Recombinant Expression: Techniques and Challenges
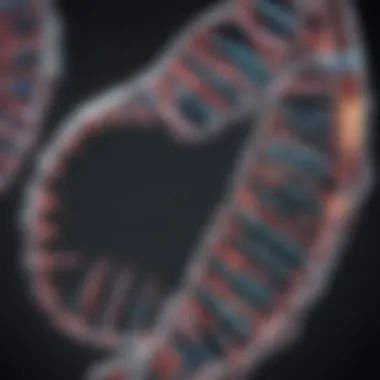
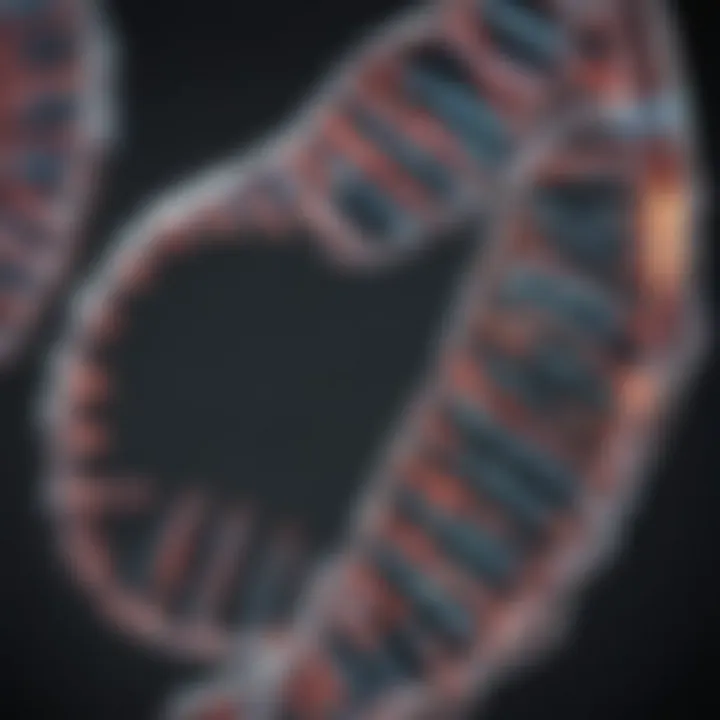
Intro
Recombinant expression is a pivotal method in biotechnology, prominently enabling the production of proteins with enhanced properties. This process involves the insertion of specific genes into host cells, which then utilize their cellular machinery to produce the corresponding proteins. Understanding this technique is essential for students, researchers, educators, and professionals engaged in the life sciences.
This article dissects various aspects of recombinant expression, including the most effective techniques, real-world applications across different industries, and the inherent challenges researchers face. Furthermore, it highlights the dynamic nature of protein production, inviting a deeper look into potential solutions for identified issues. The exploration of recombinant expression will benefit those striving for innovation and efficiency in medicine, industry, and related fields.
Understanding Recombinant Expression
Recombinant expression is a cornerstone of modern biotechnology. It enables the production of proteins by inserting genes into host organisms, leading to a range of practical applications. Understanding recombinant expression is essential for researchers and professionals working in fields like molecular biology, pharmacology, and bioengineering. The significance of this topic resides in its potential to address biological questions, develop therapeutics, and propel industrial advancements.
One key benefit of recombinant expression is its ability to generate large quantities of proteins that would be difficult or impossible to purify from native sources. This capacity expedites the development of diagnostic tools, vaccines, and therapeutic agents. Moreover, it allows for the modification of proteins to enhance their stability, activity, or specificity - factors crucial for various applications.
As such, grasping the fundamental principles and methodologies of recombinant expression becomes imperative for driving innovation in biotechnology.
Definition and Importance
Recombinant expression refers to the process of producing a specific protein by introducing and expressing a gene within a host cell. This technique exploits the host's cellular machinery to transcribe and translate the inserted gene, leading to the synthesis of the target protein.
