Insulin Action Mechanism: Key to Glucose Metabolism
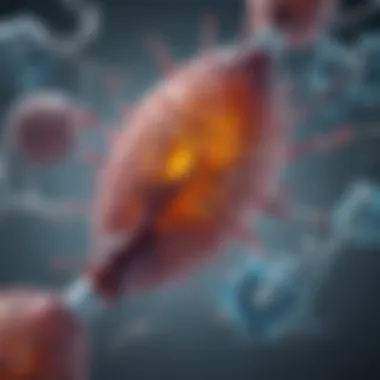
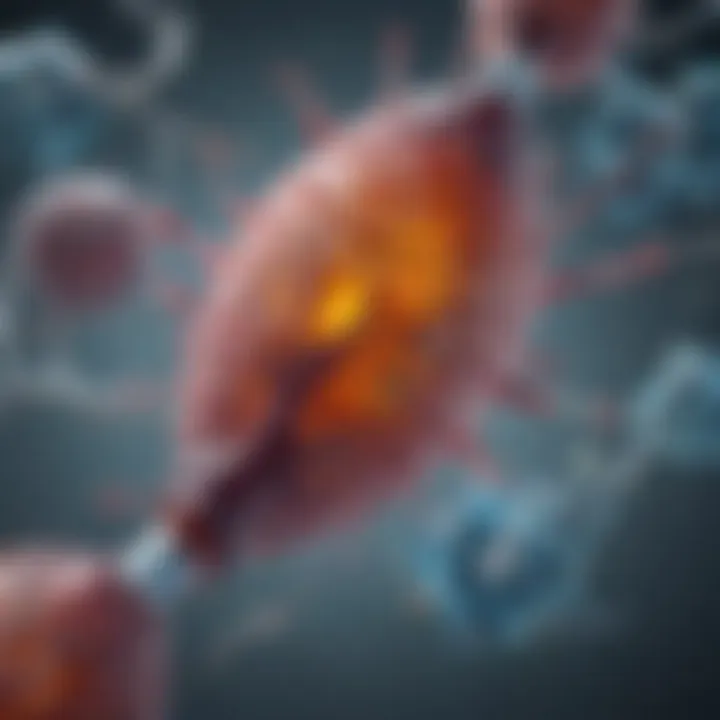
Intro
The role of insulin in the body is more than just a regulator of blood sugar levels; it is a crucial player in various biochemical processes that sustain life. To appreciate insulin's function, one must look deeper into its mechanisms. This exploration will shed light on how insulin works and why it matters not only in the context of metabolic health but also in conditions such as diabetes.
Research Overview
Summary of Key Findings
The investigation of insulin action reveals a complex interplay between biochemical pathways, receptor interactions, and cellular responses. Several notable findings stem from this research:
- Insulin Receptors: Insulin operates through its receptors on cellular membranes, initiating a cascade of reactions that facilitate glucose uptake and utilization.
- Biochemical Pathways: Critical pathways like the PI3K/Akt signaling pathway are activated, influencing various cellular functions, including lipid synthesis and protein metabolism.
- Insulin Resistance: A growing body of evidence highlights the rise of insulin resistance as a widespread issue, particularly in relation to obesity and sedentary lifestyles.
Relevance to Current Scientific Discussions
Insulin's significance extends beyond individual health; it intersects with broader discussions in public health, nutrition science, and diabetes research. Current debates revolve around effective strategies to combat insulin resistance and the exploration of novel therapeutic approaches. Notably, the implications of metabolic health are now critical as it relates to global health trends.
Methodology
Research Design and Approach
The exploration of insulin mechanisms was anchored in a combination of experimental and observational studies, including:
- Clinical Trials: Investigating insulin administration and its effects on participants with varying degrees of insulin sensitivity.
- In Vitro Studies: Laboratory studies on cell lines that provide insight into receptor behavior and signaling pathways.
- Data from Epidemiological Studies: Correlating lifestyle factors with insulin sensitivity metrics across diverse populations.
Data Collection and Analysis Techniques
Data was gathered through:
- Surveys and Questionnaires targeted at understanding dietary habits and physical activity levels.
- Blood Tests assessing insulin levels and other metabolic markers.
