wafer?
A silicon wafer is essentially a thin slice of silicon crystal, typically ranging from a few millimeters to several inches in diameter. These wafers serve as the substrate upon which electronic devices are built. To elaborate, the wafers are polished to achieve a smooth surface before various manufacturing processes are applied.
The manufacturing of silicon wafers involves a highly controlled process. First, high-purity silicon is melted and pulled into a cylindrical ingot using methods like the Czochralski process. Once cooled, the ingot is sliced into wafers of predetermined thickness. This meticulous procedure ensures that the wafers exhibit the right mechanical and electronic properties, making them suitable for further processing in the semiconductor industry.
Importantly, silicon wafers' thermal properties are crucial. They influence how easily heat can traverse the material, which has direct implications for the performance of electronic devices. A solid understanding of these properties can help engineers tackle challenges like overheating and improve design efficiency in future technology.
Historical Context of Silicon Usage
Silicon's journey to becoming a cornerstone of modern electronics is fascinating and speaks to the material's versatility. The use of silicon in electronics began mid-20th century, with the first practical applications appearing in the 1950s. While before that, germanium had been used as the primary semiconductor, silicon soon took the lead largely due to its abundance and superior thermal properties.
By the time the 1970s rolled around, silicon emerged as the material of choice for integrated circuits. This was not by mere happenstance; its atomic structure offers a perfect platform for doping, allowing for the enhancement of electrical conductivity without compromising other essential material properties. Silicon's stability at high temperatures also marked it as a suitable material for devices that operate under various environmental conditions.
Further developments in silicon technology have paved the way for advancements in the semiconductor industry. Innovations such as silicon-on-insulator (SOI) technology have revolutionized how devices are structured and function. Today, silicon remains at the forefront of material science and electronic application, continuing to influence emerging technologies such as photovoltaics and energy-efficient devices, owing much to its unique thermal management capabilities.
Fundamentals of Thermal Conductivity
Understanding the fundamentals of thermal conductivity is pivotal for grasping how silicon wafers function in various applications. This section sheds light on key concepts surrounding thermal conductivity, laying the groundwork for exploring more complex interactions in silicon wafer technology. Thermal conductivity essentially describes how well heat can move through a material. This property plays a significant role in the efficiency and performance of electronic devices and solar cells. It's not merely academic; it's about optimizing performance in real-world applications.
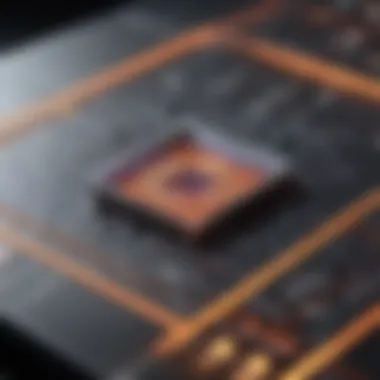
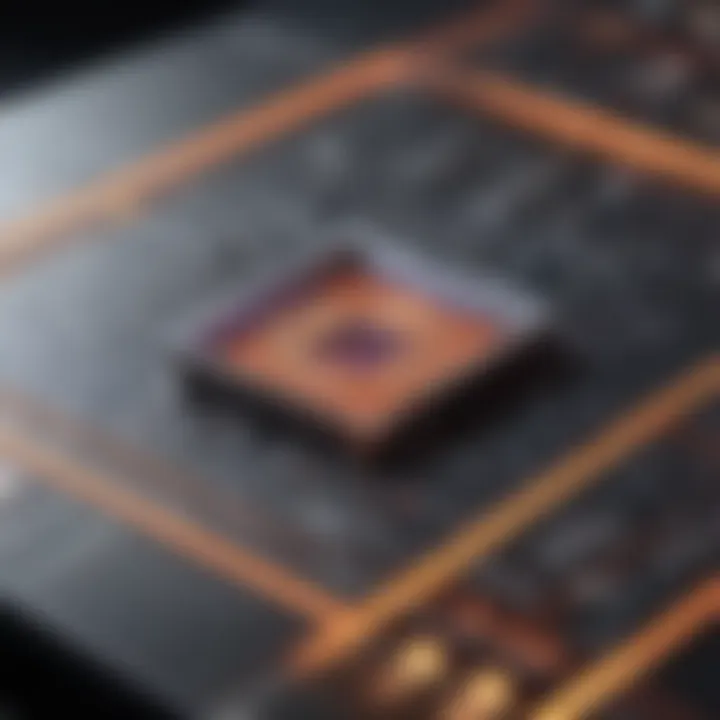
Definition of Thermal Conductivity
Thermal conductivity is the property of a material to conduct heat. In the context of silicon wafers, it refers to the ability of the silicon material to transfer heat from one part to another. Measured in watts per meter-kelvin (W/m·K), a higher value indicates that the material can dissipate heat more effectively. This characteristic is crucial in scenarios where temperature management is essential to prevent overheating and maintain operational reliability. For silicon, the thermal conductivity typically ranges around 150 W/m·K at room temperature, although this figure can vary with purity and temperature.
