Understanding the Liquefaction of Hydrogen
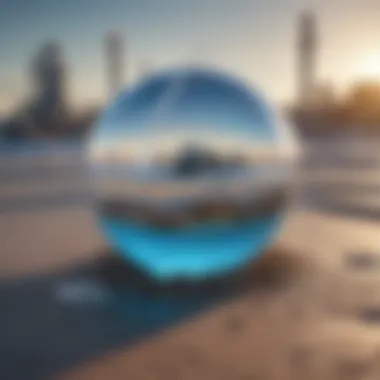
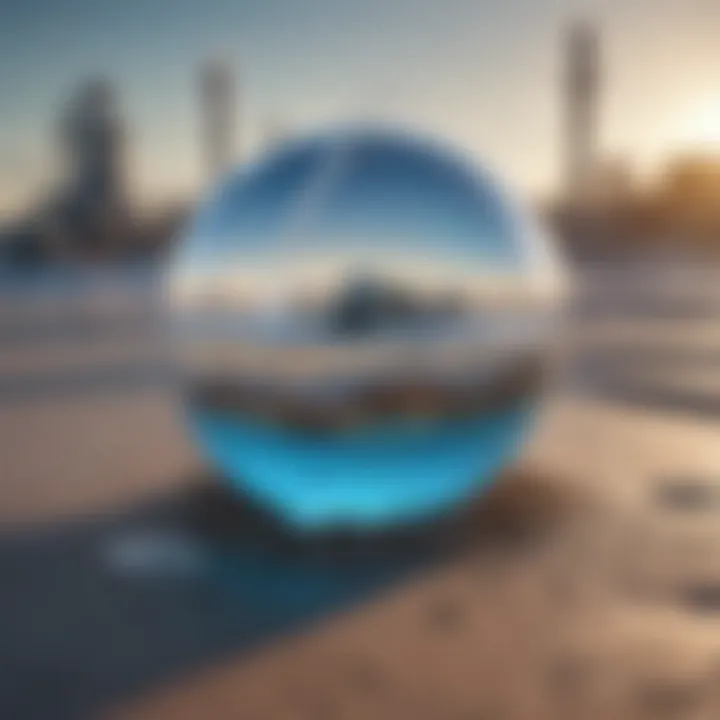
Intro
Hydrogen has emerged as a significant component in the pursuit of sustainable energy solutions. As the world seeks alternatives to fossil fuels, the importance of hydrogen becomes increasingly clear. This article explores the liquefaction of hydrogen, essential for several scientific and industrial applications. Understanding the liquefaction process provides insights into the applications of hydrogen as an energy carrier, the technology used for its processing, and implications for the environment and safety.
Research Overview
Summary of Key Findings
The liquefaction of hydrogen is essential due to its low volumetric energy density. In its gaseous state, hydrogen occupies a large volume, making transportation and storage inefficient. Liquefying hydrogen significantly reduces its volume, facilitating easier transport and utilization. Key findings highlight multiple technological methods employed in this process, most notably the Claude cycle and the Linde-Hampson process.
Additionally, research indicates that liquefied hydrogen plays a crucial role in various sectors, including aerospace, fuel cell technology, and energy storage systems. Its application in these areas can greatly enhance energy efficiency and feasibility.
Relevance to Current Scientific Discussions
The discussion surrounding hydrogen liquefaction aligns with global trends toward green energy and sustainability. Scientists and researchers are increasingly focusing on hydrogen as a clean fuel alternative. Examinations of the efficiencies and safety protocols related to hydrogen storage and transportation are crucial.
"Liquefied hydrogen represents a critical development in green energy technologies and presents new avenues for research and application."
Methodology
Research Design and Approach
In investigating the liquefaction of hydrogen, the research employs a multidisciplinary approach. It combines aspects of thermodynamics, materials science, and engineering. The focus is on the principles of cryogenic cooling used in liquefaction, alongside technological innovations that improve efficiency.
Data Collection and Analysis Techniques
Data on liquefaction methods was primarily gathered from scientific journals, patents, and energy reports. Comparative analysis was employed to evaluate different liquefaction technologies. This evaluation included performance metrics related to energy consumption, operational safety, and overall environmental impact.
Furthermore, case studies from existing industrial operations provided real-world applications of liquefaction technology. These insights are pivotal for understanding both current applications and future developments in the field of hydrogen energy.