- Analysis of Genetic Data to understand hereditary factors associated with insulin action.
Through these comprehensive methods, researchers aim to demystify the mechanisms of insulin, revealing practical relevance for future medical interventions.
Prelims to Insulin Action
Insulin action is a fundamental pillar in the complex architecture of human metabolism. Understanding it opens doors to grasp pivotal biochemical processes that govern how our bodies utilize energy, maintain glucose levels, and manage overall metabolic health. The intricate interplay between insulin and various cellular mechanisms can make a significant difference not only in academic settings but also in real-world applications, such as diabetes management and nutritional science.
Historical Context
The narrative surrounding insulin traces back to the 1920s when the discovery of this vital hormone transformed medical science. Before then, diabetes was a near-certain death sentence as no effective treatments existed. The isolation of insulin by Frederick Banting and Charles Best marked a watershed moment in the field of endocrinology and opened up an entire new area of research. This discovery led to the synthesis of insulin, introducing a practical solution for those suffering from diabetes mellitus.
Over the decades, research has richly developed to explore not only the compound's therapeutic roles but also its broader implications in metabolic pathways, thriving research efforts to understand resistance mechanisms and potential innovative treatments for disorders linked to insulin dysfunction.
Importance of Insulin in Metabolism
Insulin is often positioned as the gatekeeper of glucose metabolism. It promotes the uptake of glucose in various tissues, particularly muscle and adipose tissue, a process necessary for energy production and storage. When you munch on that slice of cake, your blood glucose rises, and insulin comes into play like a well-timed conductor. It signals cells to extract sugar from the blood, thus preventing the dreaded spike that can lead to long-term health issues.
Furthermore, insulin plays an essential role in the lipid metabolism. It influences the synthesis of fatty acids and their storage in adipose tissue. In this sense, the hormone doesn’t merely help with sugar balance; it orchestrates a cohesive response linking carbohydrate and fat metabolism, essential for maintaining energy homeostasis within the body.
In summary, grasping the nuances of insulin action reveals an intricate tapestry of biochemical events. It provides critical insight into how our bodies perform daily metabolic functions while also highlighting areas where dysfunction can lead to significant health challenges. The discussion that unfolds in this article is not just about a single hormone; it's about an expansive metabolic dialogue crucial for our well-being.
Biochemistry of Insulin
The biochemistry of insulin is fundamental to understanding its role in regulating glucose levels and maintaining metabolic balance. This section not only deciphers the molecular structure and functional aspects of insulin but also provides insights into its synthesis and timely release, which are crucial for effective metabolic regulation. Grasping these biochemical intricacies is essential for professionals and researchers alike, as they lay the groundwork for exploring conditions such as diabetes and metabolic disorders.
Insulin Structure and Composition
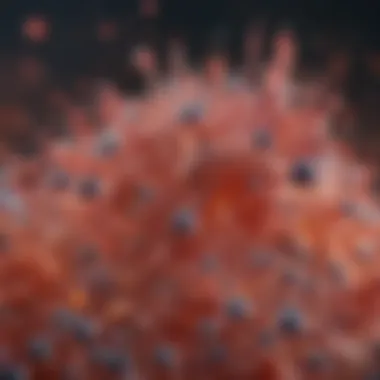
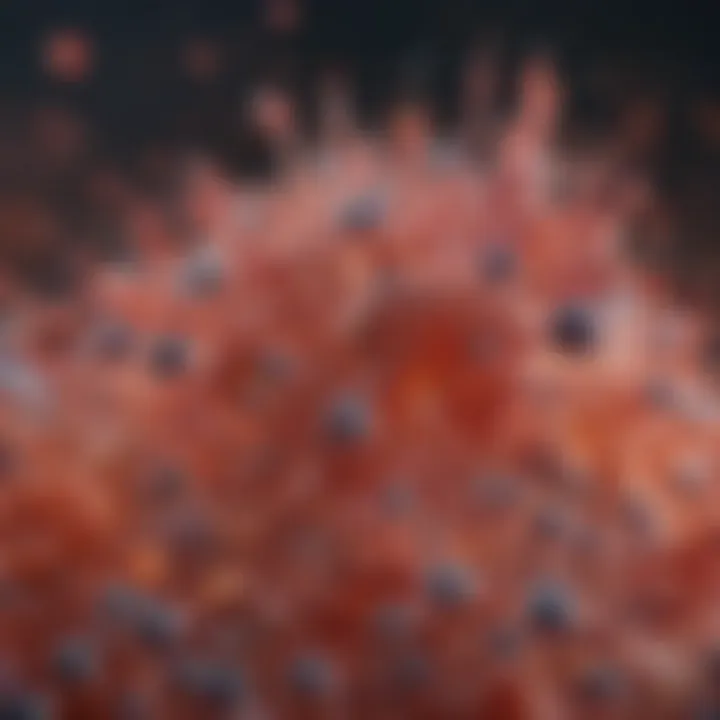
Insulin is a peptide hormone with a well-defined structure that consists of two chains: the A chain and the B chain, linked together by disulfide bonds. The A chain has 21 amino acids, while the B chain contains 30 amino acids. This precise arrangement enables insulin to fit into its receptor, triggering the biological response that facilitates glucose uptake into cells.