Mechanisms of Heat Transfer
Thermal transfer can occur via various mechanisms, each playing a unique role in how heat travels through silicon wafers. The three primary modes are conduction, convection, and radiation.
Conduction
Conduction is the process where heat is directly transferred through a material. In silicon wafers, lattice vibrations known as phonons carry thermal energy from hot to cold regions. A key characteristic of conduction is that it requires physical contact; heat doesn't flow through empty space in this method. This makes conduction particularly relevant in densely packed devices like integrated circuits, where components are placed closely together. The efficient conduction in silicon allows for timely cooling of hot spots, making it a favored choice in electronics. However, as temperature rises, the phonon scattering can increase, which in turn may reduce the material's effectiveness to conduct heat.
Convection
Convection occurs in fluids—gases or liquids—when heated particles move and transfer energy. In the context of silicon wafers, convection is less significant compared to conduction but still deserves mention, especially in thermal management systems. Here, the heat transferred by the movement of a surrounding fluid can enhance overall heat dissipation, contributing positively to performance. A critical aspect of convection is the velocity of the fluid; faster-moving fluids can carry heat away more efficiently. In practical terms, measures like cooling fans are often employed to facilitate convection in electronic devices, thereby optimizing their thermal performance.
Radiation
Radiation is the transfer of heat through electromagnetic waves, primarily at higher temperatures. While silicon wafers predominantly rely on conduction and convection, radiation comes into play when dealing with elevated temperatures. A notable characteristic of radiation is that it doesn't require a medium, allowing heat transfer even through a vacuum. This property can be crucial in applications like solar energy systems, where wafers can absorb sunlight and must release heat efficiently to prevent overheating. However, radiation is typically less effective at the temperatures encountered in most silicon wafer applications, therefore not usually relied upon alone for heat management.
Factors Affecting Thermal Conductivity
Various elements influence thermal conductivity, making it a complex but essential aspect to consider in design and application.
Temperature
Temperature itself is a significant factor affecting thermal conductivity. Generally, as temperature increases in silicon, the thermal conductivity decreases due to increased phonon scattering. This quality can pose challenges in applications where maintaining a stable thermal performance is crucial. Understanding the temperature dependence helps in designing devices that can operate efficiently over a range of conditions.
Impurity Levels
The presence of impurities in silicon can significantly alter its thermal conductivity. High purity silicon generally exhibits better thermal conductivity due to fewer defects in its crystal lattice that would scatter the phonons. Conversely, the introduction of impurities can lead to reduced thermal transport efficiency. This relationship emphasizes the importance of materials engineering in optimizing silicon wafers for specific applications, particularly in high-performance electronics.
Crystal Structure
The arrangement of atoms in a crystal structure also influences thermal conductivity. Silicon wafers are usually single-crystal structures, providing superior conductivity compared to polycrystalline forms. The uniform alignment of atoms aids in the efficient transfer of thermal energy, whereas grain boundaries in polycrystalline silicon can hinder the flow of phonons, thus reducing conductivity. Understanding these structural characteristics helps engineers choose the right type of silicon for their thermal management needs.
The detailed investigation into these fundamentals not only enhances the comprehension of silicon wafer applications but also aids in future innovations in semiconductor technology.
Thermal Conductivity in Silicon Wafers
Understanding thermal conductivity in silicon wafers is crucial, especially considering the vital role these components play in the semiconductor industry. Silicon wafers serve as the backbone of countless electronic devices, from smartphones to solar panels. The ability of a silicon wafer to efficiently conduct heat can significantly influence the performance and reliability of these products. This section tackles how thermal conductivity affects heat management, device efficiency, and ultimately, the lifespan of silicon-based products in various settings.
Measuring Thermal Conductivity
Laser Flash Analysis
Laser Flash Analysis (LFA) is a predominant method used in the measurement of thermal conductivity of silicon wafers. The technique involves sending a short laser pulse onto one side of the sample while monitoring the temperature increase on the opposite end. This allows for accurate calculation of thermal diffusivity. The key characteristic of this method is its speed and sensitivity, making it a preferred choice in labs for quickly obtaining results on thermal properties.