The importance of recombinant expression extends beyond mere protein production. In many ways, it underpins advancements in medical therapies, agricultural improvements, and industrial applications. By allowing the precise tailoring of proteins, it fosters breakthroughs in drug design and biomolecule engineering, which ultimately serve to address complex challenges faced by society.
Historical Background
The journey of recombinant expression began in the 1970s with the advent of recombinant DNA technology. Early pioneering work by scientists such as Paul Berg and Herbert Boyer laid the groundwork for the first instances of gene cloning. In these initial stages, researchers learned how to isolate and manipulate DNA fragments. This opened up possibilities for expressing proteins in various systems.
By the 1980s, the first recombinant protein products were available, including human insulin and growth hormone. This marked a turning point in medicine, leading to significant shifts in how diseases were treated. As technology evolved, so did the methodologies employed for recombinant expression, paving the way for advancements in vectors, promoter selection, and expression systems.
Today, recombinant expression continues to be a rapidly evolving field. Each development brings newfound potential, shaping the future of biotechnology and its contributions to health, industry, and research.
Molecular Mechanisms of Recombinant Expression
Understanding the molecular mechanisms involved in recombinant expression is crucial for advances in biotechnology and genetic engineering. These mechanisms underpin the entire process of producing recombinant proteins, which serve vital functions in research, diagnostics, and therapeutics.
By unraveling the complexities of how genes can be manipulated and expressed in different systems, scientists can create proteins with desired properties. The detailed examination of techniques such as gene cloning, vector systems, and regulatory elements allows for a comprehensive grasp of this intricate and essential field. Furthermore, recognizing the molecular pathways involved facilitates the optimization of these methods to improve yields and functionality in applications.
Gene Cloning Techniques
Restriction Enzyme Digestion
Restriction enzyme digestion is a fundamental method in molecular biology for manipulating DNA. This technique utilizes enzymes that cut DNA at specific sequences, allowing for targeted modifications. The precision of restriction enzymes makes them an essential tool in gene cloning.
A key characteristic of this method is its ability to create complementary ends on DNA fragments. This feature is beneficial because it simplifies the subsequent steps of ligation and insertion into vectors. However, one must consider that the specificity required can sometimes limit the choice of restriction sites, which may not always be available within the target gene.
Ligation
Ligation is the process that follows restriction enzyme digestion, where DNA fragments are joined together. This step is crucial for ensuring that the desired insert is incorporated into a vector, allowing for gene expression in host cells.
One significant aspect of ligation is the use of DNA ligase, an enzyme that facilitates the joining of DNA strands. Its efficiency contributes to higher success rates in cloning. Nevertheless, the process can be influenced by factors such as the concentration of DNA fragments and ligase. Managing these variables is essential for optimizing the cloning process and reducing the possibility of unsuccessful attempts.
Transformation
Transformation is the method by which plasmid DNA is introduced into host cells. This step is vital for achieving recombinant expression, as it allows a host to produce the desired protein encoded on the plasmid.
Transformation is popular due to its relatively simple protocol, especially in bacterial systems like E. coli. However, the efficiency of transformation can vary depending on several factors, including the method used (e.g., heat shock or electroporation) and the state of the host cells. Ensuring optimal conditions can enhance the success rate of this critical step.
Vector Systems
Plasmid Vectors
Plasmid vectors are circular DNA molecules that replicate independently within host cells. They play a pivotal role in recombinant DNA technology, providing a means to carry and express foreign genes.
One of the key advantages of plasmid vectors is their versatility. They can incorporate various elements, such as promoters and selection markers, which aid in the identification of successful transformations. However, their size limitations can restrict the amount of genetic material they can carry. This consideration is important for projects that require larger genes or multiple genes to be expressed simultaneously.
Bacteriophage Vectors
Bacteriophage vectors utilize viruses that infect bacteria to deliver DNA into host cells. This method allows for high-efficiency gene transfer and is particularly useful for cloning larger fragments of DNA.
