Exploring the Impact of Methane-Eating Microbes
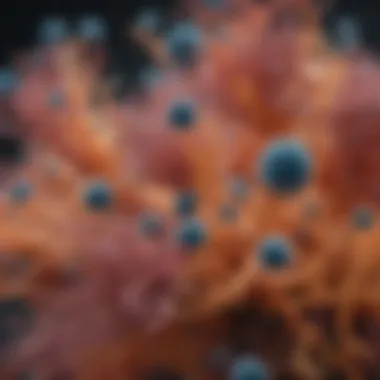
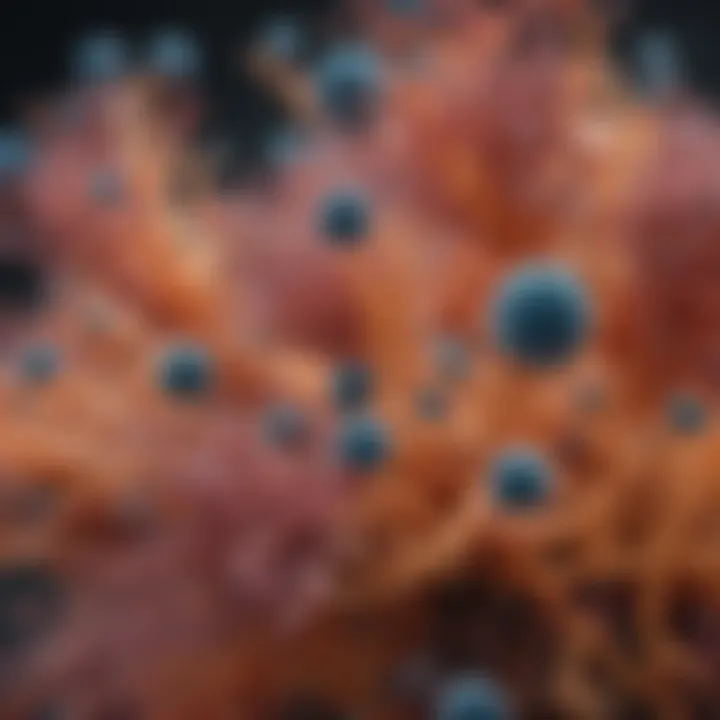
Intro
Methane-eating microbes are tiny organisms that play a crucial role in the ecosystem. They dramatically influence the methane cycle, helping to mitigate greenhouse gas emissions. This article explores the biology and ecology of these unique microbes, along with their potential contributions to addressing climate change.
Methane is a potent greenhouse gas, more effective than carbon dioxide in trapping heat in the atmosphere. The involvement of microbes in the breakdown of methane is a key factor in reducing its levels. Understanding their functions and relationships within ecosystems offers insights into sustainable solutions for our climate issues. We will discuss recent research, biological pathways, and the current scientific discourse surrounding these microorganisms.
Foreword to Methane Eating Microbes
Methane eating microbes, also known as methanotrophs, play a critical role in controlling methane emissions in the environment. Understanding these microorganisms is essential not only for recognizing their ecological impact but also for addressing climate change. Methane is a potent greenhouse gas, and its reduction is necessary for sustainable environmental management. By exploring methanotrophs, we uncover their biological processes, ecological interactions, and potential applications in bioremediation and agriculture.
Defining Methanotrophs
Methanotrophs are a group of bacteria that thrive on methane as their sole carbon and energy source. They can oxidize methane, transforming it into carbon dioxide and biomass. This metabolic capability is significant, as it mitigates methane emissions which are a major contributor to global warming. Methanotrophs fall into two primary categories: Type I and Type II. Type I methanotrophs utilize the ribulose monophosphate pathway for methane oxidation, while Type II methanotrophs use the serine pathway. These differences in metabolic pathways indicate diverse ecological strategies and adaptations.
