The Significance of pH in Science and Ecosystems
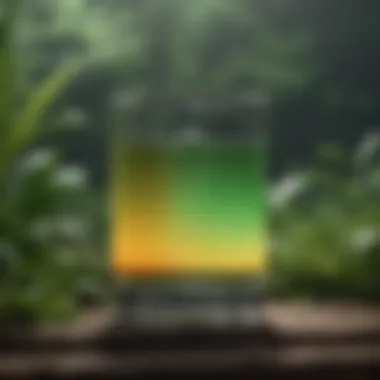
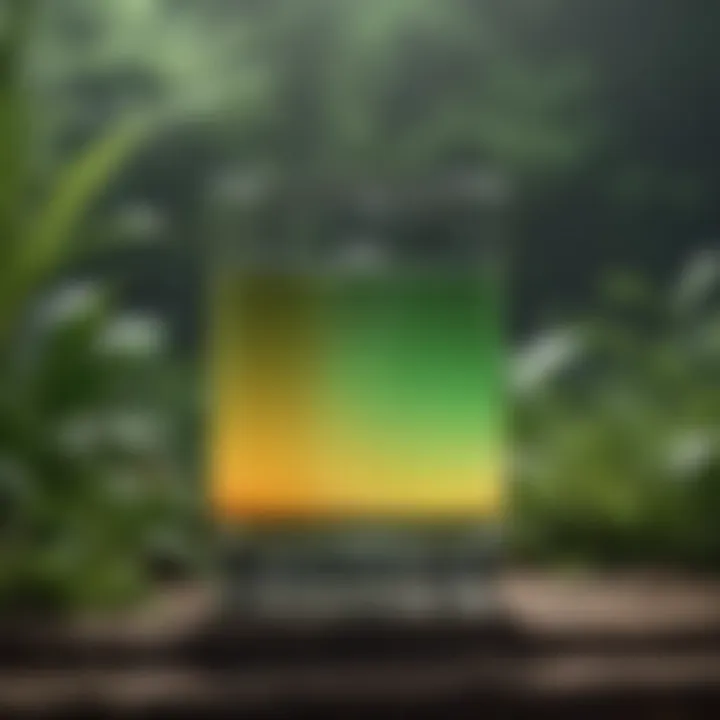
Intro
Understanding pH is akin to holding a compass in a complex landscape; it guides scientists through the intricacies of biological and chemical systems. The term pH refers to the hydrogen ion concentration in a solution and serves as a key indicator of acidity or alkalinity. This measure is often overlooked, yet its significance stretches far across various scientific fields, influencing everything from biochemical reactions in living organisms to the behavior of minerals in soil.
The concept of pH can be both fascinating and a bit tricky. A standard scale ranges from 0 to 14, with 7 being neutral, values below indicating acidity, and numbers above suggesting alkalinity. However, the implications of these numbers are profound. For instance, a slight adjustment in pH can dramatically change the dynamics of a biochemical reaction. The importance of this balance is starkly depicted in natural systems, where disruptions can have cascading effects on ecosystems, agriculture, and even human health.
As we delve deeper into the nature of pH, we’ll explore various dimensions of this essential concept. From examining its role in aquatic chemistry to its consequences in biological processes, we're set to uncover how critical pH management and measurement is to maintaining the integrity of life itself. It’s not just about numbers; it’s about understanding the very fabric of interaction that sustains our world.
Preamble to pH Concepts
The concept of pH is one of the cornerstones of understanding both biological and chemical systems. Whether you are exploring cellular processes in biology, developing products in chemistry, or managing ecosystems in environmental science, pH plays a critical role across these varied domains. For students and professionals alike, grasping the nuances of pH can enhance their abilities to analyze, predict, and manipulate parameters within any system effectively.
This section covers essential definitions and the historical context of pH measurement, paving the way for a more profound exploration of its implications in later sections.
Definition and Importance of pH
At its core, pH is a logarithmic scale that quantifies the acidity or basicity of a solution. The scale generally ranges from 0 to 14, with 7 being neutral. Solutions with a pH less than 7 are considered acidic, while those with a pH above 7 are basic (or alkaline). Many might think pH is just a scientific curiosity, but its implications are far-reaching.
In biological systems, for instance, enzymes—the workhorses of metabolic reactions—often operate within specific pH ranges. If these levels fluctuate too much, enzyme activity can slow or even cease altogether, leading to dire consequences for cells.