The unique aspect of bacteriophage vectors is their ability to integrate into the bacterial genome, which can lead to stable expression of the inserted gene. This characteristic makes them attractive for specific applications. However, the complexity of working with bacteriophages and the potential for safety issues pose challenges that researchers need to navigate.
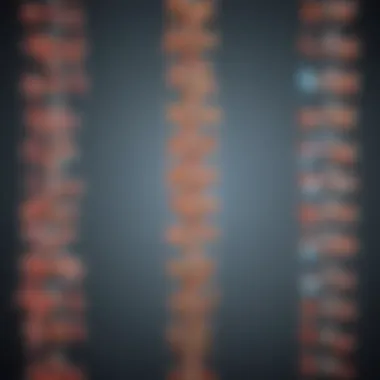
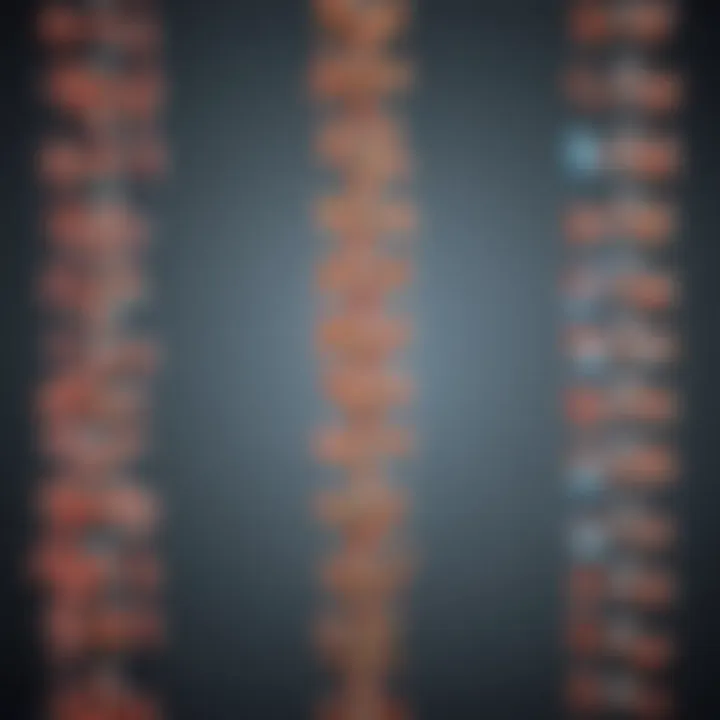
Viral Vectors
Viral vectors are engineered viruses used to deliver genetic material into various types of cells, including mammalian cells. Their potency lies in their natural ability to infect cells efficiently.
A major advantage of viral vectors is their capability to transduce a wide range of cell types, making them powerful tools in both research and therapeutic settings. On the downside, some viral vectors may raise safety concerns due to their pathogenic potential. Furthermore, the production and purification of viral vectors can be more elaborate compared to plasmid systems, complicating their use in routine applications.
Promoters and Regulatory Elements
Constitutive Promoters
Constitutive promoters are sequences that drive the continuous expression of a gene. They are fundamental for experiments requiring consistent production of a protein over time.
The defining trait of constitutive promoters is their ability to provide stable and ongoing transcription in various conditions. This makes them a reliable choice for many applications. However, their lack of regulation can lead to challenges in contexts where tight control of gene expression is desired.
Inducible Promoters
Inducible promoters are sequences that allow gene expression to be controlled by specific stimuli. This targeted approach is particularly useful in research scenarios where timing of protein production is critical.
A significant feature of inducible promoters is their ability to minimize background expression, which can be crucial to studying the function of proteins without interference. However, optimizing the conditions for induction can sometimes be challenging, particularly if the response to the inducer is variable or unpredictable.
Enhancer Elements
Enhancer elements are regulatory sequences that can significantly increase the expression of a gene. They do not necessarily need to be located close to the target promoter, adding a level of flexibility to genetic design.
The key advantage of enhancer elements is their ability to boost expression levels substantially, which can lead to more productive recombinant protein yields. However, the complexity in designing constructs that effectively utilize enhancers can complicate experiments. Careful design is essential to harness their full potential without causing unwanted effects.