Historical Context
The research on methanotrophs began in the 20th century, with significant milestones achieved from the 1970s onwards. Initial studies focused on their detection in different environments, particularly in soils and aquatic systems. As methanotrophs gained attention, scientists began to understand their roles in the carbon cycle. Discoveries like the effective methane oxidation in wetlands and rice paddies highlighted the importance of these microbes in natural ecosystems. Nowadays, studies emphasize the potentials of methanotrophs in biotechnological applications, leading to innovations in environmental management and energy production.
"Methanotrophs serve as nature's mechanism for reducing methane, making them an essential component of climate remediation strategies."
The Biology of Methane-Eating Microbes
The biology of methane-eating microbes plays a crucial role in understanding their function within ecosystems. By investigating their cellular structure and metabolic processes, we can appreciate how these organisms contribute to environmental stability and the reduction of greenhouse gases. Methanotrophs, the primary microbes responsible for methane consumption, possess unique cellular characteristics that enhance their survival in specific habitats. Exploring these aspects not only enriches our knowledge of microbial life but also informs strategies for climate change mitigation.
Cell Structure and Metabolism
Cellular Composition
Cellular composition of methanotrophs is distinctive, marked by the presence of specialized structures. The presence of intracellular membranes is a key characteristic, allowing these microbes to compartmentalize metabolic reactions effectively. These structures optimize the conversion of methane into carbon dioxide and water, showcasing a vital aspect of their contribution to carbon cycling.
In addition, methanotrophs have unique cell membranes that contain lipids suited for extreme environments, enabling them to thrive in niches with high levels of methane. This adaptability is beneficial for their role in combating climate change, as it allows them to flourish in diverse ecosystems.
Metabolic Pathways
Metabolic pathways of methanotrophs are intricate and efficient. They primarily utilize methane as their carbon source, transforming it through oxidation processes. The key characteristic here is their ability to convert methane into methanol and then further into methanediol and eventually into carbon dioxide. This pathway is advantageous because it not only helps in reducing atmospheric methane but also offers metabolic energy for the microbes.
Unique features of these pathways include the presence of methane monooxygenase, an enzyme crucial for initiating the oxidation of methane. This enzymatic action is valuable as it opens pathways for further metabolic reactions, contributing to their effectiveness in mitigating methane emissions.
Genetic Makeup
Genomic Insights
Genomic insights into methanotrophs reveal much about their evolutionary history and functional capabilities. The genomes of these organisms often exhibit genes that support methane metabolism, underscoring the adaptation this group has undergone to thrive in methane-rich environments.
A notable characteristic is the presence of specific plasmids that carry genes for enzymes involved in methane oxidation. This genetic redundancy provides a survival advantage, allowing these microbes to adjust to varying environmental conditions, making their study beneficial for biotechnology applications.
Evolutionary Adaptations
Evolutionary adaptations of methanotrophs play an integral role in their ecological success. These microbes have evolved various physiological traits enhancing their efficiency in converting methane. A key characteristic is their varying metabolic pathways, which allow them to use alternate substrates when methane levels are low.
This adaptability is a great asset as it offers resilience in changing environments. Furthermore, understanding these adaptations can aid scientists in designing more effective biotechnological applications aimed at greenhouse gas reduction.
Ecological Role of Methanotrophs
Methanotrophs play a significant role in the ecosystem by metabolizing methane, a potent greenhouse gas. Understanding their ecological role is crucial for identifying potential strategies to mitigate climate change. These microbes are crucial in maintaining the balance of the carbon cycle, acting as natural regulators. They convert methane emitted from various sources into carbon dioxide, which is less harmful contributing to carbon stocking in the environment.
Their functions extend beyond carbon cycling; they also influence soil and aquatic quality. By breaking down methane and other compounds, these microbes create a synergy that enhances nutrient cycling, influencing plant growth and microbial diversity. This complex interaction within ecosystems reveals the multifaceted importance of methanotrophs in our environment.