Consider the example of E. coli, a common bacterium in the human gut. This organism thrives at a neutral pH. Altering the environment could upset its balance, impacting our digestive health. On the chemical side, pH influences reaction rates and equilibria. For example, in a solution, the ionization of weak acids and bases is dependent on pH, which can dictate yield in industrial processes.
"The understanding of pH is not merely an academic exercise; it holds the key to manipulating biological functions and chemical reactions."
Ultimately, a solid grasp of pH allows professionals in their field to make informed decisions, affecting not just experiments, but also applications in health and agriculture.
Historical Context of pH Measurement
The journey of pH measurement dates back to the early 20th century when chemist Søren P. Sorensen introduced the term in 1909. Sorensen devised this scale to simplify the language around acid and base concentrations, enriching discussions in laboratory settings.
Before the pH scale, understanding acid-base interactions relied heavily on visual indicators, such as litmus paper. These initial methods provided a basic means of understanding acidity but were limited in accuracy and sensitivity. The advent of potentiometric pH meters in the mid-20th century revolutionized the field, providing precise and consistent measurements that could cater to a vast array of applications, from pharmaceuticals to environmental monitoring.
To appreciate the significance of pH measurement today, consider its crucial role in ensuring public health through wastewater treatment or quality control in food and beverage industries. Subtle shifts in pH can signal larger issues. For instance, in aquatic ecosystems, even a minor alteration in water pH can lead to the die-off of sensitive species. Therefore, the legacy of pH measurement extends beyond mere numbers; it influences regulations, conservation efforts, and health standards worldwide.
The Chemistry of pH
The concept of pH is at the heart of understanding many chemical and biological processes. It acts like a compass, guiding the reactions that occur in everything from your body's cells to the ecosystems around us. Thus, grasping the chemistry of pH is fundamental to both academic study and practical applications in various fields of science.
Understanding pH requires knowledge about acids and bases, how they interact, and the tools we use to measure those interactions. The implications of pH extend beyond mere numbers; pH levels can dictate the outcome of chemical reactions and biological functions alike. For instance, the right pH can enhance enzyme activity or ensure aquatic life thrives in the waters they call home.
Understanding Acids and Bases
Acids and bases are the two key players when discussing pH.
Bronsted-Lowry Theory
The Bronsted-Lowry theory provides a crucial framework for understanding how acids and bases operate. It defines acids as proton donors and bases as proton acceptors, which illustrates their interactive relationship in reactions. One of the key characteristics of this theory is its versatility; it applies across many chemical scenarios and aids in predicting the direction of a reaction.
A beneficial aspect of the Bronsted-Lowry model is its simplicity, making it accessible for students to visualize reactions. Yet, its limitation lies in the need for knowledge of protons in various environments, which can be tricky at times.
Arrhenius Definition
The Arrhenius concept is another foundational aspect of acid-base chemistry. This definition characterizes acids as substances that release hydrogen ions in solution, while bases release hydroxide ions. Its straightforward nature allows for quick assessments of acid and base behavior, contributing to our understanding of pH in both academic and practical contexts.
One key characteristic is its focus on water as the medium for reactions, which is vital since many biological and environmental processes occur in aqueous solutions. However, the Arrhenius definition can sometimes be overly restrictive, failing to include non-aqueous environments that are equally important in various scientific fields.
Lewis Acid-Base Concept
The Lewis acid-base concept takes the analysis a step further by defining acids as electron pair acceptors and bases as electron pair donors. This definition illuminates various non-protonic chemical reactions, making it an essential tool for chemists.
A notable characteristic of the Lewis model is its broader applicability; it can encompass reactions outside of aqueous solutions. This makes it particularly valuable in organic chemistry and materials science. On the downside, the abstraction can sometimes be complex, requiring a more advanced understanding of chemical interactions.
Measuring pH Levels
Accurate measurement of pH is pivotal for both scientific research and practical applications. Specialists rely on several methodologies to assess pH levels, each with its merits and downsides.
pH Meters and Electrode Types
Utilizing pH meters is one of the most common methods for measuring pH. These devices function by analyzing the voltage difference between two electrodes inserted into a solution. A standout aspect of pH meters is their precision; they can provide quick and accurate readings critical for lab experiments or industrial processes. Different types of electrodes exist, each tailored to specific conditions—glass electrodes are notable for general applications, while specialized ones cater to extreme pH levels.
However, the main caveat with pH meters is that they require regular calibration and maintenance to ensure their accuracy, demanding a certain level of expertise and diligence in their use.