Recombinant Expression Systems
Recombinant expression systems are the backbone of modern biotechnology. They provide the necessary environments for the production of proteins that have significant implications across various fields, including medicine and research. The choice of system can impact the yield, quality, and functionality of the protein produced, making it vital to understand different systems available.
Prokaryotic Systems
Prokaryotic expression systems, particularly those based on Escherichia coli, are widely used due to their simplicity and efficiency. These organisms can rapidly grow in inexpensive media, allowing for quick protein production.
The advantages of prokaryotic systems include:
- High yield: They often produce proteins in large quantities in a short time.
- Ease of manipulation: Genetic engineering techniques are well-established in these systems.
- Lower cost: The resources needed for growth are relatively cheap compared to eukaryotic systems.
However, there are limitations. Prokaryotic cells lack the necessary machinery for post-translational modifications, which are crucial for the functionality of many eukaryotic proteins. Therefore, while prokaryotic systems serve many purposes well, they may not always be suitable for complex proteins requiring modifications.
Eukaryotic Systems
Eukaryotic systems are essential for producing proteins that need modifications similar to those performed in human cells. This systems include yeast and mammalian cells.
Yeast Expression Systems
Yeast expression systems like Saccharomyces cerevisiae have gained recognition due to their capability to perform some post-translational modifications. They offer a middle ground between prokaryotic and mammalian cells in terms of complexity and cost.
Key characteristics include:
- Eukaryotic features: Yeast can glycosylate proteins, making them more similar to those produced in human cells.
- Cost-effective: Compared to mammalian cell cultures, yeast systems are generally less expensive to maintain.
A disadvantage is that yeasts may produce glycosylation patterns that do not exactly mimic mammalian systems, which can affect protein efficacy in therapeutic applications.
Mammalian Cell Culture
Mammalian cell culture is the gold standard for producing proteins that require complex post-translational modifications. HEK293 and CHO cells are among the most commonly used. This choice allows researchers to generate proteins that are structurally and functionally similar to those in humans.
Unique features include:
- Comprehensive modifications: They can perform all types of post-translational modifications, making the proteins functional and active.
- Valuable for therapeutics: Particularly important for therapeutic proteins such as antibodies and hormones.
However, mammalian cell culture presents challenges, including:
- Higher costs: Growth media and bioreactor setups can be expensive.
- Lower yield: Compared to prokaryotic systems, the quantity of protein produced is often lower.
Insect Cell Systems
Insect cell systems, such as Spodoptera frugiperda cells, offer another alternative for recombinant protein expression. These systems are particularly useful for expressing eukaryotic proteins with necessary modifications.
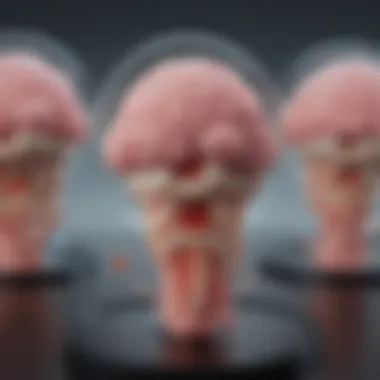
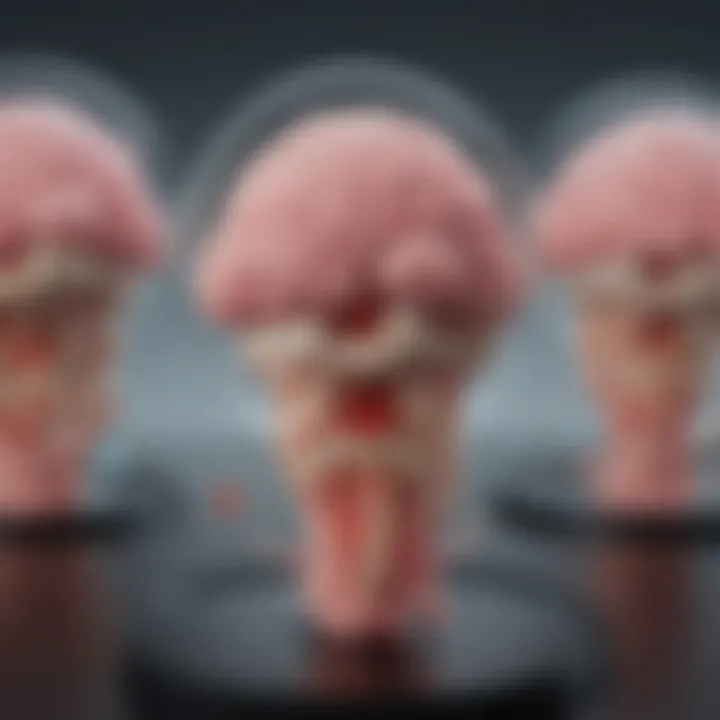
Insect cells can grow in simpler media compared to mammalian cells, combining advantages from both prokaryotic and eukaryotic systems while avoiding many of their pitfalls. They often enable higher yields for specific types of proteins, particularly when expressing larger proteins that require complex folding.