Prelims to Hydrogen Liquefaction
The liquefaction of hydrogen is a crucial process that enables the effective handling and storage of hydrogen, a gas with significant potential in multiple sectors. Understanding this process is vital for students, researchers, educators, and professionals looking to explore the role of hydrogen in energy solutions, particularly in the shift towards cleaner energy alternatives.
Hydrogen is considered a clean fuel. When used, its only byproduct is water. This makes it an attractive option in combating climate change. However, storing and transporting hydrogen in its gaseous form can be inefficient and challenging, primarily due to its low density. Here, liquefaction becomes important. By converting hydrogen into its liquid state, we increase its density. This allows for easier storage and transportation.
Benefits of hydrogen liquefaction include:
- Higher energy density compared to gaseous hydrogen.
- Reduced volume for storage, which aids in logistics.
- Opportunities for integration into existing energy infrastructures.
Yet, liquidity must be achieved through complex cryogenic processes, which require careful consideration of thermodynamic principles. This article delves into those principles and outlines various methods utilized in liquefying hydrogen. It highlights their applications, such as fuel cells and rocket propulsion, promoting a deeper understanding of their role in innovative energy solutions.
The importance of comprehending hydrogen liquefaction extends beyond mere academic interest; it encompasses advances in technology and insights into sustainable energy practices. As industries continue to evolve, so does the understanding of hydrogen and its applications. Thus, the journey into hydrogen liquefaction holds not only academic merit but also facilitates future technological progress and environmental sustainability.
The Importance of Hydrogen
Hydrogen plays a transformative role in the emerging energy landscape. Its potential as a clean fuel source can help decrease reliance on fossil fuels. In addition, advanced research is focusing on hydrogen as a fundamental component of a sustainable energy economy. Its role in fuel cells, renewable energy systems, and industrial processes cannot be overlooked.
With ongoing global efforts to reduce carbon emissions, hydrogen acts as an adaptive solution across several sectors. The flexibility it offers is significant, from powering vehicles to being a feedstock for chemicals. Focusing on hydrogen fosters advancements in energy technology.
Overview of Liquefaction Processes
Liquefaction is the process of transforming hydrogen gas into a liquid state. Achieving this requires specific temperature and pressure conditions. Various methods exist to liquefy hydrogen, each with unique attributes and applications. Some of these processes include the Linde process and the Claude cycle. Each method utilizes principles of thermodynamics, such as refrigeration cycles.
The liquefaction process generally involves:
- Compressing hydrogen gas.
- Cooling it to very low temperatures.
- Allowing it to expand, thus lowering its temperature further.
In comprehending these processes, one can appreciate the efficiency gains in hydrogen storage and transportation. Knowledge of these liquefaction methods also lays the groundwork for addressing potential safety considerations in handling liquefied hydrogen.
Liquefaction aids in maximizing the potential of hydrogen as a key player in the sustainable energy future.
Physical Properties of Hydrogen
Understanding the physical properties of hydrogen is essential for comprehending its behavior during liquefaction. Hydrogen, as the lightest and most abundant element in the universe, presents unique characteristics that influence its phase transitions and processes. These properties include its molecular structure, thermodynamic attributes, and reactivity. A detailed study of these aspects can help optimize liquefaction methods, enhance efficiency in usage, and mitigate safety concerns related to hydrogen applications in various fields.
Molecular Characteristics
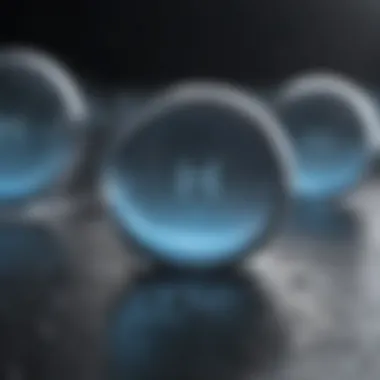
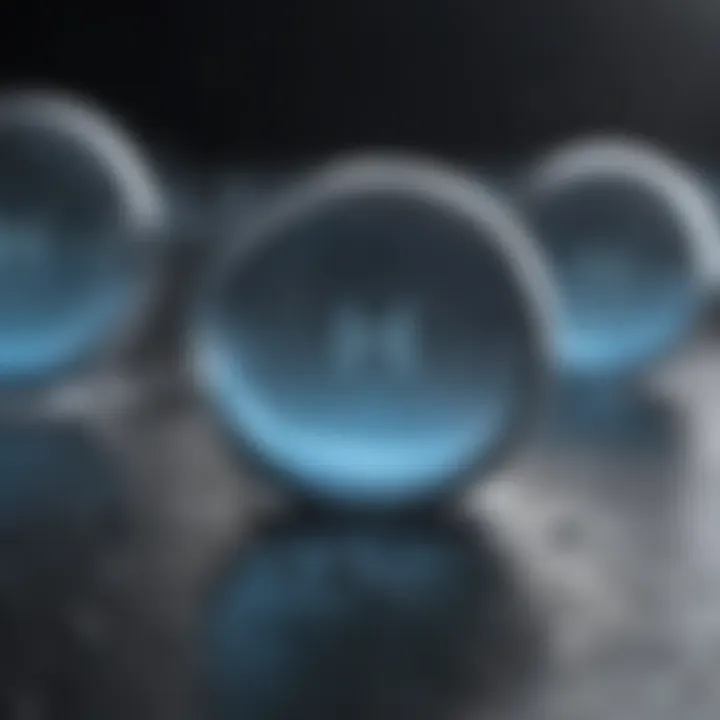
Hydrogen () exists as a diatomic molecule, presenting a simple yet significant molecular structure. One of the major elements influencing hydrogen's physical properties is its low molecular weight, which is about 2 g/mol. Compared to heavier gases, hydrogen has a much lower density, allowing for easier handling and transportation but also requiring careful management due to its high diffusivity.