Colorimetric Methods
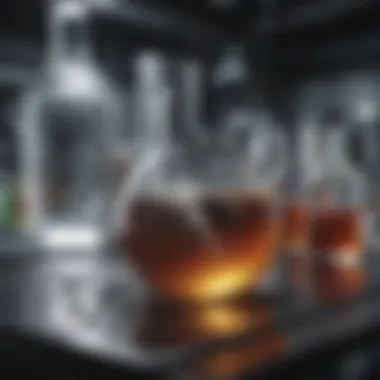
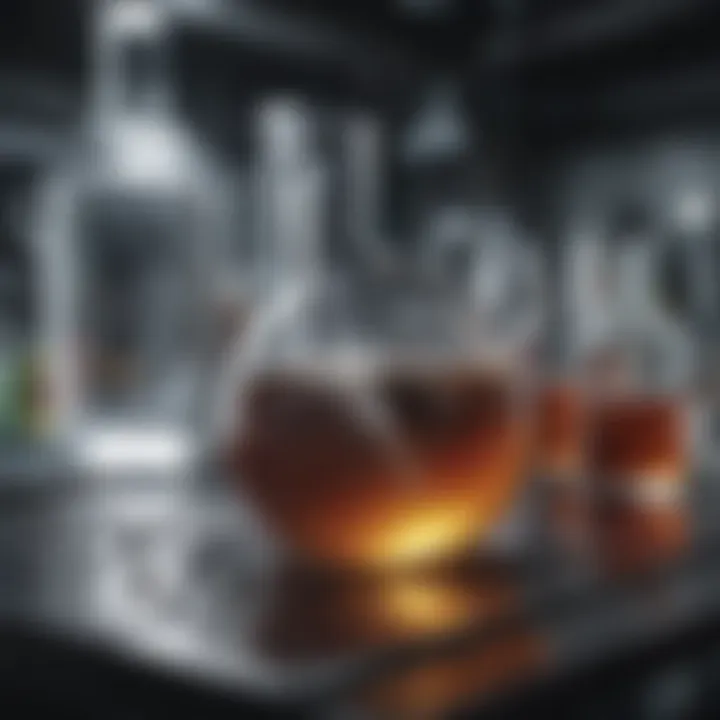
Colorimetric methods provide an alternative avenue for pH assessment. By using pH indicators that change color based on acidity or alkalinity, researchers can visually interpret pH levels. This method is particularly beneficial in educational settings, demonstrating basic concepts of chemistry in a hands-on manner.
The key characteristic of colorimetric approaches is their simplicity—no specialized equipment is needed, making them accessible for various applications, from beginner labs to field studies. That said, they can lack the precision of meters, especially in the presence of complex mixtures or when subtle pH differences are at play.
Challenges in Accurate Measurement
Despite advancements in measurement techniques, challenges persist in achieving accurate pH readings. Environmental factors, such as temperature and ionic strength, can significantly skew results. This characteristic highlights the need for careful sample preparation and consideration of external influences on pH readings.
Additionally, pH levels can fluctuate rapidly in some systems, like in dynamic biological environments, complicating the reliability of measurements. Thus, understanding these variables is crucial for anyone seeking to make informed conclusions based on pH data.
"Accurate pH measurement is not just about understanding the numbers; it’s about grasping the underlying dynamics that affect chemical and biological systems."
Biological Significance of pH
The significance of pH in biological contexts cannot be overstated. Throughout various life processes, the delicate balance of pH works like a finely tuned instrument, allowing for optimal function of enzymes, cellular metabolic pathways, and the overall homeostasis of living organisms. Understanding how pH levels fluctuate and affect biological systems reveals not only the adaptive strategies of organisms but also the underlying principles that sustain life.
pH in Biological Systems
Enzyme Activity and pH
Enzymes, the catalysts of biological reactions, have a specific pH range in which they exhibit maximal activity. This range is often referred to as the enzyme's optimal pH. For instance, pepsin, an enzyme in the stomach, is most active at a pH around 1.5 to 2, which corresponds to the highly acidic environment necessary for digestion. On the flip side, enzymes found in the small intestine, such as trypsin, function best in a more alkaline pH around 7.5 to 8.
Key characteristic: The sensitivity of enzyme activity to pH is crucial for understanding metabolic processes. Rapid changes in pH can lead to denaturation of enzymes, rendering them ineffective.
Unique feature: This sensitivity can be a double-edged sword; on one hand, it allows for precise biochemical control. On the other, it means that even slight changes in pH can severely impact an organism's ability to process nutrients, signaling the importance of maintaining a stable internal environment, or homeostasis.