Applications of Recombinant Proteins
Recombinant proteins have vast applications across various fields, particularly in medicine, industry, and research. The ability to produce specific proteins through recombinant expression has revolutionized how biotechnological processes are approached. This section delves into important applications, offering insight into their roles and significance in advancing this technology.
Biomedical Applications
The biomedical sector is one of the primary beneficiaries of recombinant proteins. These proteins play a critical role in the development of therapies and vaccines that save lives and improve health.
Therapeutic Proteins
Therapeutic proteins, such as insulin and monoclonal antibodies, are pivotal in treating diseases. Their importance stems from their ability to mimic naturally occurring proteins, which can restore normal physiological function. Insulin, for example, is essential for controlling blood sugar levels in diabetic patients. The production of human insulin through bacteria showcases a significant advancement in pharmacotherapy.
One key characteristic of therapeutic proteins is their specificity. They are designed to target particular pathways or cells, minimizing side effects. This specificity makes them a popular choice for treatments. However, the production process can sometimes lead to challenges, like protein misfolding, which can impact efficacy. Thus, while therapeutic proteins have significant advantages, they also come with complexities that require ongoing research and optimization.
Vaccine Development
Vaccine development is another critical area where recombinant proteins are utilized. They allow for the creation of safe and effective vaccines without the need to grow live pathogens, reducing the risk of disease transmission. For instance, recombinant proteins can be used as antigens, stimulating an immune response without causing illness.
A key characteristic of vaccine development through recombinant methods is adaptability. Techniques allow for rapid modification of vaccine components in response to emerging diseases, as demonstrated in recent COVID-19 vaccine development efforts. However, reliance on this technology can be seen as a disadvantage in terms of production costs and time, especially in large-scale manufacturing.
Industrial Applications
Recombinant proteins have substantial uses in various industrial applications, contributing significantly to fields such as agriculture, food production, and environmental management.
Enzyme Production
One significant application of recombinant proteins is in enzyme production. Enzymes produced through recombinant DNA technology are utilized in several industries, including food processing, textiles, and biofuels. They enhance efficiency and performance in reactions, leading to reduced waste and cost savings.
A key characteristic of recombinant enzymes is their enhanced activity or specificity compared to their natural counterparts. This trait makes them highly desirable in industrial processes. Nonetheless, challenges in integrating these enzymes into existing processes can arise, necessitating careful consideration and adjustment.
Bioremediation
Bioremediation is an innovative application wherein recombinant proteins are used to detoxify polluted environments. Microorganisms engineered to express specific enzymes can degrade harmful substances, such as heavy metals or petroleum products. This method is crucial for environmental restoration efforts.
The unique feature of bioremediation is its ecological advantage. Using recombinant proteins minimizes chemical usage and reduces the impact on the environment. However, reliance on genetically modified organisms poses regulatory and public perception challenges that can affect implementation.