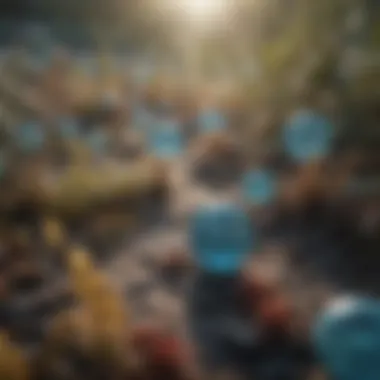
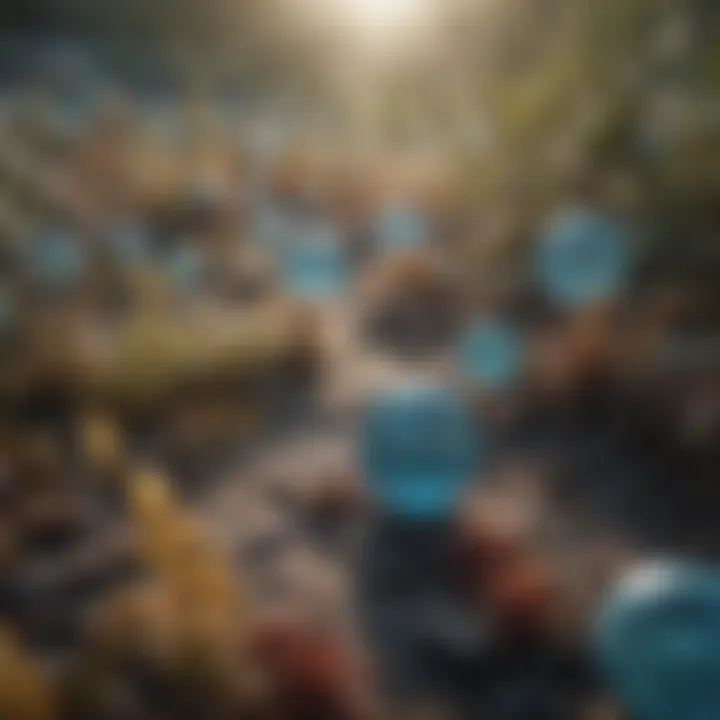
Habitats and Distribution
Soil Environments
Soil environments serve as critical habitats for methanotrophs. These unique niches harbor diverse microbial communities that utilize methane available from decaying organic matter. The distinction of soil as a habitat lies in its stratification and varying moisture levels, which create a favorable condition for methanotrophic activity.
Key characteristic: Soil provides a rich source of organic carbon and nutrients that facilitate methanotroph growth. Their presence in soil contributes not only to the reduction of methane emissions but also to improving soil health. However, variations in soil composition can impact their efficiency, thus influencing overall microbial dynamics.
Unique feature: The ability to thrive in different soil types shows their adaptability. The disadvantage, however, is that not all soils are conducive for effective methanotrophic activity, limiting their global impact in certain areas.
Aquatic Ecosystems
Aquatic ecosystems also support methanotroph populations, particularly in environments like wetlands and ocean floors. These ecosystems act as significant sinks for methane, emitted by both natural and anthropogenic sources. Methanotrophs in aquatic systems utilize dissolved methane, contributing to the methane oxidation in water bodies.
Key characteristic: The interactions occurring in these systems are crucial, as they involve complex microbial networks. Wetlands, for example, contribute on substantial methane emissions but are also sites of enhanced methane oxidation due to the presence of methanotrophs.
Unique feature: The inherent nutrient-rich environment of aquatic ecosystems fosters methanotroph diversity. A challenge remains, though. Their ability to perform in fluctuating salinity and temperature conditions may limit them, impacting the overall methane mitigation potential in these ecosystems.
Interactions with Other Organisms
Symbiotic Relationships
Symbiotic relationships are a notable interaction category wherein methanotrophs or mutualistic associations with other organisms enhance both partners' success. This relationship often benefits plants, which gain nutrients as methanotrophs break down methane and organic material. These interactions boost overall soil fertility and stability.
Key characteristic: Methanotrophs contribute nitrogen, a nutrient that enhances plant growth, showcasing their ecological value. Such synergies result in richer biodiversity, benefiting ecosystem resilience.
Unique feature: The mutualistic association directly impacts agricultural practices, promoting sustainable farming methods. However, the dependency on specific partners may create vulnerabilities, especially as ecosystem dynamics change.