Cellular Metabolism
Cellular metabolism encompasses a myriad of chemical reactions that sustain life, including catabolic and anabolic processes. These reactions are also highly dependent on pH levels. For example, during glycolysis, the conversion of glucose to pyruvate is influenced by the acidic or alkaline nature of the cytoplasm.
Key characteristic: The microenvironments within cells may have differing pH levels, affecting how various metabolic pathways operate. Metabolic acidosis, for instance, can occur when the body produces excessive acids or the kidneys do not remove enough acid.
Unique feature: The dependence of metabolic pathways on pH demonstrates why organisms adapt their environments or bodily fluids to maintain homeostasis, thus ensuring efficient energy production.
Homeostasis Mechanisms
Homeostasis mechanisms are the processes through which living organisms regulate their internal environment. For example, organisms have buffer systems, which are solutions that resist changes in pH upon the addition of acids or bases. One of the most well-known buffer systems in human blood is the bicarbonate buffer system, which plays a crucial role in maintaining a stable pH around 7.4.
Key characteristic: Homeostatic control mechanisms often involve feedback loops that either enhance or shut down certain processes based on pH readings. This characteristic is vital because a shift beyond optimal pH ranges can lead to severe biological consequences, including metabolic disorders.
Unique feature: While the regulation of pH is generally effective in complex organisms, certain factors such as diet, illness, and environmental stress can dramatically affect these control systems, illustrating the fragility of homeostatic mechanisms.
Effects of pH on Organisms
Adaptations to pH Variability
Organisms have evolved impressive strategies to cope with varying pH levels in their environments. For instance, certain fish species can thrive in waters with widely different pH, such as the unique adaptations of the Atlantic salmon that migrate between freshwater and saltwater habitats.
Key characteristic: Such adaptations include physiological changes to regulate internal pH, which allow organisms to flourish in diverse conditions.
Unique feature: This versatility is beneficial as it supports survival and reproduction amidst changing environments, though it comes at the cost of energy expenditure and potential stress responses.
Impacts on Growth and Development
The impacts of pH on growth and developmental stages are profound. In plants, for example, an optimal soil pH is essential for nutrient uptake. Many nutrients become unavailable at extreme pH levels; for instance, soils that are too acidic can lock away vital minerals.
Key characteristic: The link between pH and nutrient availability emphasizes the importance of pH in agricultural practices and ecosystem sustainability.
Unique feature: The complex interplay between pH and nutrient absorption can dramatically affect not just individual plant health but also broader ecosystem dynamics, illustrating the interconnected nature of environmental factors.
Case Studies in Microenvironmental pH
Research into specific microenvironments demonstrates the critical nature of localized pH variations. For example, studies of soil pH have shown that different plant communities exhibit distinct adaptations to local pH conditions.
Key characteristic: These microenvironments can greatly affect biodiversity, as species that are intolerant to certain pH thresholds may struggle to survive.
Unique feature: Understanding these local adaptations provides valuable insights for conservation efforts and habitat management, showcasing the importance of pH in ecological research.
Understanding the role of pH in biological systems not only enlightens us on how life sustains itself but also guides research efforts toward improving health and environmental sustainability.
pH in Environmental Science
The concept of pH resonates deeply in environmental science, unearthing vital insights into how living organisms interact with their surroundings. The measurement and regulation of pH are fundamental, seeing as they govern the availability of nutrients in soil, affect aquatic life, and influence broader ecological relationships. Through understanding pH, we can unlock keys to enhancing agricultural practices and preserving aquatic ecosystems. Exploring pH in this context reveals its indispensable role in fostering sustainable development and ecological balance.