The specifics of insulin's molecular structure play an integral role in its function. As a protein, insulin is synthesized as a prohormone known as preproinsulin, which is then processed into proinsulin. Within the beta cells of the pancreas, proinsulin undergoes cleavage to yield insulin and C-peptide. Understanding this structure highlights why even slight alterations in its composition can lead to significant metabolic dysfunctions.
"The precise structure of insulin is not merely a molecular curiosity but a determinant of its effectiveness in glucose metabolism."
Synthesis and Secretion of Insulin
The synthesis of insulin occurs predominantly in the pancreatic beta cells. This intricate process begins with the transcription of the insulin gene into messenger RNA (mRNA), which then directs the assembly of the preproinsulin peptide. Upon being synthesized, this precursor molecule is transported into the endoplasmic reticulum, where it is folded correctly and cleaved to form proinsulin. Finally, proinsulin is sent to the Golgi apparatus, where it gets packaged into secretory granules.
Insulin secretion is a tightly regulated process that mainly occurs in response to elevated blood glucose levels. When glucose is available, it enters the beta cells, resulting in increased ATP production. The rise in ATP levels leads to the closure of ATP-sensitive potassium channels, causing depolarization of the cell membrane. This triggers the opening of calcium channels, allowing calcium ions to flow into the cell. The surge in intracellular calcium ultimately induces the fusion of insulin granules with the membrane, releasing insulin into the bloodstream. The elegance of this biochemical cascade underscores the intricate relationship between glucose levels and the secretion of insulin.
In summary, the biochemistry of insulin extends beyond mere structure and synthesis; it includes an understanding of the physiological conditions that govern insulin release and its downstream effects on cellular metabolism, making it a cornerstone of metabolic health.
Mechanism of Insulin Action
In the realm of metabolic processes, understanding the mechanism of insulin action is nothing short of essential. This article highlights how insulin not only serves as a key regulator of glucose levels but also orchestrates various physiological responses that maintain energy balance. Grasping the intricacies of insulin action reveals the interconnectedness of hormonal influences and sheds light on conditions like diabetes, where such mechanisms falter. This section will break down the components of insulin signaling, providing insight into its functions and the implications of effective glucose homeostasis.
Insulin Receptor and Signal Transduction
Insulin receptors stand at the gateway of insulin signaling, ensuring that the hormone exerts its influence on cells engaged in glucose uptake and metabolism. The unique aspects of insulin receptors underpin their significance.
Type of Insulin Receptors
There are two primary types of insulin receptors: commercially referred to as Insulin Receptor Type A and Type B. Type A receptors are sparsely found in tissues like the liver, while Type B receptors dominate in muscle and fat tissues. Each type’s distribution reflects its functional priorities in metabolism. The characteristic of having diverse receptor types allows for a tailored response to insulin, adapting to different tissue environments. This specificity is chief in the adaptation of insulin sensitivity among various cells. Notably, the selective expression of these receptors influences how the body responds to insulin and, thus, its effect on overall metabolic health.