In gas form, hydrogen molecules exhibit relatively weak intermolecular forces. This makes hydrogen highly reactive and capable of forming various compounds with other elements. The bond strength of is moderate, providing stability under normal conditions but becoming unstable under high energy or specific chemical reactions. Furthermore, hydrogen transitions seamlessly between gas and liquid states, making it crucial in processes that require state manipulation, such as liquefaction.
Thermodynamic Properties
The thermodynamic behavior of hydrogen is characterized by its unique heat capacity and thermal conductivity. Hydrogen has a high specific heat capacity compared to other common gases, which means it can absorb a significant amount of heat without a large change in temperature. This property plays an important role in liquefaction as it affects how hydrogen must be cooled and compressed for effective liquefaction.
Moreover, hydrogen has excellent thermal conductivity, which enhances its ability to dissipate heat quickly. This property can be both advantageous and disadvantageous in various applications related to liquefaction. For instance, while effective heat removal can expedite the liquefaction process, it can also lead to increased energy consumption if not managed properly.
Additionally, the critical temperature of hydrogen is approximately -240 degrees Celsius. This means hydrogen must be cooled below this temperature to reach its liquid state, highlighting the need for extensive energy input in liquefaction techniques. The ability to understand these thermodynamic properties allows for more informed decisions related to liquefaction methods and energy management.
"Understanding the molecular and thermodynamic properties of hydrogen is key to improving liquefaction processes and ensuring safe storage and transport."
By analyzing these physical properties, we can effectively grasp the challenges and strategies involved in hydrogen liquefaction. The insights gained offer a pathway to innovate and enhance current technologies for hydrogen handling and applications.
Principles of Liquefaction
The principles of liquefaction play a fundamental role in understanding how hydrogen transitions into a liquid state. This section covers the critical scientific concepts that underpin hydrogen liquefaction, facilitating its wide range of applications. Grasping these principles is essential for researchers and industry professionals seeking to enhance efficiency in liquefaction processes.
Cryogenics and Phase Transitions
Cryogenics involves the study of materials at extremely low temperatures. The liquefaction of hydrogen operates within this scientific domain, relying on two key processes to achieve liquid hydrogen from its gaseous state.
As hydrogen is cooled below its boiling point of 20.28 K (-252.87 °C), it undergoes a phase transition. This transition is integral because it allows hydrogen to be stored and transported more efficiently. In its gaseous form, hydrogen occupies significantly larger volumes, making it impractical for some applications. Liquefaction reduces the volume by a factor of about 800, enhancing storage capabilities and making it economically viable.
The cryogenic processes must be carefully controlled. The cooling must occur in stages, reducing the temperature gradually to avoid solidification. Any rapid temperature change can lead to inefficient energy use or damage to the equipment. Therefore, understanding the principles of thermodynamics is vital.
The Joule-Thomson Effect
The Joule-Thomson Effect is another critical principle in the liquefaction of hydrogen. This effect describes how the temperature of a gas changes when it is allowed to expand freely at constant enthalpy. More specifically, for hydrogen, this effect is vital during the liquefaction phase.
When hydrogen is compressed and subsequently allowed to expand, its temperature can decrease significantly, depending on the conditions. This property is exploited in liquefaction methods, where gases are compressed and then expanded to achieve low temperatures necessary for phase transition. The Joule-Thomson effect is particularly pronounced at cryogenic temperatures, which makes it essential in designing efficient liquefaction systems.