Research and Development
Recombinant proteins are not only essential in application areas but also play a vital role in research and development. They provide valuable tools for understanding biological processes and discovering new therapies.
Protein Structure Analysis
One key area in research is protein structure analysis. Recombinant proteins can be produced in large amounts, making them available for studies aimed at determining three-dimensional structures. This information is crucial for drug design and understanding disease mechanisms.
A key characteristic of recombinant proteins in this context is their uniformity. This consistency simplifies the analysis process, leading to more reproducible results. Despite this advantage, challenges exist in terms of stability and solubility, which can affect the insights gained from structural studies.
Functional Studies
Functional studies of proteins are an important application of recombinant technology. Understanding the roles of proteins in biological systems helps elucidate mechanisms of disease and informs therapeutic strategies. Recombinant proteins provide a way to investigate functional characteristics that are difficult to study in native contexts.
The unique feature of functional studies is the ability to manipulate protein expression and observe changes in cellular behaviour. This adaptability offers significant benefits for research. However, the accuracy of these studies can be impacted by the organism used for expression, requiring careful selection of systems to ensure relevant findings.
Recombinant proteins are key players across biotechnology sectors, highlighting their significance in applications that span from medicine to environmental science.
Challenges in Recombinant Expression
The process of recombinant expression is not without hurdles. Various factors influence how successfully proteins can be produced in host cells. Addressing these challenges is essential for optimizing yield, ensuring functionality, and minimizing production costs. This article section highlights three principal challenges: protein misfolding and aggregation, low yield issues, and cost considerations. Understanding these aspects provides insights into the complexities involved in recombinant protein production.
Protein Misfolding and Aggregation
One of the most significant obstacles in recombinant expression is protein misfolding. Proteins must attain specific three-dimensional structures to perform their biological functions properly. When produced in host cells, sometimes they do not fold correctly. Misfolded proteins can lead to aggregation, which can inhibit product yield and activity. This aggregation often results in insoluble protein deposits, referred to as inclusion bodies. These bodies must be solubilized and refolded, however, achieving correct refolding is challenging and can vary based on the protein.
Proper folding of proteins is essential for their functionality and overall stability. Misfolding can severely affect their therapeutic efficacy and increase production costs due to additional purification steps needed to remove aggregates.
Low Yield Issues
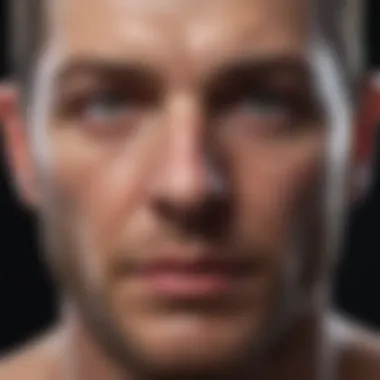
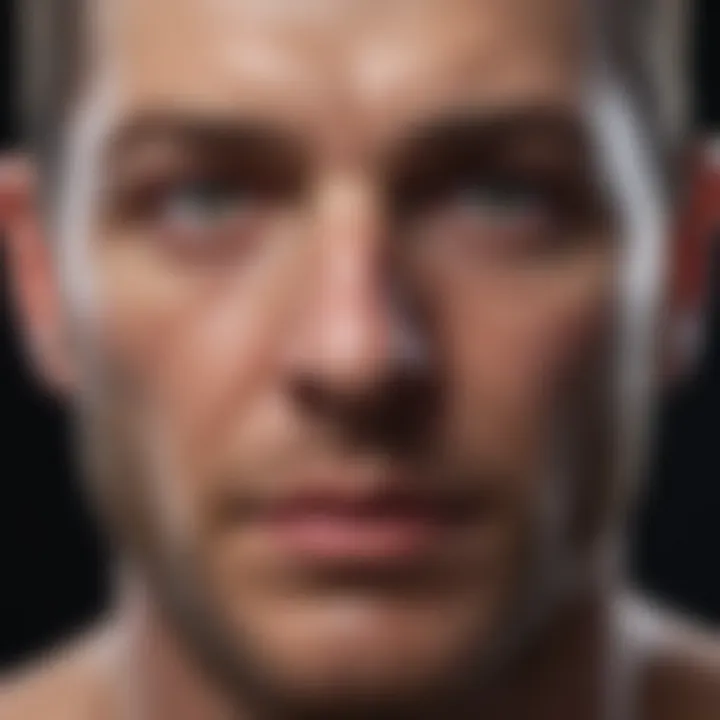
Low yield of recombinant proteins is a major concern for researchers and industrial producers alike. Several factors influence protein yield, including:
- Choice of expression system: Prokaryotic systems like E. coli may produce proteins rapidly but often at lower complexity. Eukaryotic systems, such as yeast or mammalian cells, can yield higher quality proteins but may have slower growth rates.
