Head Electrodes: Key Principles and Future Insights
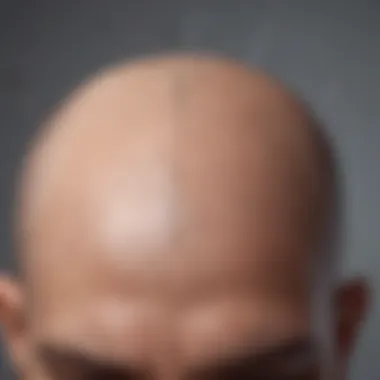
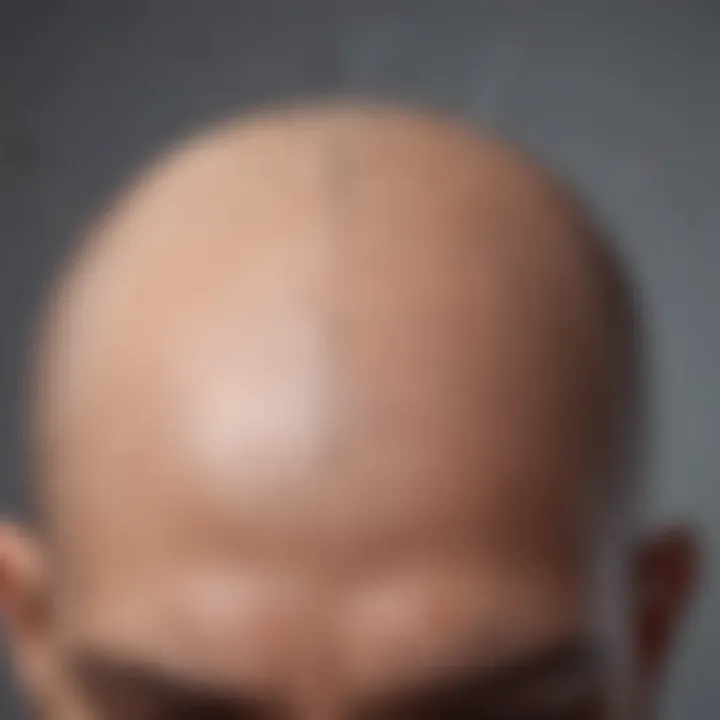
Intro
Head electrodes serve as vital instruments in the field of neuroscience, providing insights into the complex workings of the human brain. These devices facilitate the measurement of electrical activity, allowing researchers and clinicians to observe brain function in real-time. The design and application of head electrodes have evolved significantly over the years, driven by advances in technology and a deeper understanding of neuro physiology. This article will delve into the principles governing head electrodes, their diverse applications, and the future directions that research may take in this important area of study.
Research Overview
Summary of Key Findings
Head electrodes are critical for various applications, including electroencephalography (EEG), which is widely used in both clinical and research settings. Recent advancements have focused on improving the comfort and functionality of these devices. Innovations in materials, such as conductive polymers and nano-scale components, have enhanced signal quality while also reducing the bulk of the electrodes. Studies have revealed that these technologies can provide cleaner, more precise data on brain activity, enabling better diagnosis and treatment for neurological conditions.
"The potential of head electrodes in transforming our understanding of brain activity is immense, making them indispensable tools in modern neuroscience."
Relevance to Current Scientific Discussions
Current discussions in the scientific community revolve around the integration of head electrodes with other imaging techniques, such as functional magnetic resonance imaging (fMRI). The combination of data from these divergent modalities can lead to a more comprehensive understanding of brain activity in both healthy and diseased states. Furthermore, the growing interest in brain-computer interfaces (BCIs) underscores the importance of developing more sophisticated electrode systems.
Methodology
Research Design and Approach
The approach to studying head electrodes often involves both experimental and computational techniques. Researchers may conduct controlled experiments to evaluate the performance of different electrode designs under various conditions. This could also include simulations to predict how changes in material or configuration could impact data collection.
Data Collection and Analysis Techniques
Data collection typically involves recording electrical signals from the scalp using head electrodes in conjunction with software designed for EEG analysis. The data is then processed using specialized algorithms to extract meaningful information, such as frequency bands and event-related potentials. Comparative studies are often conducted to assess the efficacy of new electrode designs against traditional configurations, ensuring that innovations lead to significant improvements in data quality and usability.