Understanding how this effect varies with temperature and pressure also influences the efficiency of hydrogen liquefaction. Researchers work on optimizing these conditions to minimize energy consumption and maximize output. Each liquefaction method, such as the Linde or Claude cycles, utilizes this principle to achieve the desired levels of hydrogen liquefaction.
In summary, the principles of liquefaction delineate how hydrogen transitions from gas to liquid. Cryogenics and the Joule-Thomson effect are central to these processes, representing critical areas where advancements can significantly impact the efficiency and utility of liquefied hydrogen.
Methods of Hydrogen Liquefaction
The methods of hydrogen liquefaction are crucial in achieving efficient, practical applications for hydrogen in various sectors. Understanding these methods provides insight into how hydrogen can be transformed from a gaseous to a liquid state, vastly improving its transport and storage capabilities. Each process shares common goals but employs distinct techniques to achieve liquefaction. Evaluating these processes highlights advantages and potential limitations associated with each.
Linde Process
The Linde process is one of the oldest and most widely used methods for liquefying hydrogen. It operates on the principle of continuous cycle refrigeration.
- Basic Principle: The Linde process uses a gas refrigerant that undergoes expansion in a turbine, cooling the gas due to the Joule-Thomson effect. Following this, the cooled gas is allowed to expand, resulting in the liquefaction of hydrogen.
- Advantages: This method is known for its energy efficiency and relatively simple design. It can also produce hydrogen in large quantities, supporting industrial demands.
- Features: However, it may require significant electrical power and complex heat exchange systems.
- Applications: It is commonly employed in industries dealing with hydrogen as a feedstock or fuel source, demonstrating the process’s real-world relevance.
Claude Cycle
The Claude cycle represents another established technique for hydrogen liquefaction, utilizing a different physical principle than the Linde process.
- Operating Principles: This method involves cooling hydrogen through a closed cycle. The process consists of compression, heat exchange, and expansion, leading to lower temperatures and the resultant liquefaction of hydrogen.
- Advantages: A notable benefit of the Claude cycle is its ability to achieve lower temperatures than the Linde process, making it effective for noble gases and lighter elements. It provides flexibility in use across various applications.
- Energy Efficiency: While more complex, its design integrates well with cascade refrigeration setups. This complexity can enhance the overall energy efficiency when done correctly.
- Industries: It is particularly beneficial in low-temperature applications, such as cryogenics or space exploration programs.
Modified Fritsche Process
The Modified Fritsche Process is a more recent advancement in liquefaction technology and reflects ongoing innovation in the field.
- Mechanism: This process fundamentally innovates upon earlier refrigeration methods by optimizing energy consumption and improving liquefaction rates. The method is characterized by its use of multiple stages of expansion and heat exchange to reach target temperatures necessary for liquefaction.
- Benefits: This approach can reduce energy costs and improve overall yield. As a newer technology, it often integrates modern advancements in engineering, making it more efficient than some older methods.
- Adaptability: It can be adapted to suit varying scales of production, making it valuable across both industrial and research settings.
- Future Prospects: Ongoing research and development may enhance its applications further in a world increasingly focused on hydrogen as a cleaner energy source.
Applications of Liquefied Hydrogen
Liquefied hydrogen plays a crucial role in various industries and scientific fields. Its unique properties, such as low density and high energy content, make it an attractive option for multiple applications. Understanding these uses is essential for advancing technologies related to energy, transportation, and more. This section delves into specific applications that highlight the benefits and considerations of liquefied hydrogen.
Fuel Cells
Fuel cells are among the primary applications of liquefied hydrogen. They convert chemical energy directly into electrical energy through an electrochemical reaction. In this process, hydrogen serves as a fuel, reacting with oxygen from the air. The result is electricity, water, and heat.
- Efficiency: Fuel cells can achieve high efficiency rates compared to traditional combustion engines. This efficiency is a key reason for their rising popularity in various sectors, including automotive.
- Emissions: Using liquefied hydrogen in fuel cells significantly reduces greenhouse gas emissions. They produce only water as a byproduct, making them cleaner alternatives.