A unique feature of LFA is its minimal sample size requirement, which enables testing of small samples without significant material loss. Its advantage lies in its high precision and ability to assess a variety of materials, including thin films. However, one should note that LFA can struggle with very low thermal conductivity materials, leading to potential inaccuracies under certain conditions.
Hot Disk Technique
The Hot Disk technique is another method frequently employed to gauge thermal conductivity. This approach involves the use of a thin sensor that acts both as a heat source and temperature gauge. The sensor is sandwiched between two pieces of the silicon wafer and heats up, allowing the measurement of how quickly the heat spreads through the material.
A notable aspect of the Hot Disk technique is its versatility, as it can measure thermal conductivity at different temperatures and sample sizes. This method is popular due to its non-destructive nature, which preserves the integrity of the wafer after testing. Nevertheless, precision can suffer if the sensor is not properly calibrated, making it essential to account for this in the analysis.
Comparative Analysis with Other Materials
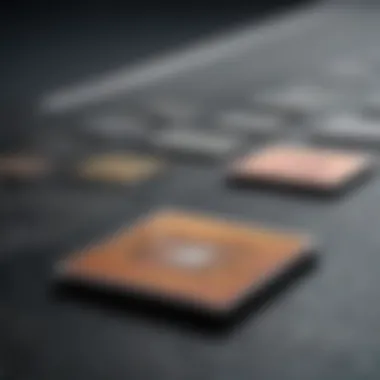
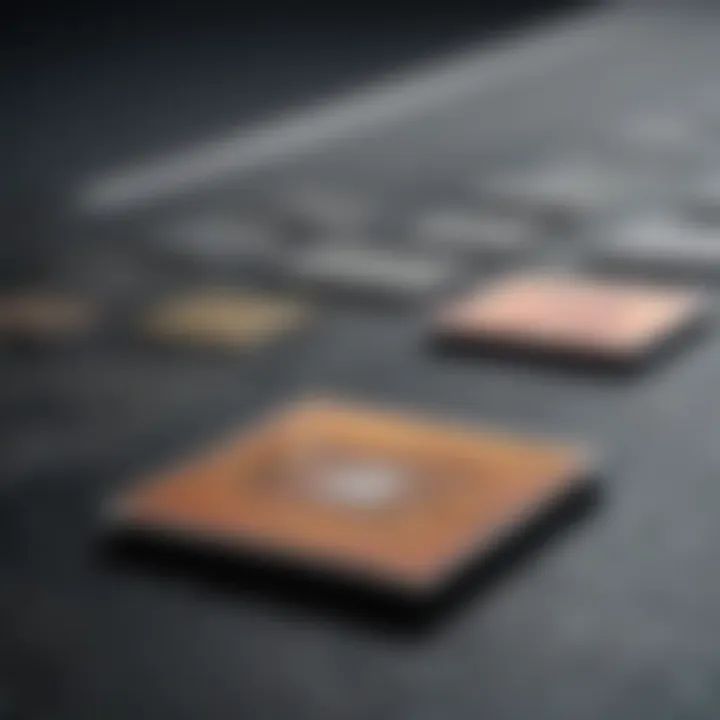
Silicon vs. Gallium Arsenide
When discussing thermal properties, it’s pivotal to compare silicon wafers with Gallium Arsenide (GaAs). Both materials are widely utilized in semiconductor devices, yet their thermal conductivity characteristics diverge. Silicon possesses higher thermal conductivity than GaAs, which translates to superior heat dispersion in high-performance applications. This characteristic makes silicon a bulkier thermal conductor, benefiting circuits that elevate in temperature under load.
The unique feature of silicon lies in its relatively stable thermal performance across varying temperatures, making it a reliable choice for diverse applications. On the other hand, GaAs, while offering certain advantages in speed and efficiency for specific devices, may face limitations when it comes to thermal management and reliability.
Silicon vs. Diamond
Diamond is frequently touted as the best thermal conductor known, surpassing silicon by a considerable margin. The thermal conductivity of diamond can be several times higher than that of silicon, fostering interest in diamond coatings or composites for thermal interface materials in advanced electronics. This contrast highlights silicon's advantages in terms of cost and availability, even if it lacks the ultimate thermal performance of diamond.
