The Integral Role of Robotics in Space Exploration
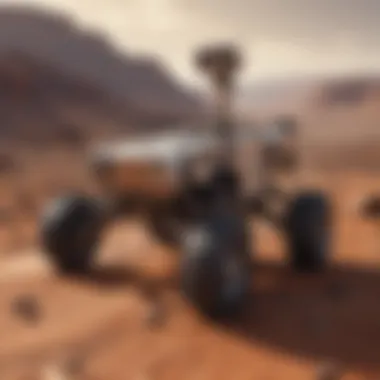
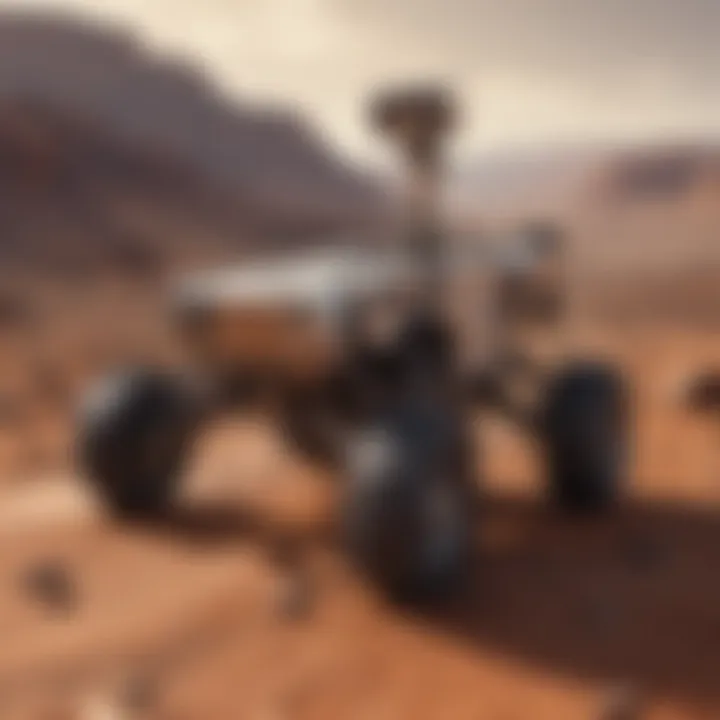
Research Overview
The integration of robotics into space exploration is a subject that has garnered significant attention in both academic and practical realms. The evolution of robotic systems over the decades exhibits a remarkable journey from rudimentary prototypes to sophisticated machines capable of conducting complex tasks in hostile environments.
Summary of Key Findings
Robotic technologies have proved essential for numerous missions, enabling the collection of data and enhancing our understanding of various celestial bodies. Notable examples include the Mars rovers, such as Curiosity and Perseverance, which have revolutionized our approach to planetary exploration. These rovers are equipped with advanced sensors and cameras that allow for high-resolution imaging and geological analysis.
Additionally, the contribution of robotic arms on the International Space Station (ISS), such as the Canadarm2, has facilitated maintenance and assembly tasks, increasing operational efficiency in low Earth orbit. This highlights not only the utility of robotics in exploration but also their role in supporting human presence in space.
Relevance to Current Scientific Discussions
The intersection of robotics and space exploration raises discussions about future missions to destinations such as Mars and beyond. The utilization of autonomous robots will be critical in those endeavors, especially for tasks considered hazardous for human astronauts. Furthermore, as technology continues to advance, the potential for robotics to play an even more significant role in enabling deep space missions becomes increasingly viable. The implications of robotics traverse beyond mere functionality; they venture into ethical realms concerning human-robot collaboration, risk management, and the future of human presence in extraterrestrial environments.
"The growing capabilities of robotic systems will redefine how humanity explores the final frontier."
Methodology
Research Design and Approach
This exploration utilizes a mixed-method design that incorporates both qualitative and quantitative analyses. The qualitative analysis stems from an examination of historical mission data, while quantitative assessment may involve metrics related to mission success rates and the efficiency of robotic tasks.
Data Collection and Analysis Techniques
Data collection involves a thorough review of existing literature, mission reports, and technical documents from agencies such as NASA and ESA. Analysis techniques may include thematic coding for qualitative data and statistical methods for quantitative metrics. This allows for a well-rounded understanding of robotic contributions to space exploration and identifies patterns that inform future developments.