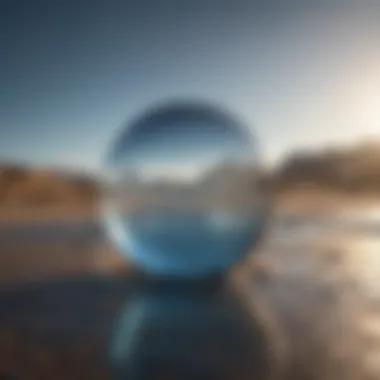
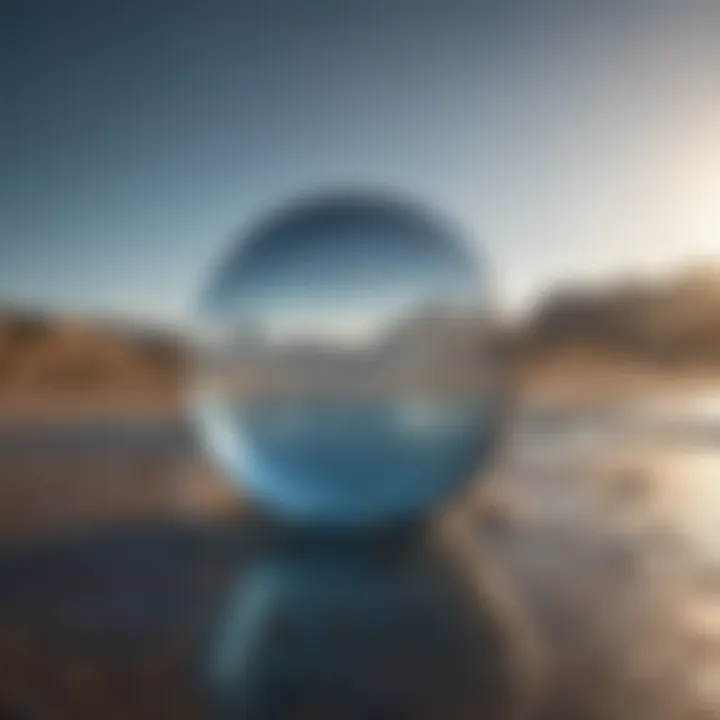
Yet, challenges exist. The production and storage of liquefied hydrogen must be managed responsibly. Production methods often involve fossil fuels, which can negate some environmental benefits. Hence, focusing on green hydrogen production becomes vital.
Rocket Propulsion
Rocket propulsion is another significant area where liquefied hydrogen is indispensable. It has been utilized in several space exploration initiatives, including the Space Shuttle program.
- High Specific Impulse: Liquefied hydrogen provides a high specific impulse when burned with liquid oxygen. This means rockets can achieve greater thrust with less fuel.
- Cryogenic Characteristics: The cryogenic nature of liquefied hydrogen allows it to be stored at very low temperatures, which is required for optimal performance in rocket engines.
However, handling liquefied hydrogen in space missions requires rigorous safety protocols. Any leaks or mishandling can lead to severe consequences, emphasizing the need for stringent safety measures in rocket design and operations.
Energy Storage Solutions
As renewable energy sources such as wind and solar gain traction, the need for effective energy storage solutions becomes paramount. Liquefied hydrogen offers a viable approach to balancing supply and demand in cleaner energy systems.
- Scalability: Liquefied hydrogen can be stored in large quantities, making it suitable for utility-scale energy systems. This scalability can help manage excess energy during peak production efforts and supply it during low production periods.
- Flexibility: It can be converted back to electricity in power plants or used directly in other applications, increasing its value within the energy ecosystem.
Despite its potential, challenges in storage technology and transportation remain. Developing better containment methods and reducing costs for liquefaction can enhance its broader adoption.
"The versatility of liquefied hydrogen positions it as a cornerstone for the future of sustainable energy and transportation."
Each application outlined above illustrates the vital role of liquefied hydrogen in today's technological landscape. As research and development continue, focusing on improving efficiency and sustainability will further solidify its place in various industries.
Safety and Environmental Considerations
The liquefaction of hydrogen is not merely a technical process; it involves significant safety and environmental factors that must be thoroughly understood and managed. As hydrogen becomes increasingly relevant in various sectors, its handling, storage, and transportation present unique risks. Addressing these hazards while capitalizing on the advantages of hydrogen is essential for its sustainable development and integration into clean energy solutions.
Hazards of Liquefied Hydrogen
Liquefied hydrogen, while essential in many applications, presents distinct hazards that need careful attention. One significant danger is flammability. Hydrogen can ignites easily when mixed with air in certain concentrations, leading to potential explosions. The flash point of hydrogen is around -252 °C, which is extremely low, making its handling particularly risky in open environments. Another hazard includes the potential for asphyxiation. In confined spaces, the density of hydrogen being lighter than air can result in it accumulating at high altitudes, displacing oxygen and creating an asphyxiation risk for individuals nearby. Furthermore, the low temperatures associated with liquefied hydrogen raise concerns about cold burns or frostbite upon contact with skin.