Biodiversity Impacts
Biodiversity impacts from methanotrophs manifest in different ways, primarily regarding their role in community structuring. These microbes influence the richness of other microbial communities through their metabolic activities, contributing to a balanced ecosystem. They help in maintaining species diversity by allowing a variety of organisms to thrive.
Key characteristic: The preservation of biodiversity is crucial, as various bacterial and fungal species rely on the ecological niches created by methanotroph activity. They indirectly shape communities by affecting resource availability.
Unique feature: They can promote or inhibit the growth of competitors and other microbial populations, affecting community dynamics significantly. A downside here is that drastic environmental changes can drastically disrupt these balances, affecting the overall ecosystem health.
Factors Affecting Methanotrophic Activity
Understanding the factors that affect methanotrophic activity is crucial in grasping how these microbes impact the environment and the global carbon cycle. Methanotrophs play a vital role in consuming methane, thus mitigating its harmful effects as a greenhouse gas. Various external and internal factors influence their metabolic rates and population dynamics. These factors include environmental influences such as soil moisture and temperature variability, in addition to nutrient availability like nitrogen, phosphorus, and organic carbon sources. By examining these elements, we can better understand how to manage and utilize methanotrophic bacteria effectively, particularly in the context of climate change mitigation.
Environmental Influences
Soil Moisture
Soil moisture is one of the primary environmental factors influencing methanotrophic activity. The availability of water in the soil directly affects microbial metabolism. In particular, methanotrophs require an optimal range of moisture to thrive; too much or too little can inhibit their functions. The key characteristic of soil moisture is its capacity to maintain a thriving microbial community. When soil is adequately moist, methanotrophs can effectively utilize the oxygen necessary for their aerobic oxidation of methane.
However, there are advantages and disadvantages to consider. On the upside, optimal soil moisture enhances gas exchange and overall microbial health. On the downside, saturated soils can lead to anaerobic conditions where methanotrophs may become less active or even inhibited.
Temperature Variability
Temperature variability significantly impacts methanotrophic activity, influencing both their growth and metabolic rates. Generally, methanotrophs prefer moderate temperatures and can become less effective at consuming methane at extremely high or low temperatures. A key feature of temperature variability is its effect on the enzymatic reactions that drive methanotrophic metabolism. Enzymes function optimally within specific temperature ranges, and deviations can lead to reduced efficiency of methane degradation.
This factor is beneficial for our understanding as it provides insights into how climate change may alter methanotrophic dynamics in different ecosystems. If temperatures rise, the increased metabolic rates can lead to higher methane consumption, yet prolonged high temperatures may stress the microbial populations. Thus, managing temperature variability is key for promoting methanotrophic activity in various habitats.
Nutrient Availability
Nitrogen and Phosphorus Levels
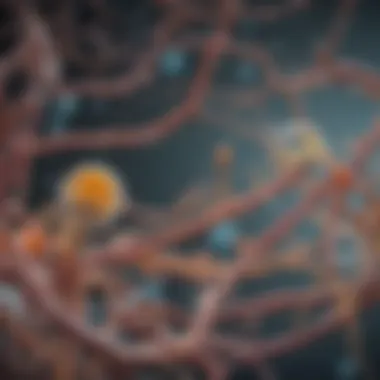
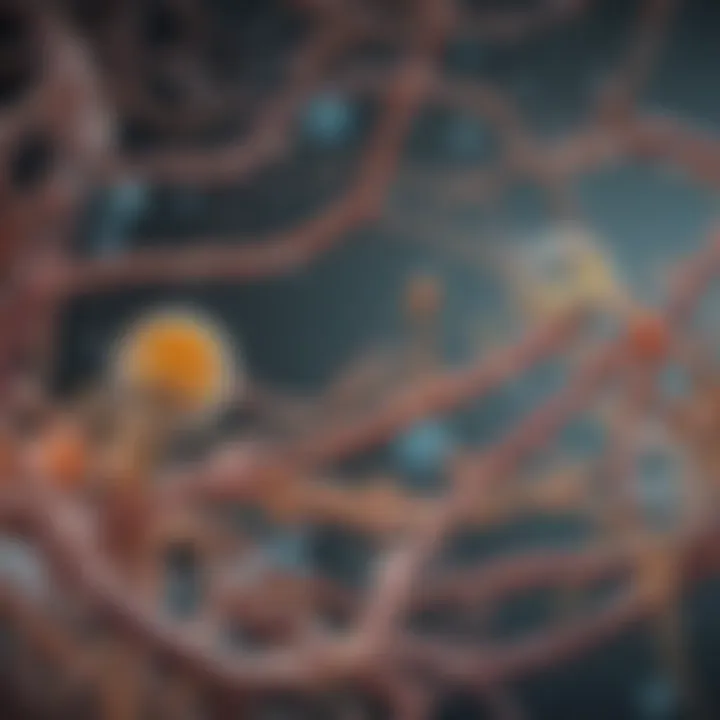