The ongoing exploration of head electrodes is crucial for advancing neuroscience and clinical practices. As technology continues to evolve, the implications of these advancements will extend far beyond traditional applications, reinforcing the importance of continued research and development in this critical field.
Intro to Head Electrodes
Head electrodes play a critical role in both neuroscience research and clinical applications. Their function encompasses various techniques that allow us to monitor and understand brain activity. Understanding the principles, materials, and technologies behind head electrodes is essential for those engaged in research or clinical practice.
Definition and Purpose
Head electrodes are devices designed to record electrical activity from the brain. They measure brain waves and provide valuable data regarding neurological function. The purpose of these electrodes is multifaceted, serving not only for research but also for clinical diagnostics and treatments. The data gathered can help in assessing conditions like epilepsy and sleep disorders, offering deeper insights into brain functionality. In essence, head electrodes bridge the gap between raw neural signals and interpretable data that can inform both science and medicine.
Historical Context
The history of head electrodes is rich and illustrates the evolution of neuroscience as a field. The initial attempts to record brain activity can be traced back to the late 19th and early 20th centuries with the development of electroencephalography (EEG). Early devices were rudimentary. However, they laid the groundwork for modern techniques. Over the decades, advancements such as improved materials, better understanding of neurophysiology, and innovative designs have significantly enhanced electrode performance.
Currently, head electrodes have transitioned from large, bulky designs to more sophisticated setups. Technologies such as dry electrodes and flexible materials now dominate the landscape, allowing for more comfort and better signal quality.
Current Trends in Research
The research landscape surrounding head electrodes is dynamic. There is a strong emphasis on the advancement of non-invasive techniques. Researchers are now exploring the integration of wireless technology into head electrodes, which allows for greater flexibility in research settings. Moreover, the push towards more biocompatible materials is noteworthy. These materials not only improve comfort but also mitigate the risk of infections associated with invasive procedures.
Additionally, there is a growing interest in machine learning applications in analyzing data collected from head electrodes. This integration promises to enhance our ability to interpret complex neural signals and could lead to breakthroughs in understanding various neurological conditions.
"Head electrodes are not just tools; they are gateways to unlocking the mysteries of the human brain."
In summary, understanding head electrodes encompasses their definition, historical development, and current research trends. As technology progresses, the implications of these advancements will indeed shape the future of both neuroscience and clinical practices.
Physiological Principles of Head Electrodes
Understanding the physiological principles behind head electrodes is crucial for grasping their functionality and efficacy in various applications. This section delves into the core elements that underpin the technology and offers insights into its significance in neuroscience and clinical settings.
Understanding Neurophysiology
Neurophysiology is the study of the function of the nervous system, particularly the brain and spinal cord. This field is critical for head electrode applications, as it provides a framework for understanding how neural signals are generated and transmitted. Head electrodes are designed to detect these electrical signals, which are fundamental to neural communication.
Electrodes measure the difference in voltage created by neurons firing. Different regions of the brain have varying levels of activity, and these fluctuations can reveal much about cognitive processes. The mapping of brain activity offers insights into normal and abnormal functioning, supporting advancements in both research and clinical diagnostics.
By accurately interpreting the electrical activity of the brain, researchers can explore a range of neurophysiological conditions, including epilepsy, Parkinson’s disease, and cognitive impairments. Understanding these signals is not only vital for academic purposes but is also applied in developing intervention strategies in clinical settings.
Electrical Properties of Brain Tissue
The electrical properties of brain tissue significantly affect how head electrodes operate. The brain’s tissue has a distinct resistance and impedance, which influences the detection of electrical signals.