When we talk about space exploration, we refer to vast and remote areas with extreme conditions. Traditional space missions often involve human astronauts, which come with a variety of risks and limitations. By integrating robotics into these missions, we enhance efficiency and safety. Robots can withstand conditions too severe for humans and can operate autonomously, gathering data and performing experiments far beyond Earthโs atmosphere.
Benefits of Robotics in Space
Robotics can drastically reduce mission costs by replacing the need for human presence in certain exploratory roles. They also extend the duration of services in space through reliable automation, performing repeated tasks with precision. Moreover, robotic systems can be programmed to explore environments that are yet fully understood, such as the icy moons of Jupiter or the surface of Mars.
Definition and Scope
Robotics refers to the design, construction, operation, and use of machines that can perform tasks on their own. In the context of space exploration, this includes a wide array of technologies like rovers, landers, orbiters, and even space probes. The scope of robotics in this field covers everything from autonomous movement and navigation systems to advanced imaging and sample collection tools.
Robots may operate remotely, guided by human operators on Earth, or function autonomously with advanced artificial intelligence. The technologies behind robotics in space vary but include sensors, actuators, and robotic arms that allow for intricate movements and operations.
Historical Context
The journey of robotics in space began in the mid-20th century with early satellite missions. For instance, the Sputnik, launched by the Soviet Union in 1957, marked a significant moment in utilizing robotic technology for space exploration. As technology progressed, space agencies began to deploy more sophisticated robotic systems. For instance, NASA's Viking program in the 1970s employed landers on Mars that collected and transmitted data back to Earth.
In the following decades, significant milestones such as the Mars Pathfinder mission, which included the Sojourner rover, further demonstrated the potential of robotics in exploring alien terrains. Each advancement paved the way for our current understanding of robotic systems and their capabilities in space.
"Robotics not only changes how we explore, but also enhances our capability to survive in environments without human life support."
Evolution of Robotic Technologies
The evolution of robotic technologies marks a significant chapter in the narrative of space exploration. Understanding this progression is essential for grasping how modern robotics enhances our capabilities in space. Each stage in the development of these technologies brings with it enhanced functionality, improved design, and the potential for new discoveries. The importance of these developments is not just historical; they shape current missions and help prepare for future endeavors.
Early Developments
Robotic technologies began to emerge in the context of space exploration soon after the dawn of the space age. The late 1950s and early 1960s saw the initial designs of robotic spacecraft and landers. The first successful robotic spacecraft was the Soviet Luna 2, which reached the Moon in 1959. The significance of this achievement was monumental; it set a precedent for using machines to explore beyond our planet and informed future designs. These early robots were basic and lacked autonomy. They were remote-controlled and heavily dependent on human operators.
As technology progressed, the need for more sophisticated equipment became evident. The Mariner series, starting in the mid-1960s, utilized advanced instruments that allowed for effective long-range spaceflight and gathering of scientific data. The ability to send back images and data about Mars was groundbreaking. It paved the way for developments in robotics that could function autonomously, reducing reliance on human intervention in hostile environments.
Milestones in Robotics
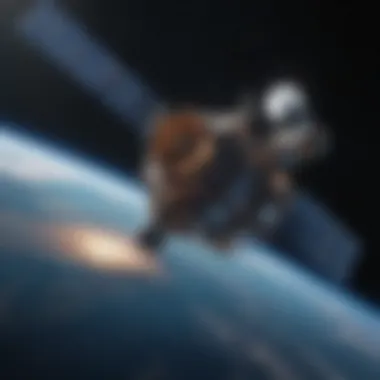
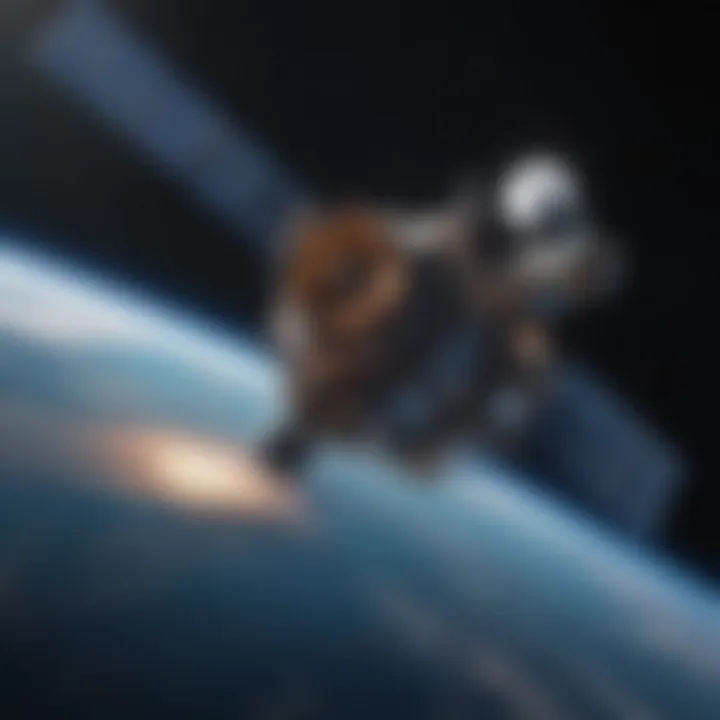
Throughout the 1970s and 1980s, a series of milestones marked the advancement of robotic technology in space. This era was characterized by the launch of several landmark missions. For instance, the Viking missions to Mars in 1976 represented a leap in robotic technology. These landers had the ability to conduct experiments on the Martian soil and transmit the results back to Earth.