Silicon wafers are advantageous due to their widespread availability and established fabrication processes. However, in specific high-performance scenarios, diamond's exceptional thermal conductivity cannot be overlooked, particularly in applications demanding superior thermal management. While integrating diamond into technologies may provide thermal benefits, it also introduces challenges related to cost and material compatibility.
"A thorough understanding of the thermal conductivity is essential for tailoring material selection and enhancing device performance."
In summary, thermal conductivity in silicon wafers is not merely a technical detail; it is an integral factor influencing design decisions and operational efficiency in semiconductor applications. The comparative analysis with other materials emphasizes silicon's strengths while revealing situations where alternatives might outshine it. This information is crucial for researchers, educators, and professionals working to innovate in the field of electronics.
Applications of Silicon Wafers
Understanding the thermal conductivity of silicon wafers allows us to grasp their profound implications in the realm of technology. This discussion is pivotal because silicon wafers serve as foundational components in many modern devices. As we break down their applications, we will touch upon how their thermal properties affect performance, efficiency, and innovation across different fields, particularly in semiconductors and solar energy.
Impact on Semiconductor Devices
Transistors
Transistors are often heralded as the building blocks of modern electronics. Their ability to act as switches or amplifiers is paramount. When it comes to thermal conductivity, the quality of silicon wafers directly influences the performance of transistors. The unique feature of using silicon in transistors is that it allows for fast and efficient switching, which is essential in computing and communication technologies. High thermal conductivity contributes to heat dissipation, minimizing the risk of overheating and ensuring longevity in device operation. The balance between thermal performance and energy consumption makes silicon a favorable choice for designers looking to enhance performance.
However, it’s not all sunshine and rainbows—poor thermal management can lead to significant challenges. A common dilemma is that while silicon's thermal conductivity is well-suited for many applications, its limitations at higher temperatures can cap performance in extreme environments.
Integrated Circuits
Integrated circuits (ICs) are another cornerstone of modern electronics, enabling the miniaturization of components critical to innovation. The thermal conductivity of silicon wafers profoundly affects integrated circuits, primarily through heat dissipation. This characteristic prevents the circuits from overheating, which is detrimental for operational efficiency and reliability.
A key aspect of integrated circuits is their design flexibility, allowing for complex functionalities packed into a small area. The use of silicon wafers ensures that the thermal management of these compact systems remains effective. This is invaluable, as thermal hotspots can lead to malfunctions or reduce the lifespan of the device. On the flip side, as circuits get denser, managing heat becomes increasingly complex, making silicon’s inherent limitations in very dense configurations a challenging point of consideration.
Role in Solar Cells
Efficiency Improvements
Solar cells rely heavily on the thermal conductivity of silicon wafers to optimize their efficiency. The arrangement allows for effective heat transfer, which is critical in maintaining performance in varying environmental conditions. Improved thermal management techniques in silicon solar cells lead to higher conversion efficiencies, meaning more sunlight transformation into usable energy.
A notable aspect is how better thermal conductivity can enhance performance at higher temperatures, where other materials may falter. This advanced thermal performance not only boosts output but also extends the operational life of solar panels, making them a practical choice for both residential and commercial applications.
Thermal Management Strategies
Employing effective thermal management strategies within solar cells is essential for achieving optimal performance. The integration of materials that complement the thermal properties of silicon wafers offers significant advantages. Techniques such as heat sinks and advanced coatings can dramatically enhance heat dissipation, making solar cells more robust during peak sunlight hours.
While the efficiency of thermal management strategies can lead to greater energy yield, one must also consider cost implications and material compatibility. Finding a balance ensures that the advantages of improved thermal management don’t come at the expense of affordability or ease of production.
"The role of silicon wafers in various applications underscores the need for continuous improvement in thermal management strategies to harness their full potential in performance across multiple industries."
In summary, the applications of silicon wafers are extensive and overlap significantly within semiconductor devices and solar technologies. Their thermal characteristics significantly impact the effectiveness, efficiency, and longevity of the systems they are used in, shaping the future of technology.
Advancements in Material Science
The field of material science stands at a pivotal juncture, especially when considering the implications these advancements hold for silicon wafer technology. Recent strides in this arena are propelling both research and applications forward, and it's vital to grasp how these developments can reshape the landscape of semiconductor devices and thermal management systems. The advent of novel formations and processing techniques promises not just enhancements in performance, but also introduces innovative solutions to age-old challenges.