Nutrient availability, particularly nitrogen and phosphorus levels, is crucial for maintaining methanotrophic populations. Methanotrophs require nitrogen to synthesize proteins and nucleic acids, which are essential for their growth and reproduction. Phosphorus plays a critical role in energy transfer and cellular function. These two nutrients act synergistically to enhance microbial activity. If nitrogen and phosphorus are scarce, methanotrophic activity can decline.
Focusing on nitrogen and phosphorus levels can lead to more effective bioengineering strategies aimed at enhancing methane digestion in various environmental settings. Optimizing these nutrients can stimulate methanotroph communities, thereby increasing their capacity to consume methane and leading to greater carbon mitigation.
Organic Carbon Sources
Organic carbon sources also significantly affect methanotrophic activity. Methanotrophs can utilize various organic compounds as carbon sources for growth, which positively influences their metabolic processes. An essential characteristic of organic carbon sources is the diversity they offer. Different organic compounds may lead to varying pathways for methane oxidation, enriching microbial communities and enhancing their resilience.
The availability of organic carbon serves as both an advantage and a consideration in methanotroph research. On one hand, sufficient organic carbon can promote bacterial growth and methane consumption. On the other hand, excessive organic matter can lead to competition with other microbial communities, potentially restricting the effectiveness of methanotrophs. Understanding this balance is vital to optimizing their roles in methane mitigation efforts.
Research Advances in Methanotroph Studies
The investigation of methane-eating microbes, particularly methanotrophs, has seen notable advancements in recent years. These studies are crucial not just for understanding the biology and ecology of these organisms, but also for leveraging their abilities in practical applications. As global focus shifts towards mitigating climate change, research on methanotrophs becomes increasingly vital. The multi-faceted aspects of their biology, ecology, and potential are being examined in more depth than ever before, providing insight into their role in the carbon cycle and their potential for innovative environmental solutions.
Innovative Culturing Techniques
Culturing methanotrophs has historically posed challenges due to their specific growth conditions. Recent advances have led to the development of innovative culturing techniques that enhance the ability to isolate and study different methanotrophic strains. Techniques such as microfluidics allow for better control of environmental variables like pH, temperature, and methane concentration. This improved culturing can facilitate the study of previously uncultured methanotrophs, thereby expanding our understanding of their metabolic capabilities and ecological roles.
Genetic Engineering Applications
Advancements in genetic engineering have opened new doors for methanotrophy research, enabling scientists to manipulate the genetic makeup of these organisms for better efficiency in methane consumption.
CRISPR Technology
CRISPR technology represents a powerful tool in the field of genetic engineering. Its key characteristic is its ability to target specific sequences in DNA with high precision and create modifications. This precision has made CRISPR a popular choice for researchers studying methanotrophs. By using CRISPR, scientists can enhance or alter specific metabolic pathways in these microbes, potentially improving their ability to degrade methane. One unique feature of CRISPR is how it allows for multiplexing, meaning multiple genes can be edited simultaneously. This offers a significant advantage for research, although it also comes with concerns regarding off-target effects that could complicate results.