Activation of Receptor Tyrosine Kinase
An important feature of insulin receptors is their function as receptor tyrosine kinases. Upon binding with insulin, they undergo autophosphorylation, triggering a cascade of events vital for downstream signaling. This activation phase is crucial, as it leads to the phosphorylation of various substrates inside the cell, which amplify the insulin signal. The capability of receptor tyrosine kinases to initiate multiple pathways emphasizes their importance in fine-tuning cellular responses. However, issues such as receptor desensitization can arise, complicating insulin action.
Intracellular Signaling Pathways
Delving deeper into cellular mechanisms uncovers intricate signaling pathways initiated by insulin receptor activation. These pathways play significant roles in mediating insulin’s effects on metabolism.
PI3K/Akt Pathway
The PI3K/Akt pathway emerges as a primary signaling route activated by insulin. Following receptor activation, PI3K, or phosphatidylinositol 3-kinase, gets recruited and activated, propelling the phosphorylation of Akt, a vital player in promoting glucose uptake. This pathway’s hallmark is its ability to foster an anabolic environment within cells, urging them to store nutrients rather than break them down. The relevance of the PI3K/Akt pathway lies in its ability to regulate metabolic actions that are crucial in maintaining energy balance.
MAPK Pathway
Another pivotal signaling route is the MAPK pathway, which interfaces with insulin action, albeit through more complex routes. Unlike the PI3K/Akt pathway, MAPK primarily deals with gene expression and cellular growth responses. This pathway’s activation is central to mediating long-term adaptations in cells, such as proliferation. Its dual functionality — short-term actions related to glucose uptake and longer-term effects on gene regulation — marks MAPK as indispensable to the full spectrum of insulin actions.
Effects on Glucose Homeostasis
Glucose homeostasis hinges on the efficient transport and storage of glucose, processes regulated meticulously by insulin. Understanding these mechanisms provides pivotal insight into metabolic control.
Uptake of Glucose
At the heart of insulin's role in glucose homeostasis lies the uptake of glucose by cells. Insulin stimulates the translocation of glucose transporters to the cell membrane, allowing for increased glucose influx. The ability to enhance glucose uptake is a central characteristic of insulin's function, making it a vital player in maintaining blood sugar levels. When insulin is effective, it encourages a shift toward cellular fuel utilization, which is critical in preventing hyperglycemia.
Storage as Glycogen
Beyond immediate glucose uptake, insulin is also key in storage as glycogen. In the liver and muscle cells, insulin promotes glycogenesis, the process of converting glucose into glycogen for energy reserve. This function is particularly crucial in face of fluctuating energy demands. The unique feature of glycogen storage is that it secures energy for future use, allowing the body to manage periods of low glucose availability effectively. However, if the storage mechanisms prevail consistently, or if there are disturbances in this process, it may pave the way for conditions such as insulin resistance or diabetes.
Understanding these mechanisms highlights the intricate balance insulin must maintain to support metabolic health. The signaling pathways activated by insulin facilitate not just glucose management; they influence a broad array of biochemical processes that sustain life.
Interaction with Other Hormones
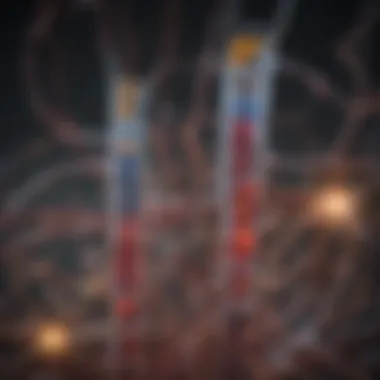
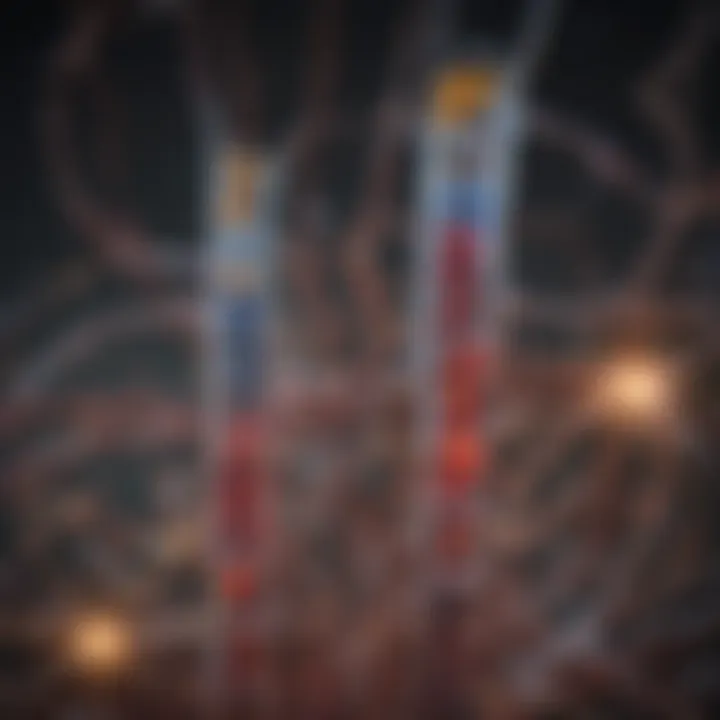