"Hydrogen has a high energy-to-weight ratio and can be a safer alternative to traditional fuels; yet, its risks must be managed meticulously."
Key Hazards:
- Flammability: Easily ignites when mixed with air.
- Asphyxiation: Can displace oxygen in confined spaces.
- Cold burns: Caused by exposure to cryogenic temperatures.
Mitigation Strategies
To ensure safety in scenarios involving liquefied hydrogen, several mitigation strategies can be implemented. Firstly, robust engineering controls are vital. This includes the use of proper containment systems, appropriate materials for piping that can handle cryogenic temperatures, and advanced monitoring systems to detect leaks or potentially dangerous conditions. Regular maintenance and inspections should be mandatories to ensure all equipment operates effectively and safely.
Training personnel extensively on the hazards and emergency protocols related to hydrogen is critical. Workers must be educated about the proper handling techniques and the specific risks associated with liquid hydrogen. Also, emergency response plans should be in place and well-practiced regularly to prepare for any incidents.
Additionally, employing atmospheric monitoring systems can alert staff to changing conditions that may signal the presence of hydrogen. Implementing passive safety systems, such as venting hydrogen into safe zones, can also reduce the risk of accumulation in critical areas.
Essential Mitigation Approaches:
- Robust engineering controls: Proper containment and materials.
- Regular training: For personnel on hazards and emergency procedures.
- Atmospheric monitoring: To detect potentially hazardous conditions.
- Emergency response plans: Preparedness drills to handle incidents effectively.
In summary, while liquefied hydrogen has considerable utility in energy applications, understanding and addressing safety risks is fundamental. Effective mitigation strategies not only enhance the safety of operations but also support the broader acceptance of hydrogen technologies in the energy landscape.
Future of Hydrogen Liquefaction
The future of hydrogen liquefaction is an area of considerable importance. As global energy demands rise, the need for efficient energy carriers becomes more critical. Liquefied hydrogen is emerging as a vital player in this energy landscape, offering advantages in both energy density and storage capabilities. Moreover, with increasing reliance on renewable energy sources, the capacity for hydrogen to act as a bridge for energy transition cannot be overstated.
Advancements in liquefaction technology promise to enhance the efficiency of hydrogen production and storage. As innovations progress, we may see significant reductions in energy consumption associated with liquefaction processes. These improvements are essential for making hydrogen a more economically viable option for various applications, especially in transportation and energy storage.
Another consideration is the integration of hydrogen liquefaction into existing infrastructure. This integration poses challenges but also presents economic opportunities. Establishing a reliable hydrogen supply chain will require collaboration across industries, from production to transportation to end-use applications.
The quest for improved technologies in hydrogen liquefaction is fundamental for achieving competitive energy solutions in a sustainable future.
Technological Innovations
Technological innovations play a crucial role in shaping the future of hydrogen liquefaction. Various cutting-edge techniques are currently under research. For instance, advancements in membrane technology show promise in minimizing energy requirements during the liquefaction process. Membranes can effectively separate hydrogen from other gases with high efficiency, which optimizes overall performance.
Another innovative area involves magnetic refrigeration technologies. These systems utilize magnetic fields to cool hydrogen without traditional refrigerants, potentially streamlining the liquefaction process and improving energy efficiency.
The development of cryogenic systems also continues to advance. Enhanced materials that withstand low temperatures, coupled with data-driven technologies, can lead to more effective and economical liquefaction operations.