Synthetic Biology Applications
Synthetic biology takes the principles of biology and engineering to design and construct new biological parts or systems. In the context of methanotrophs, synthetic biology enables the design of microbes tailored for specific purposes, such as biofuel production or enhanced methane oxidation. The flexibility of synthetic biology is a notable feature; researchers can integrate pathways from various organisms to create novel functionalities. However, this approach also raises ethical considerations concerning the release of engineered organisms into the environment. The balance of risk and reward becomes essential in weighing synthetic biology's application in methanotroph studies.
Methane Mitigation Strategies
Methane mitigation strategies are crucial in addressing the increasing emissions of methane, a potent greenhouse gas. This section explores how methane-eating microbes can play a significant role in these strategies. Understanding the methodologies that utilize these microorganisms provides insight into their potential applications in environmental management and climate change mitigation.
Bioremediation Efforts
Bioremediation efforts involve using living organisms to remove or neutralize pollutants from the environment. Methanotrophs, the methane-eating microbes, are particularly important in this context. They can degrade methane, transforming it into less harmful substances and integrating it back into the carbon cycle. Their capability to consume methane in various settings makes them valuable in bioremediation.
The significance of these microbes lies in their ability to thrive in diverse environments, such as wetlands and landfills, where methane is prevalent. This adaptability makes them a preferred choice for remediation strategies aimed at reducing greenhouse gas emissions. However, one challenge is ensuring that these microbes have the appropriate conditions to function effectively, as their activity can be influenced by environmental factors.
Integration into Agricultural Practices
Integrating methane-eating microbes into agricultural practices showcases a proactive approach to reducing methane emissions. This integration is beneficial for both crop productivity and climate health. It explores specific aspects like soil management and plant-microbe interactions.
Soil Management
Soil management involves the practices of maintaining and improving soil health. By enhancing soil microbiome diversity, which includes methanotrophs, farmers can promote the natural degradation of methane gas in soil systems. One key characteristic of effective soil management is the incorporation of organic matter, which supports microbial activity.
This choice is beneficial as it improves soil fertility while simultaneously reducing methane emissions. The unique feature of soil management is its dual function: enhancing agricultural output and mitigating greenhouse gases. However, it requires careful planning and monitoring to ensure that interventions do not disrupt current ecosystems unnecessarily.
Plant-Microbe Interactions
Plant-microbe interactions describe the relationships between plants and their microbial partners, including methanotrophs. This cooperation can enhance plant growth and resilience while aiding in methane mitigation. A key characteristic of beneficial plant-microbe interactions is their ability to improve nutrient uptake, which can lead to healthier plants.
Employing these interactions is a popular choice within modern agricultural strategies due to their multifunctionality in promoting growth and reducing harmful emissions. The unique aspect of plant-microbe interactions is their potential to create synergies that lead to improved crop yields alongside a better environmental footprint. However, realizing these advantages necessitates careful management of agricultural practices to maintain balance in the soil ecosystem.