Understanding the interaction between insulin and other hormones is pivotal in grasping the broader landscape of metabolic regulation. Insulin does not work in isolation; rather, it is part of a complex web of hormonal signaling that maintains metabolic balance. Counter-regulatory hormones like glucagon and epinephrine play crucial roles in this dynamic. These hormones, while promoting glucose production and release, counteract insulin’s effects. This balance is vital for events such as fasting, exercise, and stress responses. By delving into these interactions, we gain insights into conditions like diabetes and metabolic syndrome, where hormonal imbalances can spell trouble for health.
Counter-Regulatory Hormones
Counter-regulatory hormones are systemic messengers that elevate blood glucose levels, essentially acting as the yin to insulin’s yang. These hormones 'kick in' during stress or low glucose conditions to ensure that the body continues functioning optimally. Below, we’ll discuss two key players: glucagon and epinephrine.
Glucagon
Glucagon's primary role lies in raising blood glucose levels, acting on the liver to release stored glucose into the bloodstream. Produced by the alpha cells of the pancreas, glucagon is like a safety net, ensuring that energy sources remain available when insulin levels drop. This is particularly crucial during fasting periods or when blood sugar is falling.
A key characteristic of glucagon is its action on the liver, where it stimulates gluconeogenesis—the process of producing glucose from non-carbohydrate sources. For healthcare professionals and researchers, glucagon's regulatory function presents an intriguing opportunity for therapeutic approaches in managing hypoglycemia or improving metabolic control.
However, a unique feature of glucagon is its potential downsides. In hyperglycemic states, excessive glucagon release can exacerbate insulin resistance, creating a vicious cycle that complicates management of diabetes. The duality of glucagon in metabolic regulation, therefore, underlines its importance in this article, as understanding its role helps delineate the complexity of blood sugar control.
Epinephrine
Epinephrine, commonly known as adrenaline, fuels the body’s fight-or-flight response, enhancing blood sugar levels via several mechanisms. Synthesized primarily in the adrenal glands, this hormone increases glucose availability during stressful situations, ensuring that energy is accessible for immediate use.
One of epinephrine's influential characteristics is its ability to mobilize energy stores by enhancing glycogenolysis—the breakdown of glycogen to release glucose. This makes it an invaluable contributor to metabolic pathways during intense physical activities or stress-related scenarios. For researchers and educators, epinephrine serves as a pivotal focus in studies about metabolic health and response to stress, helping clarify how hormones coordinate to optimize energy supply.
Nevertheless, epinephrine’s unique feature also extends to its potential for adverse effects if levels remain high chronically, particularly in individuals with diabetes. Overproduction of epinephrine can lead to increased insulin resistance, further complicating the metabolic landscape. Thus, its inclusion in this examination provides nuanced insight into both physiological responses and pathological conditions.
Integration in Metabolic Pathways
The integration of insulin with other hormones like glucagon and epinephrine highlights a sophisticated regulatory network. The interplay between these hormones orchestrates metabolic responses that are crucial for maintaining homeostasis. Effective glucose homeostasis is not simply a function of insulin alone but occurs through a finely tuned balance of multiple hormones:
- Feedback mechanisms— which ensure homeostasis by modulating hormone levels based on real-time physiological needs.