- Induction conditions: Over-expression of proteins can sometimes toxic to host cells, leading to reduced growth and lower yields.
- Post-translational modifications: Many proteins require modifications after synthesis to become active. If these modifications are not correctly added, functionality declines, leading to wasted production efforts.
Addressing yield issues requires a balance between selecting the right host system and optimizing culture conditions. Researchers often experiment with different variables to enhance productivity.
Cost Considerations
The economic aspect of recombinant expression cannot be overlooked. Production costs can rise substantially due to multiple factors:
- Resource intensiveness: Culturing cells, maintaining sterile environments, and obtaining purification reagents can be expensive.
- Time consumption: Long culture times and complex purification processes increase labor and operational costs.
- Scale-up challenges: Transitioning from small-scale production to large-scale industrial processes introduces additional costs and risks.
These challenges suggest that while recombinant expression holds great promise, it is essential to strike a balance between achieving high-quality products and managing costs effectively. Innovations in automation, high-throughput screening, and development of cost-efficient vectors could help alleviate some of these financial burdens.
Being aware of these challenges allows researchers and professionals to strategize more effectively, ultimately leading to improved outcomes in the field of recombinant expression.
Innovative Solutions and Future Directions
Innovative solutions play a crucial role in shaping the future landscape of recombinant expression. This evolving field is marked by developments in technology and methodology that aim to enhance the efficiency, scalability, and precision of protein production. The integration of advanced techniques like gene editing and synthetic biology improves our ability to design and manipulate biological systems for desired outcomes. Moreover, automation and high-throughput methods address the need for speed and efficiency in research and production settings. Understanding these innovative approaches is vital for anyone involved in biotechnology.
Gene Editing Technologies
CRISPR-Cas9
CRISPR-Cas9 stands out as a revolutionary gene editing tool with wide-reaching implications for recombinant expression. Its key characteristic is simplicity and precision in targeting specific DNA sequences. The ability to introduce changes at exact genomic locations allows for the creation of highly specialized expression systems. This specificity reduces off-target effects, making CRISPR-Cas9 a popular choice for many researchers.
One unique feature of CRISPR-Cas9 is its versatility. It can be applied across various organisms, from bacteria to plants to animals. However, its disadvantages include potential ethical concerns and regulatory hurdles that might complicate its application in certain areas of research and medicine.
TALENs
Transcription Activator-Like Effector Nucleases, or TALENs, provide another alternative for gene editing in recombinant expression. The key characteristic of TALENs lies in their ability to offer a more tailored approach to gene targeting compared to traditional methods. By designing specific TAL effectors, researchers can efficiently create double-strand breaks in DNA, facilitating the introduction of desired gene modifications.
TALENs possess unique features that allow them to be highly effective in a variety of systems, similar to CRISPR-Cas9. However, they also have disadvantages such as higher complexity in design and potential issues with delivery to the target cells, which can limit their accessibility for some researchers.
Synthetic Biology Approaches
Metabolic Engineering
Metabolic engineering significantly contributes to enhancing recombinant protein production. This approach focuses on modifying biological pathways in microorganisms to optimize the yield of desired products. Its key characteristic is its ability to redesign metabolic networks for improved performance in producing proteins, thus increasing productivity.