Role in Renewable Energy Transition
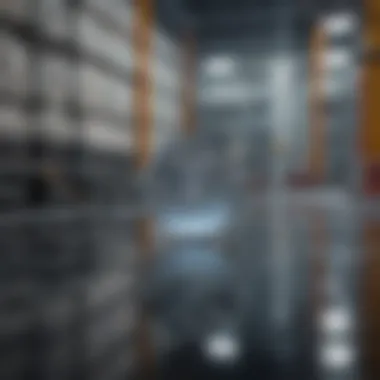
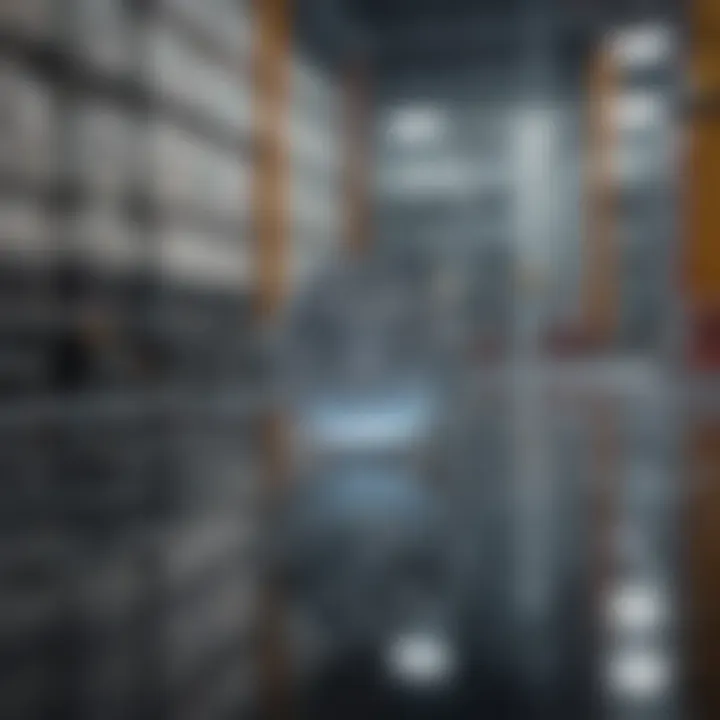
The role of hydrogen liquefaction in the renewable energy transition is fundamental. As countries aim for carbon neutrality, the integration of renewable energy sources becomes vital. Hydrogen produced from excess renewable energy can be stored in a liquefied state, allowing for flexible usage across sectors.
Liquefied hydrogen can transport renewable energy over long distances, providing a solution to the intermittency of sources like solar and wind. This flexibility not just alleviates storage issues but also supports grid stabilization, which is crucial to any renewable strategy.
By facilitating this transition, liquefied hydrogen can also significantly reduce reliance on fossil fuels. As industries strive to decarbonize, hydrogen serves as a clean alternative in applications ranging from manufacturing to shipping. Recognizing these promising roles of hydrogen contributes to an optimistic outlook for sustainable energy developments.
Comparison with Other Gases
Liquefaction of Natural Gas
Natural gas, primarily composed of methane, undergoes liquefaction at much higher temperatures compared to hydrogen. The liquefaction temperature for natural gas is around -162°C, which is significantly warmer than the -253°C required for hydrogen. This distinction has important implications.
Natural gas liquefaction typically uses technologies like the Cascade process or the Mixed Refrigerant process, which involve cooling the gas until it condenses. In contrast, hydrogen requires methods that utilize cryogenic technologies, which are more complex and expensive, reflecting the need for specific equipment and operational conditions.
The liquefied natural gas (LNG) industry is well established, with extensive global shipping and storage infrastructure. In this regard, hydrogen liquefaction is still developing, and more research is required to create efficient and economically viable processes.
A key benefit of liquefied natural gas is its storage density, which facilitates transportation over long distances. The widespread experience with natural gas helps provide critical insights into how to tackle challenges faced in hydrogen liquefaction.
Storage and Transportation Differences
When comparing storage and transportation, hydrogen exhibits distinct challenges compared to natural gas. Hydrogen's low boiling point necessitates specialized cryogenic storage tanks, which are much more costly to construct and maintain than LNG tanks. Furthermore, hydrogen has a lower energy density when compared to LNG, which means more volume is required to store equivalent energy amounts.
The transportation of liquefied hydrogen also poses safety concerns. Hydrogen is highly flammable and can be prone to leakage. This necessitates rigorous regulatory measures and safety protocols to mitigate risks when transported. In contrast, while natural gas is also flammable, it is handled with more established safety practices due to its longer history in the energy sector.
Some noteworthy differences between hydrogen and natural gas transportation include:
- Pipeline Infrastructure: Currently, hydrogen distribution systems are not as widespread as those for natural gas, leading to higher logistic challenges.
- Cryogenic Transport: Hydrogen requires specialized cryogenic containers for transportation, unlike LNG, which has more accommodating transport technologies.
- Energy Efficiency: The efficiency of energy transport can vary significantly; therefore, optimization is essential for hydrogen to be a viable alternative fuel source.
In summary, the comparison between hydrogen and other gases, particularly natural gas, highlights critical differences in liquefaction processes, storage challenges, and safety measures. Recognizing these distinctions is vital for advancing hydrogen liquefaction technology and facilitating its integration into existing energy systems.