The Future of Methane-Eating Microbes in Technology
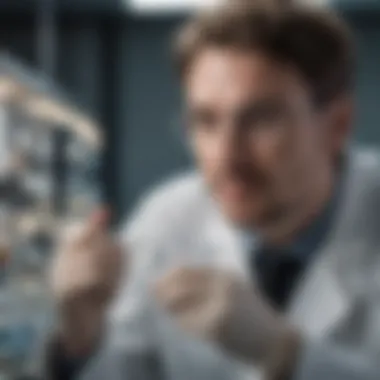
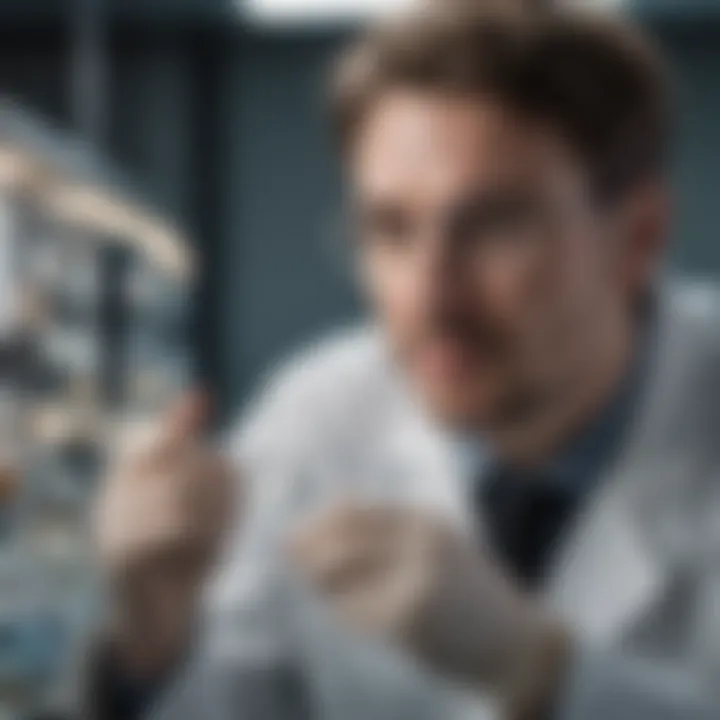
Methane-eating microbes represent a frontier in environmental technology and bioremediation strategies. Their unique capabilities to metabolize methane offer significant potential benefits in various industrial applications. These microbes could play a crucial role in addressing pressing environmental issues such as climate change and energy sustainability. By harnessing their natural functions, researchers aim to develop innovative approaches that can be integrated into existing technologies.
Potential in Biofuels Development
The growing interest in renewable energy sources makes methane-eating microbes increasingly relevant. These microbes can process methane, a potent greenhouse gas, into energy-rich compounds. This process can be enhanced to produce biofuels, which provide a cleaner alternative to fossil fuels. The conversion of methane to biofuels, such as bioethanol or biodiesel, involves complex biochemical pathways that these organisms navigate efficiently.
Here are some considerations regarding biofuel production from methanotrophs:
- Sustainability: Utilizing methane for biofuel production can help reduce greenhouse gas emissions.
- Cost-Effectiveness: Methane is often abundant and inexpensive, making it a viable feedstock for large-scale production.
- Energy Yield: Biofuels derived from methane-eating microbes may exhibit favorable energy outputs compared to traditional biomass sources.
Research into optimizing these processes is ongoing. The genetic manipulation of methanotrophic bacteria could improve their productivity and resilience, allowing for more efficient energy conversion.
"The potential of methanotrophs in biofuel development opens new pathways to combat energy challenges while also addressing environmental concerns."
Applications in Carbon Capture
In addition to biofuels, methane-eating microbes hold promise for carbon capture technologies. The ability of these microorganisms to absorb and convert methane can significantly mitigate carbon emissions from various sources, including landfills and agricultural activities. Through their metabolic activities, they can transform methane into less harmful byproducts, thereby curtailing its release into the atmosphere.
Key aspects of carbon capture applications include:
- Direct Methane Utilization: Implementing methanotrophic systems may enable direct capture of methane from emission sources.
- Enhanced Carbon Management: Integrating methanotrophs within existing carbon management frameworks can improve the overall efficacy of reduction efforts.
- Research and Innovation: Continued study of methane-eating microbes can lead to breakthroughs in carbon sequestration technologies, enabling a transition to greener alternatives.
The development of such technologies depends heavily on funding and interdisciplinary collaboration to advance research in this area.
Challenges in Methanotroph Research
The study of methane-eating microbes, particularly methanotrophs, is significantly beneficial for environmental science and climate change mitigation. However, research in this field faces several challenges that can hinder our comprehensive understanding of these microorganisms and their potential applications. Addressing these challenges can enhance our ability to leverage methanotrophs effectively in biotechnological advancements and ecological conservation efforts.
Limited Funding and Resources
One primary challenge in methanotroph research is the limited funding and resources available to scientists. This limitation restricts the extent of experiments and investigations, leading to slower progress in understanding the full biological capabilities of these microbes. Methanotroph research demands extensive resources for both field and laboratory work, which can deter potential funding sources.