- Cross-talk between signaling pathways— that amplifies or dampens responses based on overall metabolic status.
- Adjustments in hormone secretion that reflect shifts in energy demands or availability.
An illustrative case of this integration can be seen during fasting. Insulin levels drop, allowing glucagon and epinephrine to take the lead in increasing blood glucose levels. This multilayered interaction serves as a reminder of how these hormones contribute collectively to metabolic health and the complexities surrounding disorders like diabetes.
Understanding these dynamics can shine a light on innovative approaches for therapeutic interventions and lifestyle modifications aimed at restoring metabolic harmony.
Pathophysiology of Insulin Resistance
Understanding insulin resistance is crucial for comprehending the intricacies of diabetes and other metabolic disorders. This pathophysiological state occurs when cells fail to respond effectively to insulin, a critical hormone for glucose metabolism. The implications of insulin resistance dive deep into various health complications, including type 2 diabetes, obesity, and cardiovascular diseases. By exploring the mechanisms behind insulin resistance, we can gain essential insights that could inform therapeutic strategies and lifestyle interventions.
Mechanisms of Insulin Resistance
Receptor Dysfunction
Receptor dysfunction plays a significant role in the overall picture of insulin resistance. This condition occurs when the insulin receptors on cell membranes become less sensitive to insulin. Various factors contribute to this issue, including prolonged exposure to high levels of insulin, leading to a phenomenon known as receptor downregulation.
One of the key characteristics of receptor dysfunction is the alteration of cell signaling pathways. When insulin binds to its receptor, it should trigger a cascade of events that facilitate glucose uptake. However, receptor dysfunction disrupts this process, often resulting in reduced glucose absorption by tissues.
This topic is particularly beneficial for this article because it highlights a critical layer of insulin resistance. The unique feature of this dysfunction is that it can be influenced by several factors, including genetic predispositions, inflammation, and toxins. These factors can disadvantage individuals, rendering them more susceptible to metabolic disorders.
Understanding receptor dysfunction offers advantages such as identifying potential targets for therapeutic interventions, paving the way for more personalized medicine approaches in treating insulin resistance and its associated conditions.
Post-Receptor Defects
On the heels of receptor dysfunction are post-receptor defects, which encompass various abnormalities that occur after the initial insulin-receptor interaction. This aspect is important because even when receptors function correctly, any defect in downstream signaling mechanisms can contribute to insulin resistance.
The key characteristic of post-receptor defects is that they can arise from multiple causes, including genetic variations, lipid accumulation, and oxidative stress. These defects impede vital metabolic processes, leading to impaired glucose uptake and utilization. This malfunction can ultimately culminate in the development of type 2 diabetes and other serious health issues.
The uniqueness of post-receptor defects lies in their ability to persist even when insulin levels normalize, highlighting a persistent state of metabolic dysfunction. Recognizing these defects can lead to significant advancements in research and treatment, leading to novel strategies to target specific signaling pathways involved in insulin action.
Impact on Glucose Metabolism
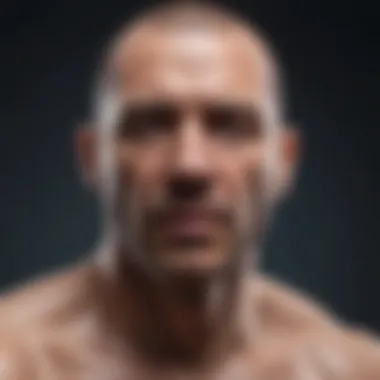
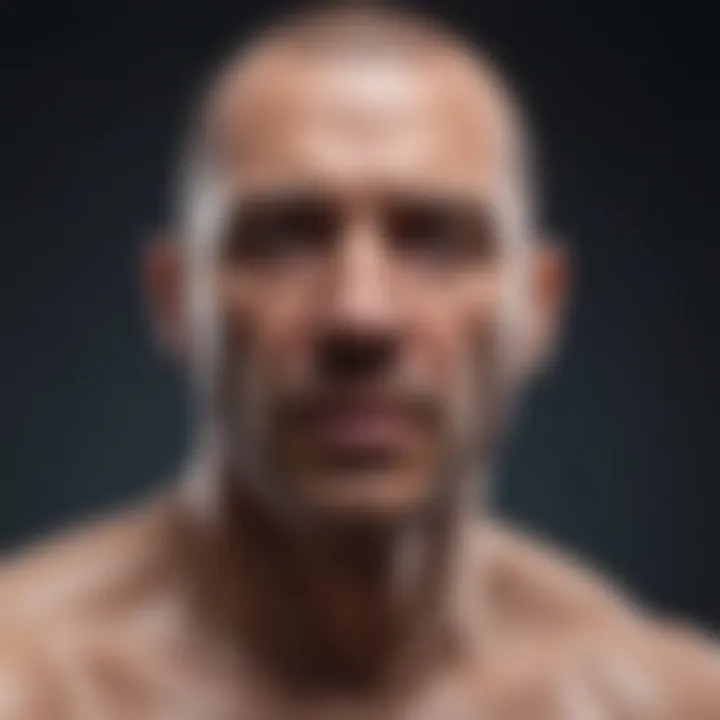