Following this, the exploration of the outer planets was revolutionized by the Voyager probes launched in 1977. They carried advanced instruments capable of conducting long-term studies over vast distances. The successes of these missions demonstrated the capability of robotic technologies to gather data from some of the most remote areas of our solar system. Such milestones have provided invaluable insights into planetary systems and fueled further investment in robotics for space exploration.
Advancements in Automation
The advances in automation have profoundly influenced robotic technologies in space exploration. The introduction of systems that allow for semi-autonomous functions has proven essential. For example, the Mars rovers, such as Spirit and Opportunity, introduced innovative navigation and obstacle avoidance techniques. They were equipped with sophisticated algorithms that allowed them to make decisions based on the environment around them.
As a result, these rovers were able to extend their missions significantly beyond their expected lifetimes, showcasing enhanced durability and functionality. Enhanced automation has also allowed for the design of robotic systems that can operate under extreme conditions. As space missions continue to target distant locations, these automated systems ensure safety and efficiency, critical factors in exploring challenging environments.
Key Missions Utilizing Robotics
Robotics plays a vital role in modern space exploration. It extends our reach to distant planets and moons, enhances scientific understanding, and maintains operations in the harsh environment of space. The missions utilizing robotic technologies show how these systems can perform tasks that are either too dangerous or infeasible for humans. This section delves into the significant missions where robotics has made a difference, examining specific examples like the Mars Rovers, Voyager Probes, and robotics on the International Space Station.
Mars Rovers
Mars Rovers are among the most significant robotic missions. They have provided invaluable data about the Martian surface, atmosphere, and potential for life.
Spirit and Opportunity
Spirit and Opportunity were twin rovers launched in 2003. Their main goal was to explore the Martian surface and analyze soil and rock samples. These rovers operated far beyond their expected lifespan, providing crucial insights into Mars' geological history. The key characteristic of Spirit and Opportunity was their ability to traverse and analyze varied terrains. This mobility enabled them to access diverse geological features across multiple locations.
The advantage of these rovers was their durability and flexibility in operations. However, their communication systems faced challenges due to the distances between Earth and Mars, leading to delays in data transmission. Overall, they significantly advanced our understanding of Mars.
Curiosity
Curiosity rover, launched in 2011, is equipped with advanced scientific instruments that allow it to assess the habitability of Mars. The highlight of Curiosity is its nuclear power source, which enables long-term and sustainable operations without the limitations of solar energy.
Curiosity's unique feature is its ability to analyze soil samples and conduct in-situ experiments. This feature provides real-time data, making it beneficial for scientists on Earth. However, the complexity of its systems can lead to higher risks of malfunction, which is a disadvantage in remote exploration.
Perseverance
Perseverance rover, launched in 2020, aims to seek signs of ancient life and collect samples for future return to Earth. Its key characteristic is the advanced technology it employs, including the MOXIE instrument, which converts Martian carbon dioxide into oxygen.
The advantage of Perseverance lies in its sophisticated capabilities for sample collection and analysis. This could pave the way for future human missions by assessing resources available on Mars. However, as it operates in unfamiliar terrain, it faces potential risks related to navigation and unexpected environmental conditions.