- Many grants focus on more mainstream research areas, often leaving niche projects like those centered on methanotrophs underfunded.
- Institutions may prioritize popular research topics, causing researchers in this field to compete for scarce funds.
Without adequate financial support, researchers may struggle to conduct large-scale studies or develop innovative experimental designs, thereby delaying crucial findings that could contribute to the global climate agenda. Limited resources can also affect collaboration opportunities, essential for interdisciplinary approaches needed in ecological studies.
Data Gaps and Research Limitations
In addition to funding issues, significant data gaps exist within the current body of knowledge about methanotrophs. Many studies focus on specific species or isolated populations. This narrow focus often results in incomplete or biased data sets. The lack of comprehensive genomic and ecological data limits researchersโ understanding of the diversity and variability among methanotrophs.
Key constraints include:
- Under-researched Habitats: Not all habitats where methanotrophs thrive have been thoroughly explored. Many ecological niches still need to be studied to uncover how methanotrophs function in different environments.
- Methodological Challenges: Techniques for culturing methanotrophs can be complex, affecting data reliability and consistency. As a result, findings can sometimes be hard to replicate, leading to further confusion within the scientific community.
"Addressing data gaps and enhancing methodological rigor will accelerate innovation in methane mitigation strategies."
Furthermore, emerging technologies such as metagenomics and bioinformatics hold great promise for bridging these gaps. However, as seen in many fields, researchers may need training in these newer methods, which adds another layer of complexity to the advancements in methanotroph studies. Thus, overcoming these research limitations is crucial for advancing our understanding and application of methane-eating microbes in addressing climate change.
Ending
The study of methane-eating microbes, or methanotrophs, highlights their essential role in addressing climate change directly. By consuming methane, a potent greenhouse gas, these microorganisms contribute significantly to reducing atmospheric levels while offering insights into sustainable practices. This conclusion emphasizes the cumulative findings from earlier sections and positions methanotrophs as critical players in future environmental strategies.
Summarizing Key Insights
- Biological Function: Methanotrophs have unique metabolic pathways that allow them to convert methane into less harmful compounds. This capability makes them vital in the global carbon cycle.
- Ecological Importance: Their presence in diverse environments, from soils to aquatic systems, ensures that they play a major role in maintaining ecosystem balance. Interactions with other organisms enhance biodiversity and contribute to nutrient cycles.
- Mitigation Strategies: The exploration of innovative bioremediation techniques positions methanotrophs as a natural solution for reducing methane emissions from important sectors like agriculture and industry.
- Research and Development: Continued research into their genetic makeup and potential applications in bioengineering and climate strategies is essential for realizing the full benefits of these microbes.
- Future Potential: The adaptability of methanotrophs to varying environmental conditions suggests that they can be leveraged for future biotechnological applications, like carbon capture and biofuels production.
"Methanotrophs may hold the key to a sustainable future, offering solutions for a major environmental challenge."
The Path Forward
Addressing climate change requires a multifaceted approach. As methane-eating microbes gain prominence in environmental science and engineering, several steps should be considered:
- Increased Funding: Investing in methanotroph research can spur innovative solutions. Academic institutions and industries should collaborate to provide necessary resources.
- Public Awareness: Educating communities about the role of methanotrophs can enhance support for sustainable practices involving these microbes.
- Policy Support: Governments can include methanotrophic strategies in climate action plans. Regulatory frameworks could promote the use of bioremediation techniques that incorporate these organisms.
- Interdisciplinary Research: Scientists from various fields, like microbiology, ecology, and environmental science, should work together to fully understand and exploit the capabilities of methanotrophs.
- Technological Advancements: Further exploration into genetic engineering might unveil new methods to enhance the effectiveness of these microbes in various settings. Such innovations could transform how methane emissions are managed.
By committing to a path that integrates these microorganisms into our future strategies, we can address one of the most critical environmental issues of our time. The evolution of research and application surrounding methanotrophs may indeed play a pivotal role in shaping a sustainable world.