Metabolic engineering is a beneficial choice because it allows scientists to tailor the metabolic pathways of host cells used in protein expression, making them more efficient. One unique feature is the capability to integrate synthetic circuits into the microbial genome, allowing fine control over expression levels. However, there can be challenges like unintended metabolic burden and regulatory concerns in modified organisms.
Pathway Optimization
Pathway optimization is closely related to metabolic engineering and aims to refine the existing pathways for enhanced protein production. This method focuses on identifying bottlenecks in the metabolic flux and addressing these issues for better yield. Its key characteristic is precision; by optimizing specific enzymes or pathways, researchers can significantly elevate production rates.
The importance of pathway optimization cannot be downplayed as it helps in maximizing the potential of host systems in producing recombinant proteins. A unique feature of this approach is its capability to synergize with other techniques, such as gene editing. However, achieving this level of optimization demands extensive research and validation, which can require considerable time and resources.
Automation and High-Throughput Methods
Automation and high-throughput methods represent a transformative direction in the field of recombinant expression. By implementing robotic systems and automated workflows, laboratories can dramatically increase their experimental throughput while minimizing human error. This enhancement in efficiency leads to faster data acquisition and protein production.
Using high-throughput screening techniques allows researchers to evaluate numerous candidates systematically, leading to improved decision-making and optimization strategies. Automation in this context is more than just a tool; it is a necessary evolution in the competitive biotechnology landscape.
"Innovative approaches such as automation not only enhance scale but also ensure reproducibility in experiments, making them essential for modern research."
In summary, the focus on innovative solutions and future directions underscores the potential for recombinant expression to evolve continuously. Through gene editing technologies like CRISPR-Cas9 and TALENs, as well as synthetic biology tools like metabolic engineering and pathway optimization, the field is poised to overcome challenges. Automation further amplifies these advancements, making the future of recombinant expression promising and dynamic.
Finale
In synthesizing the intricate components of recombinant expression, this article underscores its significance in modern biotechnological advancements. The ability to produce proteins through recombinant techniques is not merely a scientific curiosity; it has profound effects on healthcare, research, and industry. As various methods of recombinant expression have been explored, it becomes clear that each approach carries distinct benefits and challenges. Understanding these nuances enables professionals, researchers, and educators to navigate the complexities of this field more effectively.
Summary of Key Points
- Importance of Recombinant Expression: It is vital in the production of therapeutic proteins, enzymes, and vaccines, bridging gaps in various sectors from healthcare to environmental science.
- Techniques Discussed: The article reviews gene cloning techniques, vector systems, and regulatory mechanisms that facilitate effective recombinant expression.
- Types of Systems: Different systems—prokaryotic, eukaryotic, and insect cell systems—are outlined, each with unique advantages.
- Applications: Applications span from clinical therapies to industrial uses, showcasing the versatility of recombinant proteins in meeting contemporary challenges.
- Challenges and Solutions: Issues like protein misfolding, low yield, and cost considerations are addressed, with innovative solutions highlighted for overcoming these obstacles.
The Future of Recombinant Expression
Looking ahead, recombinant expression is poised to evolve significantly, driven by cutting-edge gene editing technologies such as CRISPR-Cas9 and TALENs. These tools not only enhance precision but also expand the possibilities for protein engineering. Furthermore, the field of synthetic biology is advancing rapidly, focusing on optimizing metabolic pathways, which could lead to more efficient production systems.
Automation and high-throughput methods are likely to streamline the production process, enhancing both scalability and cost-effectiveness. The convergence of these developments will make recombinant expression more accessible and effective, transforming its role in research and application moving forward.
Innovation is key to addressing current limitations and unlocking new potentials in recombinant technology, paving the way for future breakthroughs in medicine and industry.
As the landscape of biotechnology continues to shift, staying informed about these trends will be essential for those involved in scientific discipline. The dynamic nature of recombinant expression signifies its lasting impact and relevance in the forthcoming years.