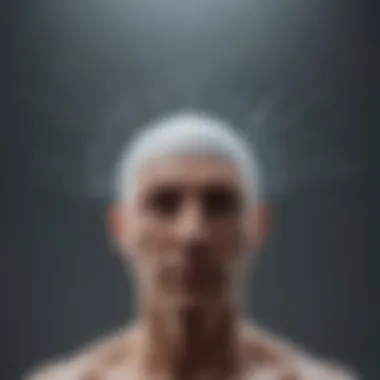
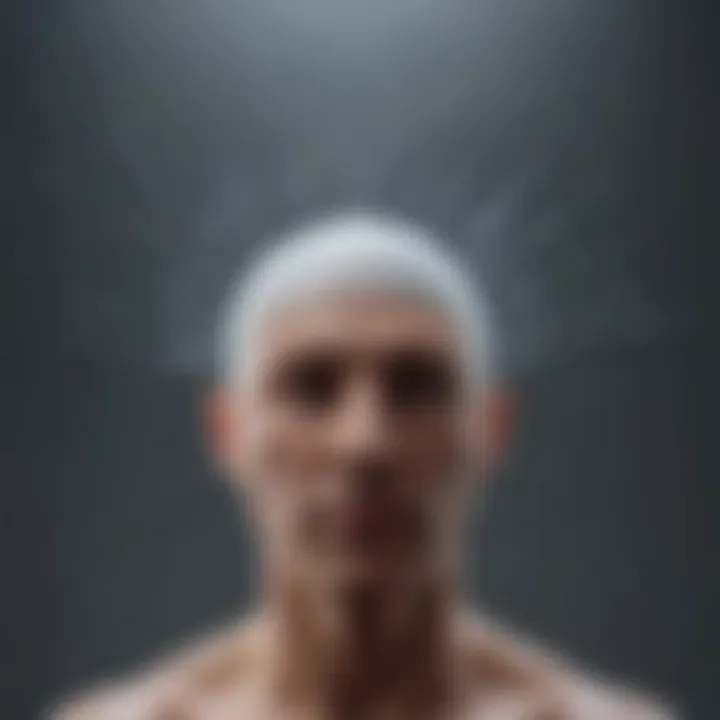
- Conductivity: Brain tissue's conductivity varies across different types of cells and structures. It is essential to understand these variations for optimal electrode design.
- Impedance: High impedance means that less current flows through the tissue, which can affect signal quality. Low impedance is desirable as it allows for better signal transmission.
The choice of electrode material also plays a role in the interaction with brain tissue. Some materials may minimize signal loss and enhance sensitivity. Conductive materials such as gold, silver, and platinum are common choices, each offering unique benefits and limitations.
Signal Transmission Mechanisms
Signal transmission mechanisms are vital for understanding how head electrodes relay data from the brain to external devices. Electrical signals generated by neuron activity propagate through brain tissue and reach the electrode surface. These mechanisms can be broken down as follows:
- Action Potentials: Neurons communicate via action potentials, which are rapid changes in electrical charge. Head electrodes capture these changes as they occur on the surface of the scalp or brain.
- Field Potentials: Unlike action potentials, field potentials reflect the graded changes in membrane potential across many neurons within a localized area. They provide insights into the collective electrical activity of larger groups of neurons.
- Recording Techniques: Different methods such as electroencephalograms (EEGs) and electrocorticograms (ECoGs) utilize various electrode configurations to capture signals effectively. Each technique offers unique advantages in resolution and depth, tailored to specific research and clinical needs.
Understanding these mechanisms highlights the complexity and sophistication of brain activity and the technology designed to study it. Each factor plays a role in enhancing our comprehension of neurological function and dysfunction.
In summary, the physiological principles involving neurophysiology, electrical properties of brain tissue, and signal transmission mechanisms lay the groundwork for head electrode effectiveness. These elements not only impact the performance of the electrodes but also facilitate breakthroughs in neuroscience research and clinical applications.
Types of Head Electrodes
Understanding the various types of head electrodes is critical in the exploration of their applications and advancements in neuroscience. Their classification into invasive, non-invasive, and specialized electrodes allows researchers and clinicians to select the appropriate method for their needs. Each type has unique characteristics, advantages, and considerations which greatly influence the outcomes of studies and clinical results. Choosing the right electrode type can significantly impact the quality of data collected, the comfort of subjects, and the applicability of the results in practice.
Invasive Electrodes
Invasive electrodes are used internally, often requiring surgical procedures to implant them in or near the brain. These electrodes allow for precise measurements of electrical activity and can provide detailed information about neuronal function. The ability to gather data directly from brain tissue yields insights that non-invasive methods cannot achieve. However, the use of invasive electrodes comes with significant risks, including infection, inflammation, and other complications associated with surgery.