Soil pH and Agriculture
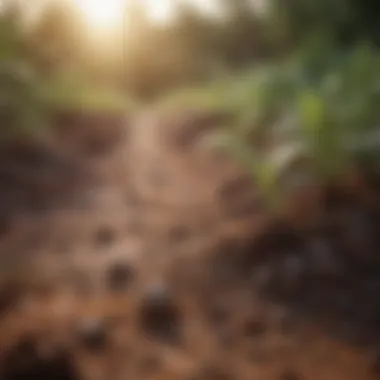
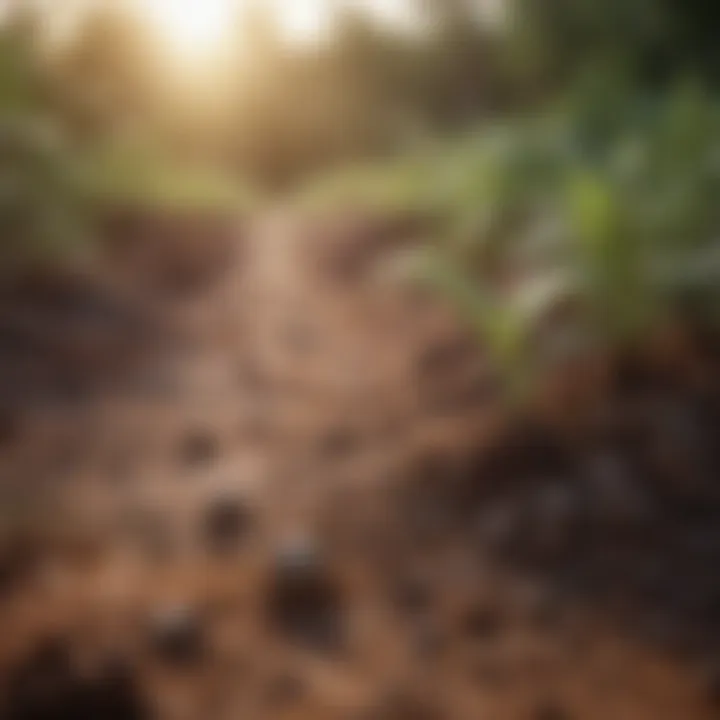
Soil Nutrients and pH
Soil pH directly impacts the availability of nutrients essential for plant growth. It determines whether the soil is acidic, neutral, or alkaline, which in turn influences how well plants can absorb minerals like nitrogen, phosphorus, and potassium. For instance, in acidic soils, nutrients such as calcium might be less available, potentially hindering crop yield.
The key characteristic of soil pH lies in its ability to influence nutrient solubility. This is why understanding and managing pH is a coveted topic in agricultural circles. Soil with a pH range of about 6 to 7 is often seen as optimal, as it creates a favorable environment for nutrient interaction.
One unique feature of soil pH is its dynamic nature; it can change with factors like organic matter decomposition and water drainage. While maintaining ideal pH can seem like a tall order, the right strategies can enhance nutrient uptake and improve soil health.
pH Management in Farming
Managing pH in farming is a critical practice that can lead to productivity gains. This involves not just measuring pH levels but also implementing strategies to adjust and maintain them within a suitable range. Soil amendments such as lime or sulfur can be added to correct acidity or alkalinity, promoting optimal growing conditions.
This aspect of pH management is noteworthy because it can significantly affect soil structure and microbial activity, which are crucial for long-term soil fertility. Unique to this practice is the idea of ongoing management; it’s not a one-time task but rather an ongoing process tailored to specific crop needs and local conditions. The drawback, of course, is that it requires regular monitoring and an understanding of the intricate relationships between soil chemistry and biological systems.
Implications for Crop Yield
The pH of soil can significantly dictate crop yield outcomes. When the pH is well-maintained, plants exhibit improved nutrient uptake, stronger root systems, and increased resilience to pests and diseases. In contrast, poor pH management can lead to nutrient deficiencies or toxicities, severely limiting crop productivity.
The distinguishing feature of this aspect is its importance in the broader agricultural framework. As climate variability makes some regions more acidic, the need for precise pH management becomes increasingly relevant. While benefits to yield are clear, the challenge rests in balancing economic factors and environmental sustainability in pH management practices.
Aquatic Ecosystems and pH
Impact on Marine Life
In aquatic ecosystems, pH significantly affects the health and diversity of marine life. Various species have specific pH requirements; for instance, most fish thrive in neutral to slightly alkaline water. Shifts in pH can lead to stress in marine organisms, affecting their growth and reproduction.
A key characteristic of the interplay between pH and marine life is the concept of tolerance. Some species are flexible, reaping benefits in nutrient-rich waters, while others are highly sensitive to even minor pH changes. This adaptability in species interactions is essential for maintaining biodiversity and ecosystem stability.
One downside to these relationships is that human activities, such as pollution and runoff, can lead to altered pH levels, endangering sensitive species and disrupting local ecosystems.
Coral Reefs and Ocean Acidification
Coral reefs serve as crucial indicators of ocean health, and they are particularly vulnerable to changes in pH due to increasing carbon emissions and ocean acidification. When ocean water absorbs excess CO2, it lowers the pH and disrupts calcium carbonate formation, which is essential for coral growth.