Regulatory Framework
The regulatory framework surrounding hydrogen liquefaction is crucial for ensuring safety, environmental protection, and consistency across the industry. The complex nature of handling liquefied hydrogen, which involves specific physical and chemical properties, necessitates detailed guidelines. These standards govern not only the technological processes but also the broader implications for public health and ecological balance. Compliance with regulations helps reduce risks associated with storage, distribution, and use of liquefied hydrogen in various applications.
This framework encompasses various elements, including international standards and national regulations, shaping the operational landscape for entities involved in hydrogen liquefaction. The benefits of following these regulations are manifold. They assist in promoting best practices, minimizing environmental impacts, and fostering public trust in hydrogen technologies.
Furthermore, a robust regulatory framework plays a pivotal role in encouraging investment in hydrogen infrastructure, facilitating the transition towards sustainable energy solutions. By clearly defining responsibilities and expectations, the framework contributes to the long-term viability of hydrogen as a key player in global energy systems.
International Standards
International standards serve as a benchmark for best practices in hydrogen liquefaction. These guidelines, often set by organizations such as the International Organization for Standardization (ISO), aim to create a uniform approach to safety and efficiency. They provide a foundation that companies can adopt to ensure their processes align with global expectations. In particular, ISO 16110-1 outlines the methods for testing hydrogen fuel systems, which is essential for assessing the safety and performance of liquefied hydrogen systems.
Adherence to these standards can lead to several benefits:
- Improved safety measures in handling and transportation of hydrogen.
- Standardization across processes, leading to enhanced operational efficiency.
- Enhanced data sharing and transparency, crucial for technological advancements.
"Adopting international standards not only enhances safety but also fosters innovation through collaboration and knowledge sharing among industry players."
National Regulations
National regulations vary by country but typically reflect the underlying principles set by international standards. They adapt global guidelines to local conditions, taking into account the specific needs and risks associated with hydrogen liquefaction in distinct regions. For example, in the United States, organizations such as the Department of Transportation (DOT) and the Occupational Safety and Health Administration (OSHA) establish regulations that govern hydrogen transportation and workplace safety.
Key considerations for national regulations include:
- Compliance with local environmental laws to prevent pollution.
- Ensuring public safety through rigorous testing and certification processes.
- Promoting technological advancement while ensuring protective measures are in place.
National regulations, thus, enhance the effectiveness of international standards by making them applicable and relevant to the specific societal and environmental contexts within each jurisdiction. They serve as a critical framework for ensuring that the liquefaction of hydrogen unfolds safely and sustainably on a global scale.
Ending
The exploration of hydrogen liquefaction is essential for understanding its wider application in various fields such as energy, transportation, and space exploration. The conclusion serves to consolidate the insights and reflections drawn throughout the article. By summarizing previous discussions, it offers clarity on the substantial impacts of hydrogen liquefaction on sustainable energy solutions. Furthermore, this section can highlight the scientific achievements that have made hydrogen liquefaction more efficient and accessible.
In examining the diverse applications of liquefied hydrogen, we uncover a multifaceted landscape where innovation meets necessity. From powering fuel cells in eco-friendly vehicles to serving as a critical propellant in rocket launches, the relevance and importance of liquefied hydrogen extend beyond mere technicality; it possesses the potential to revolutionize energy paradigms.
Summary of Key Points
- Importance of Liquefied Hydrogen: Emphasizes its role in reducing carbon emissions and contributing to green technologies.
- Methods of Liquefaction: Reviews various processes such as the Linde Process and Claude Cycle, noting their significance in achieving low temperatures.
- Safety and Environmental Concerns: Discusses hazards associated with liquefaction and mitigation strategies crucial for a safe operational environment.
- Regulatory Landscape: Highlights the importance of adhering to international standards and national regulations to facilitate growth in the hydrogen sector.
Future Outlook
The future of hydrogen liquefaction appears promising, with ongoing research driving efficiency improvements. As technologies evolve, the integration of hydrogen into existing energy systems will likely increase. The collaboration between governments and private enterprises is key in shaping a conducive framework for innovations. Legislative support and public acceptance are also critical components for the advancement of hydrogen applications.
"The transition to sustainable energy relies significantly on how effectively we can harness and utilize liquefied hydrogen in various sectors."
Ultimately, with advancements in liquefaction technology and a progressive regulatory environment, hydrogen can play a pivotal role in our efforts to combat climate change and transition to cleaner energy solutions. By understanding its processes and applications, stakeholders can better prepare for a future where hydrogen may dominate the energy landscape.