Key aspects of invasive electrodes include:
- Placement: They are typically placed in specific brain regions to capture targeted neuronal activity.
- Data Quality: They can offer higher signal fidelity due to reduced noise and interference compared to external methods.
- Applications: They are often used in epilepsy studies and other conditions requiring fine-grained analysis of brain activity.
Despite their advantages, the ethical considerations surrounding their use are substantial. Researchers must ensure informed consent, especially when operating on human subjects, and weigh the benefits against potential harm.
Non-Invasive Electrodes
Non-invasive electrodes are designed to record electrical activity from the scalp, eliminating the need for surgical intervention. Techniques such as electroencephalography (EEG) utilize these electrodes to monitor brain function and connectivity in a safe manner. This category of electrodes offers a more comfortable experience for participants, making them suitable for studies involving larger populations or sensitive groups such as children.
Key features of non-invasive electrodes include:
- Ease of Use: They can be quickly applied and removed, allowing for fast data collection across various settings.
- Functional Diversity: They can be used to monitor brain responses during cognitive tasks, sleep studies, or neurological assessments.
- Cost-Effectiveness: Generally, non-invasive methods are less expensive than their invasive counterparts, facilitating broader research access.
Nevertheless, non-invasive electrodes often face limitations regarding spatial resolution. The signals collected are indirect measurements, which means some specificity and precision may be lost compared to invasive methods.
Specialized Electrodes
Specialized electrodes bridge the gap between invasive and non-invasive techniques, designed for specific applications that require unique functionalities. These electrodes often incorporate advanced materials or technologies to enhance performance and address particular challenges that standard options do not solve.
Examples of specialized electrodes include:
- Flexible Electrodes: These adapt to the brain’s surface, reducing irritation and improving signal acquisition.
- Wireless Electrodes: Incorporating remote transmission capabilities allows for greater patient mobility during data acquisition.
- Multi-modal Electrodes: They enable simultaneous recording across various modalities, such as combining electrical activity data with imaging techniques.
The ongoing development of specialized electrodes is promising, as it may lead to innovations that significantly enhance our understanding of brain activities and disorders. As the field evolves, it is likely that new designs and materials will improve both functionality and safety for users.
"The choice of electrode type is crucial in ensuring that the physiological signals obtained are reliable and applicable to clinical practice."
In summary, the differentiation of head electrodes into invasive, non-invasive, and specialized categories plays a vital role in neuroscience research and clinical applications. Each type brings its unique set of benefits and limitations, necessitating a nuanced understanding to maximize their impact on the advancement of medical technology.
Design Considerations for Head Electrodes
Design considerations play a vital role in the effectiveness and functionality of head electrodes. These electrodes are used for various applications in neuroscience and clinical settings, so their design impacts not only the performance but also the safety of the procedures involved. Having appropriate design elements ensures optimal connectivity and reliability in signal acquisition, which is essential for accurate data analysis and interpretation.
Material Selection
Material selection for head electrodes is critical for performance and usability. The materials must be biocompatible and durable. Common materials include platinum, gold, and conductive polymers. Each material has specific attributes:
- Conductivity: This is crucial for efficient signal transmission.
- Biocompatibility: Minimizing immune response is essential to prevent adverse reactions in invasive electrodes.
- Flexibility: Materials such as conductive polymers allow for movement and comfort in patients.
Choosing the right material also affects the lifespan and maintenance of the electrodes, influencing the long-term outcomes of studies or treatments.
Electrode Configuration
The configuration of head electrodes directly influences how they interact with brain tissue. This includes considerations of:
- Physical layout: Placement of electrodes can vary depending on the specific brain regions being studied. For instance, arranging electrodes in a grid pattern might benefit certain types of brain mapping.
- Number of electrodes: More electrodes can provide greater spatial resolution but may complicate data interpretation.
- Type of electrode: Some configurations may be better suited for invasive procedures, while others are optimized for non-invasive measurements.