Voyager Probes
The Voyager probes, launched in the late 1970s, are among the most successful robotic missions ever conducted. Voyager 1 and Voyager 2 have provided critical data about the outer planets and are now in interstellar space. Their engineering and longevity are significant achievements in robotics. Each probe carries instruments capable of conducting scientific measurements and transmitting data back to Earth, often facing immense distances and communication delays. This showcases the effectiveness of robotic systems in extending human presence beyond our solar system.
International Space Station Robotics
The International Space Station (ISS) has been a hub of robotic innovation. Robotics here enhances capabilities, facilitating maintenance and experimentation.
Robonaut
Robonaut is a humanoid robot developed to assist astronauts on the ISS. Its design mimics human movements, allowing it to interact with tools and perform tasks that typically require human dexterity.
The unique feature of Robonaut is its advanced sensors and control systems that enable it to work alongside astronauts. The advantage is its potential to reduce astronaut workload and increase safety in hazardous tasks. However, its development has been gradual, and operational limitations remain.
Canadarm2
Canadarm2 is a robotic arm used on the ISS for manipulation tasks. Its main function is to move cargo and assist with satellite repairs. The distinct characteristic of Canadarm2 is its versatility. It can position payloads with precision, proving essential for station upkeep.
The advantage of Canadarm2 is that it enhances operational efficiency on the ISS. However, it requires human oversight for complex operations, demonstrating the ongoing need for collaboration between humans and robots.
In summary, key missions utilizing robotics illustrate the immense potential of these technologies in space exploration. They enhance our understanding of distant worlds, improve operational safety, and expand our capabilities in environments that are hostile to human life.
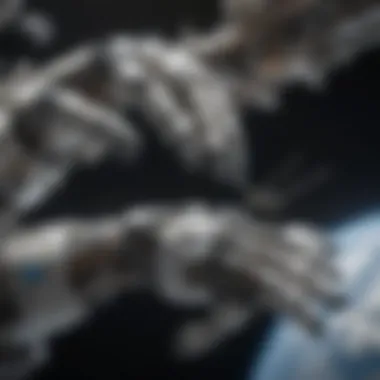
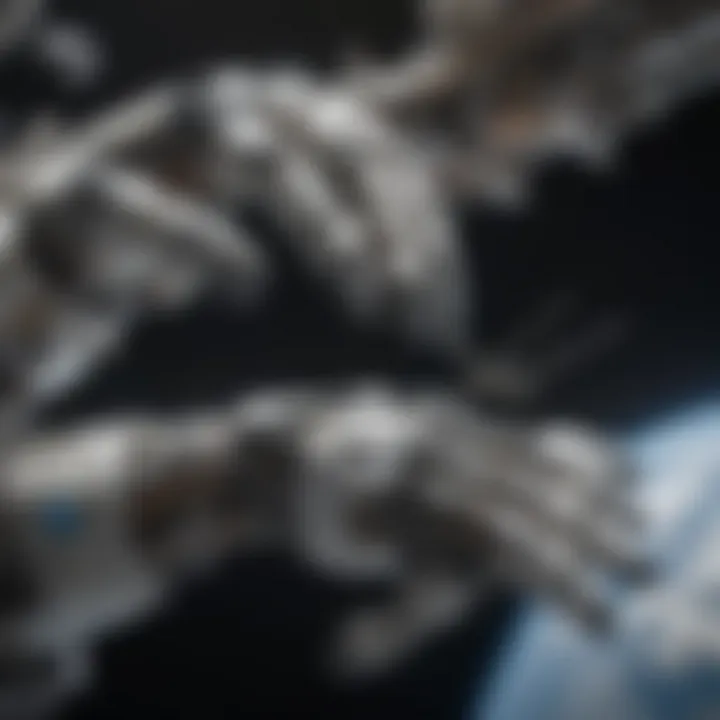
Benefits of Robotics in Space
Robotics has become a cornerstone of modern space exploration. The intersection of technology and science has led to significant advancements that enhance the ability of humanity to explore the depths of space. The benefits of using robotics in this domain are manifold, addressing key areas such as scientific research, operational safety, and cost efficiency. These aspects resonate with the demands of space missions, significantly affecting mission outcomes and the overall understanding of our solar system and beyond.
Enhanced Scientific Research
The role of robotics in advancing scientific knowledge cannot be overstated. Space robots, such as the Mars rovers, serve as invaluable tools for data collection and analysis. They can traverse harsh terrains where humans cannot easily go, gathering samples and conducting experiments on-site. This capability allows scientists to study planetary geology, atmospheres, and potential life forms in ways that were previously unimaginable.