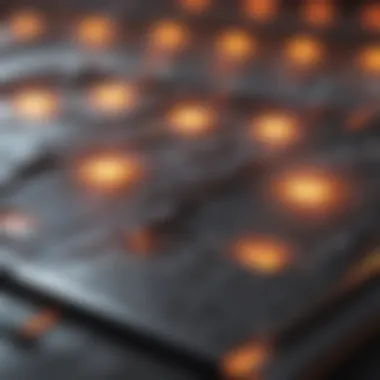
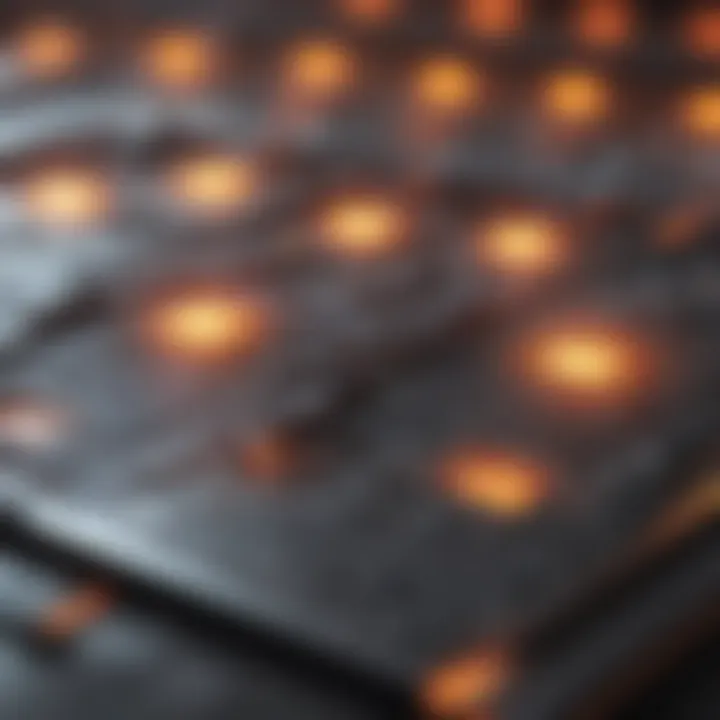
Novel Silicon Alloys
Silicon alloys have gained traction as researchers aim to fine-tune thermal properties and electrical performance. By blending silicon with other elements like germanium or tin, engineers create materials that exhibit superior characteristics compared to standard silicon. Germanium, for instance, is known for its excellent electronic properties, and when alloyed with silicon, it tends to improve carrier mobility, which can enhance device efficiency significantly.
Benefits of silicon alloys include:
- Enhanced thermal conductivity: Certain alloys can dissipate heat more effectively than pure silicon, making them ideal for high-power applications.
- Improved mechanical strength: Alloying often results in stronger materials that can withstand greater operational stress.
- Tailored electrical properties: By varying the composition of the alloy, one can optimize the semiconductor's behavior for specific applications, such as photonic devices or advanced integrated circuits.
While this provides a lot of prospects, it also comes with considerations. The manufacturing process often becomes more complex and may involve additional costs. Additionally, the long-term stability and reliability of these materials under various operational conditions remain an area of active investigation. Consequently, understanding these factors is crucial for harnessing the full potential of novel silicon alloys.
Nanostructured Silicon
Nanostructured silicon adds another layer of excitement to material science advancements. By manipulating silicon at the nanoscale, researchers unlock exciting possibilities that traditional materials cannot match. This includes working with silicon nanowires, nanopillars, and even silicon nanoparticles. Such structures inherently possess unique thermal and electrical properties, opening avenues for their application in diverse fields.
Several key points about nanostructured silicon include:
- Higher thermal conductivity: At the nanoscale, the phonon transport mechanisms change, often leading to materials that can conduct heat more efficiently. This property is invaluable in high-performance electronics.
- Size-dependent behavior: As the dimensions shrink, different physical phenomena come into play, which can be leveraged for advanced applications like sensors or photovoltaic devices that require enhanced light absorption.
- Sustainability: Nanostructured silicon often paves the way for more environmentally friendly processes and materials. By reducing material usage and potentially enhancing efficiency, these approaches may lower the overall carbon footprint of semiconductor manufacturing.
Nevertheless, alongside their advantages, nanostructured materials face challenges, particularly in scalability and consistency of properties during production. Ensuring a uniform quality can be quite the task, as variations at the nanoscale can lead to significant disparities in performance.
"Advancements in material science serve as the backbone for technological innovations, bridging the gap between theoretical exploration and practical application."