The effect of insulin resistance on glucose metabolism cannot be overstated. When tissues such as muscle and fat fail to respond to insulin appropriately, it leads to increased blood glucose levels, which can have a cascading effect on the body. The inability to utilize glucose effectively means that the liver continues to produce it, exacerbating hyperglycemia.
Moreover, as insulin resistance sets in, the body may compensate by increasing insulin production, which might work temporarily but can ultimately lead to beta-cell dysfunction in the pancreas. Chronic high levels of glucose and insulin can increase risks for multiple health complications over time, ranging from metabolic syndrome to cardiovascular diseases.
Understanding these impacts allows healthcare professionals to devise better tools and strategies for educators, researchers, and practitioners aimed at combating the effects of insulin resistance.
"A comprehensive grasp of the mechanisms behind insulin resistance paves the way for innovative research directions, integrating knowledge into practical health solutions."
In summary, the exploration of pathophysiology related to insulin resistance represents a critical facet of understanding diabetes and related metabolic conditions. By dissecting the roles of receptor dysfunction and post-receptor defects, we uncover vital pathways that can be targeted for therapeutic interventions.
Clinical Implications of Insulin Mechanisms
Understanding the clinical implications of insulin mechanisms is crucial for a comprehensive grasp of diabetes and metabolic disorders. This knowledge is not just academic; it has tangible benefits for patients, healthcare providers, and researchers alike. Recognizing how insulin functions and what disrupts its mechanism can significantly impact the management of diabetes mellitus, particularly in tailoring treatment strategies for individual patients.
Diabetes Mellitus
Type vs. Type Diabetes
When talking about diabetes, two main types often pop up: Type 1 and Type 2. Type 1 diabetes is an autoimmune condition where the body attacks insulin-producing beta cells in the pancreas. This creates a clear dependency on exogenous insulin for survival. In contrast, Type 2 diabetes is more insidious, usually arising from a combination of insulin resistance and inadequate insulin production.
The key characteristic that distinguishes the two is the origin of insulin deficiency. Type 1 is a total lack, while Type 2 often involves a gradual decline in function along with resistance. This distinction is significant as it influences treatment approaches. For instance, individuals with Type 1 require immediate insulin administration, while those with Type 2 might manage with lifestyle changes initially or oral medications.
The unique feature of Type 2 is its early development of insulin resistance, which can sometimes be reversed through lifestyle changes. However, failure to manage this can lead to complications such as cardiovascular disease, neuropathy, and kidney issues. This discussion highlights the importance of understanding the cellular mechanisms behind insulin action—an essential component in developing effective therapeutic interventions.
Therapeutic Approaches
When discussing therapeutic approaches for diabetes, the focus is on insulin therapy and newer classes of medications like GLP-1 receptor agonists and SGLT2 inhibitors. Each of these treatments has unique characteristics that make them valuable. Insulin therapy is a cornerstone of diabetes management but can lead to complications like hypoglycemia and weight gain, which present challenges.
Newer medications, on the other hand, target varied pathways related to glucose homeostasis. For instance, GLP-1 receptor agonists enhance insulin secretion in a glucose-dependent manner and help with weight management, making them quite popular for Type 2 diabetes patients. These therapies provide significant advances in managing diabetes but also pose questions about long-term efficacy and safety.