This dual challenge of pH and coral viability is significant; coral reefs not only provide habitat for a vast array of marine life but also protect coastlines. The unique features of this topic lie in the symbiotic relationships between coral and algae, which depend on stable pH levels for mutual survival and flourishing.
While the research into ocean acidification is gaining traction, the implications for biodiversity losses linked to declining coral health can't be overstated. These changes pose threats to food security, tourism, and coastal livelihoods.
Water Quality Assessment
Water quality assessment often incorporates pH as a fundamental parameter. Accurate pH readings can help identify pollutants and assess ecosystem health. Regular monitoring of pH levels assists in detecting harmful shifts that might indicate a declining ecosystem.
An important characteristic of utilizing pH in water quality assessment is its role as an early warning system. When pH drops, it can signal potential risks not only to marine life but also to human health, particularly in areas where communities rely on freshwater sources.
However, one of the challenges that come with pH monitoring is the resolution of data collection methods and ensuring consistent accuracy across various conditions. Ensuring proficient management of pH in these ecosystems calls for interdisciplinary approaches, where science meets policy, fostering better environmental stewardship.
Industrial Applications of pH
The realm of industrial applications of pH plays a pivotal role across various fields, particularly those steeped in chemical processes and healthcare. Maintaining the optimal pH level is not merely the concern of scientists in labs; it extends into manufacturing practices, where slight deviations can lead to significant implications. pH affects everything—from the safety of products to their effectiveness. As industries increasingly recognize this importance, the need for effective pH management becomes ever more evident.
pH in Chemical Manufacturing
In chemical manufacturing, pH levels are critical to the efficiency and safety of processes. Without precise measurement, manufacturers risk product inconsistency, which can lead to financial losses.
Process Optimization
Process optimization represents a key characteristic when it comes to managing pH levels in chemical manufacturing. By carefully controlling pH, manufacturers can enhance reaction rates and yield, ultimately leading to cost efficiency. It's a method that helps in maintaining balance amid varying raw material qualities. For instance, in the production of fertilizers, adjusting pH during synthesis helps ensure nutrient availability. However, it is essential to monitor this closely; too much adjustment can lead to unwanted chemical reactions or subpar products, which is not a helpful outcome.
Safety and Compliance
Safety and compliance branches into another significant aspect of utilizing pH in industry. Regulations demand that chemical manufacturers adhere to strict safety protocols, including maintaining specific pH ranges to avoid hazardous reactions. Failing to meet these standards can lead to serious legal consequences and, even worse, jeopardize employee safety. Moreover, compliance with environmental regulations often necessitates pH monitoring in waste discharge. This highlights the dual benefit of maintaining pH levels—not just for production efficiency but also to ensure industry meets legal obligations.
Product Quality Control
Quality control is integral to manufacturing, and pH measurement constitutes a core part of this process. Ensuring that products meet quality standards is non-negotiable, as compromised quality can lead to brand damage and decreased customer trust. By establishing acceptable pH ranges, manufacturers can significantly reduce the risk of defects. For instance, in the pharmaceutical industry, the efficacy of medications hinges on precise pH levels. Nevertheless, establishing such standards must also come with an awareness of the advantages and disadvantages. Rigorous pH checks can necessitate additional costs and time, but the trade-offs often result in higher quality products.
Healthcare and pH Regulation
While chemical manufacturing illustrates the necessity of pH control, healthcare brings its unique challenges and rewards. pH plays a role going far beyond mere product efficacy; it influences treatment effectiveness and diagnostic accuracy.
pH in Pharmaceuticals
In pharmaceuticals, the specific pH of drugs directly affects their stability and solubility. For instance, certain medications require a controlled pH to ensure optimal absorption in the body. Understanding the chemistry behind drug formulation helps scientists create effective therapies. The distinct characteristic of pH in pharmaceuticals is its dual role as both an active ingredient's requirement and a determinant of patient response. This requires careful balancing, as deviations might compromise drug efficacy.