Challenges and Future Directions
Understanding the challenges and future directions related to the thermal conductivity of silicon wafers is vital in propelling the semiconductor industry forward. The demand for high-performance devices, which can handle greater power and efficiency, necessitates robust thermal management solutions. Three key areas merit attention: optimizing thermal management in high-power devices and exploring potential innovations in thermal enhancement.
Thermal Management in High-Power Devices
In high-power electronic devices, such as power amplifiers and high-frequency transistors, managing heat is a pressing concern. Excessive heat generation not only leads to device failure but also hampers performance and reliability. The thermal resistance within silicon wafers can often become a bottleneck, resulting in thermal gradients that adversely affect device operation. Addressing this issue involves a multidimensional approach:
- Material Selection: Choosing appropriate materials with high thermal conductivity can significantly influence the efficiency of heat dissipation.
- Packaging Techniques: Innovative packaging designs that utilize heat spreaders or heat sinks can facilitate improved thermal management, allowing for more effective heat removal.
- Thermal Interface Materials: Employing advanced thermal interface materials can enhance thermal contact and conduction between silicon wafers and other components, reducing thermal resistance.
While challenges abound, addressing thermal management not only enhances the reliability of high-power devices but also improves overall system performance, leading to longer product lifespans and greater efficiency.
Potential Innovations in Thermal Enhancement
Looking towards the future, innovations in thermal enhancement techniques promise to revolutionize how we manage heat in silicon wafer applications. Emerging research is highlighting various promising technologies, including:
- Phase Change Materials (PCMs): By utilizing materials that absorb or release heat during phase transitions, PCMs can actively manage temperature fluctuations within devices.
- Microchannel Heat Sinks: Exploring microchannel technology, which enables fluid flow at the microscale, offers an avenue for unprecedented thermal performance in compact electronic architectures.
- Graphene and Carbon Nanotubes: The potential of 2D materials like graphene and three-dimensional structures of carbon nanotubes is alive with possibilities. These materials exhibit superior thermal conductivity and could be integrated into silicon substrates to enhance heat dispersion.
"Developing new thermal management solutions is not just about keeping devices cool; it’s about unlocking the full potential of technology we rely on every day."
As we edge closer to the limits of conventional silicon technology, these innovations present a pathway to overcome existing thermal limitations. It’s essential for researchers and industry professionals to collaborate and bring forth new strategies that can address the ongoing challenges while exploring the vast potential that lies within the thermal management of silicon wafers.
The journey ahead is undeniably challenging, but with a sharp focus on heat management solutions, the future of silicon wafer technology can unfold in remarkable ways.
End
The conclusion of this article serves not just as a wrap-up, but as a critical lens through which we can appreciate the vast implications of silicon wafer thermal conductivity. Understanding these thermal properties is vital for anyone involved in semiconductor technology. As we've sifted through the details, several key points have emerged along with their far-reaching impacts.
Recap of Key Findings
To summarize the findings, we have discussed the complex nature of thermal conductivity in silicon wafers, along with measuring techniques like Laser Flash Analysis and Hot Disk Technique. We juxtaposed silicon with other materials, elucidating its specific pros and cons, particularly against gallium arsenide and diamond. Furthermore, the substantial influence of silicon’s thermal properties on semiconductor devices, like transistors and integrated circuits, was highlighted.
Notably, we have explored a myriad of applications ranging from solar cells to high-power electronic devices. The advances in material science, such as novel silicon alloys and nanostructured silicon, have inspired innovative thermal management strategies. All these elements underscore a unified truth: the thermal conductivity of silicon wafers is not merely a subject of academic interest; it’s something that bears directly on how effectively we can harness technology in real-world applications.
Implications for Future Research
As we look toward future research in this field, several avenues beckon exploration. Firstly, the realm of thermal enhancement techniques requires rigorous inquiry. This could encompass the synthesis of new materials or the optimization of existing ones, aiming to tailor thermal properties for specific uses. Additionally, examining the interplay between thermal performance and device longevity could yield insight into more resilient semiconductor applications.
Consider also the potential for cross-disciplinary studies that link thermal management with the advancements in energy efficiency. This not only broadens the scope of thermal conductivity research but also ties it to pressing global challenges such as resource conservation and sustainability.
In summary, the pursuit of knowledge in silicon wafer thermal conductivity carries significant weight for the electronics industry. The implications of continued investigation could pave the way for cutting-edge innovations, making it essential for students, researchers, educators, and professionals alike to stay attentive to developments in this pivotal area.