Robots like NASAโs Curiosity and Perseverance have been instrumental in exploring Martian surface conditions and geology. By analyzing rock samples and atmospheric composition, these rovers provide critical data that continues to enrich our understanding of Mars. The insights gained from robotic missions often lead to new theories and hypotheses about the formation and evolution of planetary bodies.
Moreover, the longevity and durability of robots in space significantly augment the research timeline. Unlike human-led missions, which are constrained by factors like life support, robotic missions can continue for extended periods, returning data that might otherwise be lost.
Operational Safety
A key benefit of using robots in space is the enhanced safety they provide for human missions. Robots can perform hazardous tasks in extreme environments, mitigating risks to astronauts. For instance, during missions to Mars or distant asteroids, robots can scout areas, perform diagnostic tasks, and even conduct repairs on equipment. They can address potential threats before human involvement is necessary, allowing for a safer and more controlled environment for astronauts.
The operation of robots reduces the likelihood of human error in high-risk situations. In scenarios like a malfunction due to unexpected environmental conditions, robots can autonomously execute emergency protocols. By acting autonomously or semi-autonomously, robots bring an additional layer of reliability, which is crucial when mission stakes are incredibly high.
Cost Efficiency
Cost efficiency is another significant advantage of integrating robotics into space exploration. Robotic missions typically require less financial investment compared to crewed missions. The absence of the need for complex life support systems, extensive training for astronauts, and associated logistics all contribute to reduced costs in robotic missions.
Additionally, the data gathered through robotic explorations can save future missions. For instance, a successful robotic survey of a distant planet might remove uncertainty in mission planning, allowing for more precise targeting and deployment of resources during human-led missions later on.
Robotics not only enhances safety and scientific discovery, but also maximizes budget potentials in the expensive domain of space exploration.
Challenges in Robotic Space Missions
Robotic space missions present distinctive challenges. Understanding these obstacles is crucial as they directly impact mission success and the capacity of robotic systems to function effectively in hostile environments. Each challenge signifies different considerations that engineers and scientists must address to ensure the reliability and effectiveness of robotic technologies in space exploration.
Technological Limitations
Technological limitations form the backbone of challenges faced in robotic missions. Despite significant advancements, the harsh conditions of space often expose weaknesses in existing robotic systems. These limitations include issues related to power supply, mobility, and sensor performance. For example, solar panels may become less effective over extended periods or in shaded areas of a planetary surface. Moreover, the physical and computational capabilities of robots can restrict their ability to perform complex tasks. The need for ever-more sophisticated robotics is evident, driving research and development to overcome such limitations. The challenge here is to innovate while ensuring the systems remain reliable and efficient. As robotics in space matures, addressing these technological gaps becomes essential for future missions.
Communication Delays
Communication delays stand as another significant challenge for robotic space missions. Distances in space create substantial latency. For example, signals from Mars to Earth can take up to 20 minutes one way. This delay complicates real-time operation and control of robotic systems. When mission operators send commands, they must anticipate the delay, which can lead to decision-making challenges. Autonomy becomes vital in such situations, necessitating robots that can make choices based on limited information. Efficient communication systems must evolve to mitigate issues. Increased focus on autonomous operations within robots will help address these challenges.
Environmental Hazards
Environmental hazards pose considerable threats to robotic missions. Extreme temperatures, radiation, and dust storms are just a few examples of the hostile conditions in space and planetary environments. These factors can damage robotic systems and affect their operations significantly. For instance, dust accumulation on solar panels can reduce energy efficiency. Similarly, exposure to cosmic radiation can compromise the electronics of robotic equipment. Engineers must design robust systems that can withstand these hazards. Incorporating materials that endure extreme conditions and ensuring proper shielding for electronics are essential lead to resilient robots.
"Developing robotics that can traverse and operate effectively in alien environments requires extensive R&D."
Future Trends in Space Robotics
Increased Autonomy
One significant trend is the movement towards increased autonomy in robotic systems. Autonomy allows robots to perform tasks without constant human supervision, which is crucial in environments where communication delays occur, such as on Mars or distant moons. More autonomous robots can make real-time decisions, adapting to unforeseen circumstances and increasing mission success rates. This property is especially important for future missions, where crews may be light years away from Earth.