Clinical Diagnostics
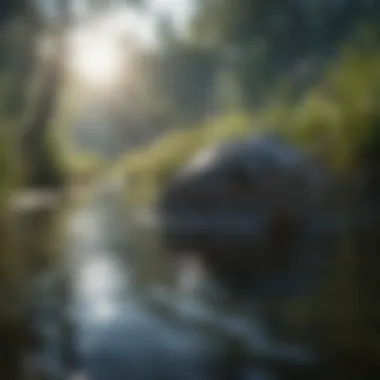
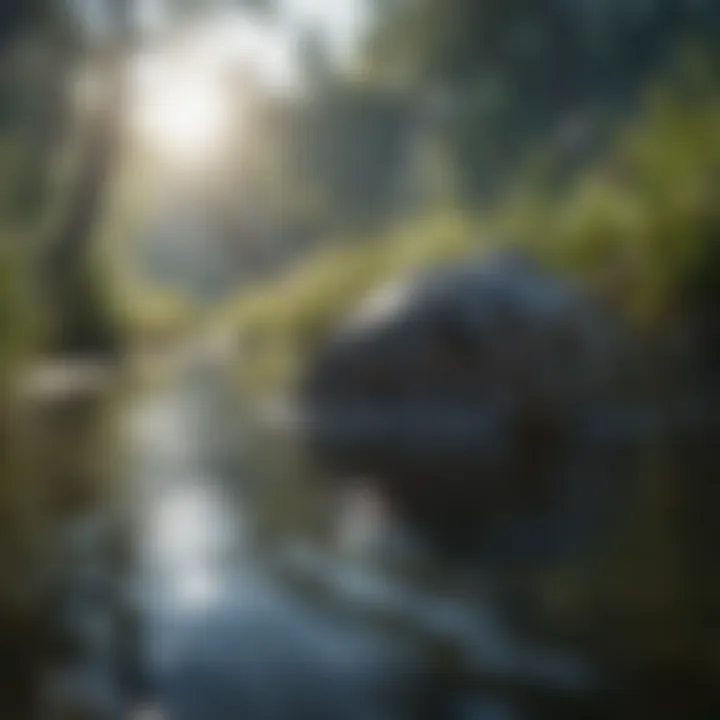
Clinical diagnostics rely heavily on accurately measuring pH levels in bodily fluids. For example, blood tests can reveal a wealth of information, and pH variations can indicate underlying health issues. The key characteristic here is the ability of pH to serve as a marker for health. As such, clinical contexts see pH as more than a measurement; it becomes a diagnostic tool. However, misinterpretation can lead to incorrect treatment plans, emphasizing the relentless need for accuracy in diagnostic processes.
pH in Blood and Body Fluids
Lastly, the pH of blood and other body fluids holds immense significance in maintaining homeostasis. The human body thrives within a very narrow pH range—much like delicate ecosystems. For example, a shift towards acidity can signal metabolic disorders, while increased alkalinity could indicate respiratory issues. Given that blood pH requires precise regulation, many healthcare interventions focus on correcting imbalances. However, the unique feature of this element lies in how sensitive it is to environmental changes and physiological responses, which can be both a challenge and a focal point in patient care.
"pH is not just a number; it is a reflection of biological rules and chemical compliance!"
As we move forward, understanding the industrial applications of pH in both manufacturing and healthcare can help us recognize its broader significance in ensuring product quality, safety, and health diagnostics.
The Future of pH Research
As we continue to advance in both technology and environmental sciences, the future of pH research holds significant promise. Understanding pH is not just a matter of measuring acidity or alkalinity; it is a window into the complex interplay of chemical and biological processes. With the ongoing developments in measurement technologies, emerging trends in studies, and the increasing necessity for interdisciplinary approaches, the focus on pH research highlights an essential aspect of scientific inquiry.
Innovations in pH Measurement Technologies
Nanotechnology Applications
Nanotechnology is set to revolutionize the way we measure pH. By using nanosensors, researchers are able to obtain extremely precise pH readings that were once unattainable. The key characteristic of these nanosensors is their size and sensitivity, which allows them to be used in highly localized environments, such as within living cells or soil samples.
What makes nanotechnology a popular choice in pH measurement is its potential for miniaturization. Measurement devices can be shrunk to sizes that allow for bio-monitoring and on-site measurements without losing accuracy. However, these sensors can be costly and require careful calibration to ensure reliable results.
Remote Sensing Techniques
Remote sensing techniques present another layer of innovation in pH measurement. By utilizing satellite or drone technology, researchers can monitor pH levels over large areas, providing valuable data for environmental studies. The primary advantage of this method is the ability to gather data from inaccessible locations, reducing the costs associated with traditional sampling methods.
The unique attribute of remote sensing is its ability to continually collect data, creating a dynamic picture of pH variations over time. Yet, this approach has its challenges, including the need for sophisticated algorithms to ensure data accuracy. The interpretation of remote data can sometimes lead to misleading conclusions if not properly analyzed.