The upside to these therapeutic approaches is their ability to personalize treatment, aligning with individual patient needs and responses. Yet, they also require continuous monitoring and education, emphasizing the need for healthcare providers to stay informed about the evolving treatment landscape.
Potential Research Directions
With the ever-evolving landscape of diabetes management, potential research directions are vast. Exploring novel insulin sensitizers or mechanisms to restore beta-cell function can open new avenues for treatment. There’s also ongoing research into understanding the genetic predisposition to insulin resistance which could lead to preventative measures.
In looking ahead, there’s a keen interest in the intersection of technology and diabetes management. Continuous glucose monitoring (CGM) systems and artificial pancreas technology are promising areas. Not only do they enhance real-time monitoring, but they could offer a more integrated way of managing blood sugars effectively.
"Grasping the nuances of insulin mechanisms not only benefits clinical outcomes but profoundly alters the patient experience through informed therapeutic decisions."
In summary, the clinical implications of insulin mechanisms are far-reaching. From understanding the differences between diabetes types to exploring new therapeutic avenues, knowledge continues to be the bridge between effective management and potential advancements in the field.
Finale
The conclusion serves as the final word in our exploration of the mechanism of insulin action, weaving together the intricate threads of knowledge presented throughout this article. By stepping back to examine our journey, we highlight the paramount importance of understanding insulin’s roles in metabolism, cellular function, and its ever-present relationship with insulin resistance.
Summarization of Key Insights
In summarizing the insights gained from each section, we uncover several critical themes:
- Biochemical Complexity: Insulin's structure and composition are not merely academic curiosities; they underpin its complex mechanisms of action. By grasping the nuances of these biochemical pathways, we enhance our understanding of both normal and pathological states.
- Signal Transduction Precision: The operation of insulin receptors and the subsequent signal transduction pathways, fundamentally changing how cells respond to the hormone, are potent examples of biological elegance. The activities of pathways like PI3K/Akt and MAPK demonstrate insulin’s multi-faceted effects, from glucose uptake to gene expression.
- Balance of Hormonal Interaction: The discussion around counter-regulatory hormones, such as glucagon and epinephrine, illustrates how insulin does not act in isolation but within a larger hormonal ecosystem, striving to maintain metabolic homeostasis.
- Impact of Insulin Resistance: Recognizing mechanisms behind insulin resistance opens doors to preventive strategies and therapeutic approaches in combating metabolic diseases like diabetes, which is an increasing concern in modern society.
In consolidating these insights, we look not just at the biochemical reactions but at their implications for metabolic health and disease states.
Future Perspectives on Insulin Research
Looking forward, the landscape of insulin research brims with opportunities for discovery and innovation. Here are several avenues worth noting:
- Personalized Medicine: Advances in genetics and genomics can pave the way for tailored diabetes management strategies. Understanding individual responses to insulin treatment can help in designing therapies that cater more precisely to patient needs.
- Novel Therapeutics: With the rise in insulin resistance, new drugs targeting different aspects of insulin signaling pathways are being explored. Research into compounds that can enhance insulin sensitivity without the unwanted side effects of current therapies is an exciting frontier.
- Long-Term Effects of Insulin Use: The implications of long-term insulin therapy need robust investigation. As diabetes management continues to evolve, understanding the long-term consequences of chronic insulin delivery will be paramount in enhancing patient outcomes.
- Integration of Technology: Wearable technology and continuous glucose monitoring systems are changing how insulin therapy is administered. This confluence of technology and biology holds promise for better managing fluctuations in blood glucose levels, allowing for a more dynamic response to insulin treatment.
In concluding our dive into insulin action mechanisms, it is clear that the implications of this research stretch far beyond the laboratory. As we continue to unravel the biological and clinical impacts, particularly concerning insulin resistance and diabetes, the importance of ongoing research cannot be overstated. Whether you're a student eager to learn, a researcher on the cutting edge, or a clinician at the forefront of patient care, this knowledge can make waves in how we understand and approach metabolic health.
"The future depends on what we do in the present." - Mahatma Gandhi.
This curated understanding of insulin action will serve as a cornerstone for future investigations and therapeutic endeavors.