Furthermore, as autonomy improves, robots can handle more complex tasks. For instance, space rovers could be programmed to autonomously conduct geological surveys or identify and analyze samples. The benefit is clear: missions become more efficient and effective, providing scientists with greater data return. Increased autonomy also reduces the risks to human astronauts, who may work alongside these machines in dangerous conditions.
Artificial Intelligence Integration
Artificial intelligence (AI) is another pivotal component shaping the future of space robotics. AI enables robots to learn from their experiences and enhance their decision-making processes. This technology can revolutionize how robots interact with their environments. For example,
- AI could enable a robot to recognize various geological features on a planetary surface, allowing it to prioritize areas for investigation.
- Machine learning algorithms could be used to optimize navigation paths, making travel faster and safer in harsh terrains.
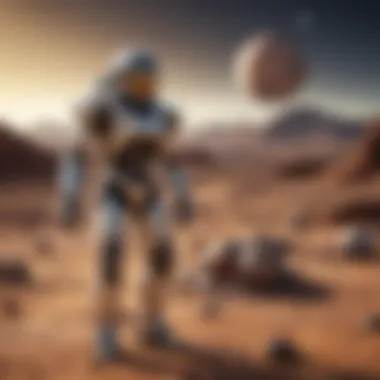
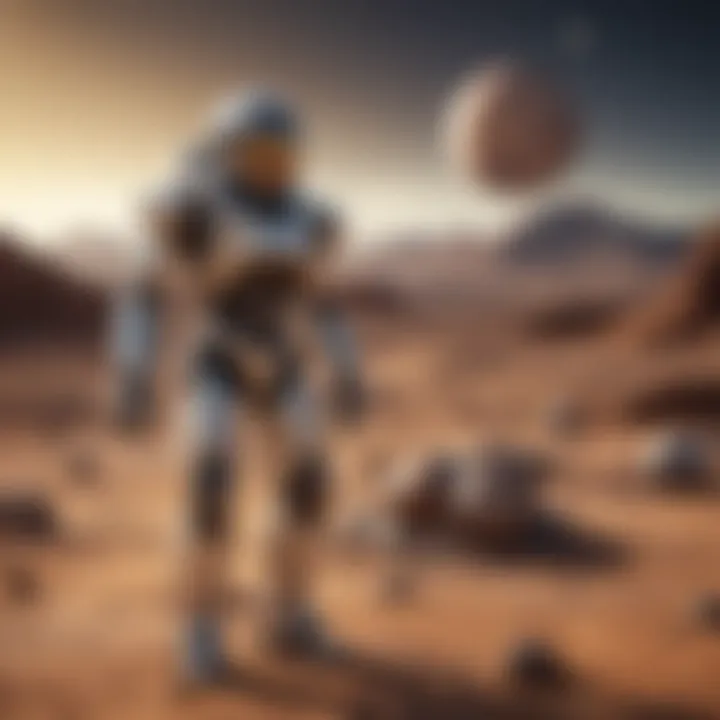
Integrating AI can lead to smarter robotic assistants that provide real-time data analysis and operational support. The implications extend to mission planning as well. With AI, mission planners can simulate various scenarios and potential outcomes, helping to create more robust mission profiles.
Interplanetary Missions
The advancement of robotics is crucial for future interplanetary missions. As agencies like NASA and private companies push the boundaries of exploration, robotic systems will play a central role. These missions will require highly sophisticated robots capable of navigating uncharted environments and executing complex tasks.
Robots designed for interplanetary travel can serve multiple purposes. They can conduct scientific experiments, prepare landing sites, and even construct habitats for future human travelers. Moreover, the collaboration between robots and astronauts becomes essential in these missions, as they complement each other's capabilities. This partnership will facilitate deeper exploration of locations such as Europa or Titan, whose unique characteristics present new challenges and research opportunities.
"Advancements in space robotics will unlock the mysteries of our solar system, paving the way for human exploration."
Human-Robot Collaboration
Human-robot collaboration is a significant aspect of modern space exploration. It refers to the symbiotic relationship between human operators and robotic systems. This collaboration enhances the effectiveness of space missions, allowing for a broader range of activities and tasks to be accomplished in the harsh environments of outer space. The interplay between human intuition and robotic precision proves valuable in various scenarios, particularly in areas where human presence may be limited or hazardous.
The key benefits of this collaboration include improved operational efficiency, access to remote areas, and the ability to handle tasks that are too dangerous for humans. Robots equipped with advanced sensors and algorithms can gather data, conduct repairs, and perform experiments in ways that complement human expertise. For instance, astronauts on the International Space Station often rely on robotic systems to carry out routine maintenance tasks, allowing them to focus on complex scientific research.