Data Analysis and Interpretation
In the realm of pH research, data analysis and interpretation play a crucial role. With the explosion of data from innovative measurement methods, effective data management systems become essential. Tools that integrate machine learning can assist researchers in identifying trends and making predictions based on pH data.
One distinguishing feature of data analysis in pH research is its potential to synthesize information from diverse sources, leading to richer insights. However, this comes with risks; erroneous data can propagate through models, leading to incorrect conclusions. Rigorous checks and balances in data validation are critically important.
Long-term Trends in pH Studies
Climate Change Impacts
The impacts of climate change on pH levels cannot be overstated. This phenomenon affects marine environments specifically, as increased CO2 leads to ocean acidification, impacting coral reefs and marine biodiversity. Understanding these changes offers critical insights into the resilience of ecosystems under threat.
Additionally, monitoring pH shifts provides a tangible measure of environmental health, making it a beneficial focus for researchers aiming to understand broader climate trends. On the downside, attributing specific pH changes solely to climate factors can be complex, often requiring long-term studies to draw accurate correlations.
Future Research Directions
Future research in pH studies is likely to push boundaries. There is a growing interest in how pH interacts with other environmental variables, paving the way for integrated approaches to studying ecosystems. This direction is crucial as the interconnectedness of life means changes to pH can have cascading effects on various biological and chemical processes.
A distinctive feature of future research directions involves leveraging interdisciplinary insights—bringing together chemists, biologists, and environmental scientists. Yet, collaboration can sometimes lead to misalignment in research objectives and methodologies, which must be managed carefully for productive outcomes.
Interdisciplinary Approaches
The necessity of interdisciplinary approaches in pH research cannot be ignored. As scientists begin to recognize the intricacies of pH-related processes, combining expertise from various disciplines promises to yield a more comprehensive understanding. Fields such as hydrology, ecology, and biology could benefit greatly from shared knowledge regarding pH's role.
The key characteristic of interdisciplinary research is its ability to bridge gaps between disciplines, leading to innovative solutions and broader perspectives. That said, balancing different methodologies and knowledge bases can pose challenges, requiring effective communication skills among team members.
How researchers tackle the future of pH remains at the forefront of scientific inquiry.
"Innovation in measuring and interpreting pH levels may lead us to unexpected insights, offering deeper understanding across numerous scientific fields."
Ultimately, the future of pH research is a blend of technology and the quest for knowledge. As research evolves, so too does our capability to understand and manage the world around us.
Culmination
In the grand scheme of science, pH occupies a pivotal position. It’s not merely a number; it’s a window into the very processes that sustain life and drive chemical reactions. Throughout this article, we’ve highlighted how pH influences numerous aspects of biological, chemical, and environmental systems.
Recap of Key Insights
In revisiting key insights from various sections:
- The definition and measurement of pH are foundational not just for laboratory settings but also for real-world applications.
- We discovered that in biological systems, pH plays a crucial role in enzyme activity and cellular processes, showcasing how living organisms adapt to maintain optimal pH levels for survival.
- The implications of pH levels on agriculture and aquatic ecosystems further illustrate the interconnectedness of chemical principles and environmental health. Whether it’s soil nutrients or the complexity of marine life, understanding pH can lead to better conservation and agricultural practices.
- In industrial contexts, the regulation of pH is paramount for ensuring product quality and safety. This underscores the relevance of pH in sectors ranging from healthcare to chemical manufacturing, impacting both consumer safety and economic viability.
By distilling these insights, we can appreciate that pH is less about numbers and more about understanding systems.
"Understanding pH is not just about the acidity or basicity of a solution, it's about grasping the delicate balance of nature's processes."
The Role of pH in Ongoing Research
Research into pH extends beyond simply measuring a solution's acidity or alkalinity. Current investigations are delving into innovations that could radically enhance pH measurement. For instance, nanotechnology is leading to more sensitive and portable pH sensors, opening new avenues in environmental monitoring and public health.
Another burgeoning area focuses on the interplay between climate change and pH levels in various ecosystems, particularly concerning ocean acidification and its effects on marine biodiversity. Future research endeavors are likely to adopt more interdisciplinary approaches, combining insights from biology, chemistry, and ecology to foster a holistic understanding of how pH influences different systems.
In summary, ongoing research promises to deepen our understanding of how pH operates within myriad contexts, illuminating pathways for better environmental management, industrial practices, and healthcare solutions. With each study, we inch closer to grasping the nuances of pH, and it’s clear that the exploration is by no means a finished endeavor.