Furthermore, the collaboration opens up new avenues for future missions, as human-robot teams are essential for ambitious goals such as Mars colonization and beyond. Organizations like NASA plan to integrate these technologies into their exploration strategies, recognizing that human inputs can guide robotic decision-making. This partnership is not without challenges, as it raises questions about control and autonomy. It is crucial to strike a balance where robots operate effectively while still under human supervision.
Cooperating on Space Missions
Cooperation between humans and robots during space missions has become a fundamental element of exploration efforts. This cooperation is particularly evident in complex missions where the risks and difficulties are higher than what a team of humans could handle alone. Robotic platforms can perform reconnaissance missions, scout potential landing sites, or assist in extracting samples from celestial bodies.
Robotic systems like the Canadarm2 on the International Space Station extend astronauts' reach and capabilities, handling tasks that would be impossible without them. Through remote operation, astronauts can guide these robots effectively, ensuring safety and efficiency.
This teamwork is not limited to physical tasks. Communication plays a vital role. Astronauts must interpret data and make real-time decisions based on robotic inputs. This two-way interaction forms a responsive operational environment, essential for the mission's success.
Training for Collaboration
The effectiveness of human-robot collaboration hinges on how well crew members are trained to work alongside robotic systems. Training programs focus on familiarizing astronauts with robotic interfaces and behaviors to improve their performance in space.
Interactive simulations are commonly used to prepare astronauts for tasks they will confront. These simulations include scenarios that mimic real-life challenges they may face in space. The aim is to build confidence and ensure that astronauts can adapt swiftly to changes during missions.
Moreover, ongoing training ensures that operators can make informed decisions about when to delegate tasks to robots. Such training promotes a deeper understanding of the limitations and strengths of the robotic systems in use. This preparation is crucial as missions become more complex and automated and require higher levels of human oversight and flexibility.
The integration of training programs that emphasize human-robot collaboration can significantly enhance the success rate of future space missions.
Ethical Considerations in Space Robotics
In recent years, ethical considerations in the realm of robotics have gained prominence, particularly in space exploration. As robotic systems become increasingly autonomous, it is vital to examine the implications of this autonomy. The integration of robots into missions presents a range of ethical challenges that require careful consideration.
Autonomy vs. Control
One of the major ethical dilemmas is the balance between autonomy and control. Robots like the Perseverance rover operate on distant planets where communication delays can range from several minutes to over twenty minutes. This lag makes real-time control impractical. Thus, these robots must be equipped with advanced decision-making capabilities. However, this autonomy raises questions about accountability. If a robot makes a decision that leads to unintended consequences, who is responsible? Is it the engineers who programmed it, the mission planners, or is it the technology itself? The delegation of control to autonomous systems can lead to challenges in accountability and transparency.
Decision-Making Frameworks
"The responsibility to shape robotic decision-making lies not only with technologists but also with ethicists and policymakers."
Epilogue
In this article, we focused on the crucial role robotics plays in space exploration. The integration of robots into missions has brought significant advancements in science and technology, enabling exploration of areas previously considered too hazardous or distant for human presence.
Summary of Findings
We observed several key findings regarding the use of robotics in space. First, robotic systems have been instrumental in exploring planetary surfaces, such as Mars, where rovers like Curiosity and Perseverance gather valuable data. Second, robotics enhance operational safety by conducting tasks that would pose risks to human astronaut lives. Additionally, these systems provide cost efficiency, often reducing the expenses associated with human spaceflight. Importantly, robotics have enabled us to gather immense amounts of scientific data, advancing our knowledge of the universe.
"Robots have expanded our reach in the cosmos, allowing us to explore places we once thought unattainable."
Implications for Future Research
Looking forward, the implications for future research in robotics for space exploration are vast. It is vital to investigate how increased autonomy in robotic systems can lead to more efficient missions, particularly in environments where communication with Earth is delayed. Moreover, integrating artificial intelligence into these systems presents opportunities for enhanced decision-making capabilities on distant planets.
As we contemplate interplanetary missions, the collaboration between human crews and robotic systems will become essential. Future research can focus on developing frameworks for effective interaction between humans and robots to optimize mission outcomes. Considering ethical implications, the discussions around autonomy versus control will shape how we deploy these technologies in space.