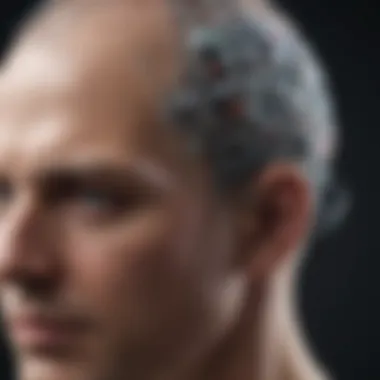
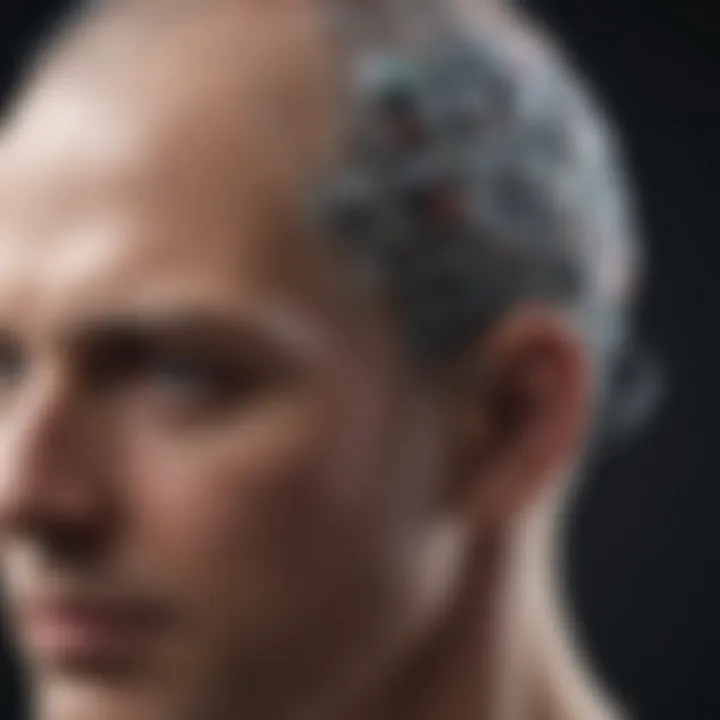
An appropriate configuration can significantly enhance data reliability and the overall efficacy of the monitoring system.
Impedance and Signal Quality
Impedance is a key factor in ensuring high-quality signals are recorded by head electrodes. High impedance can lead to poor signal quality and noise, which can distort the data. Important guidelines include:
- Target impedance levels: Optimal impedance values should be established for specific electrode types to maximize signal acquisition.
- Minimizing contact resistance: Good skin preparation and electrode gel application can reduce impedance and improve signal quality.
- Signal processing techniques: Utilizing advanced algorithms can help in filtering out noise, thus enhancing the clarity of the data being collected.
Proper management of impedance contributes significantly to the fidelity of neural signal recordings, thus enabling accurate research findings.
Applications of Head Electrodes
The applications of head electrodes span a wide range of disciplines, significantly impacting neuroscience, clinical diagnostics, and the development of neuroprosthetics. Each of these areas not only enhances our understanding of brain functionality but also improves patient outcomes and the design of assistive devices for individuals with neurological conditions. The integration of head electrode technology into clinical practices is pivotal; it advances both empirical research and practical applications in healthcare settings. Understanding these applications is critical to grasp the entire spectrum of benefits and challenges associated with head electrodes.
Neuroscience Research
Neuroscience research is one of the prominent fields leveraging head electrodes for deep exploration of brain function. The collection of electrical signals from the scalp or deeper regions allows researchers to study brain activity at unprecedented resolutions. For instance, electroencephalography (EEG) provides real-time feedback on neuronal dynamics, which can be crucial for understanding cognitive processes, emotional responses, and sensory perceptions.
The ability to record brainwaves can lead to findings that inform theories on learning and memory, synaptic efficacy, and neurodegenerative diseases. Researchers utilize head electrodes to gather data that can reveal the brain's response to various stimuli, contributing to cognitive neuroscience's growth as an academic discipline. Moreover, techniques like functional imaging coupled with head electrodes enhance spatial resolution, allowing for a more profound understanding of activity in specific brain regions during various mental tasks.
Clinical Diagnostics
In clinical diagnostics, head electrodes serve as an indispensable tool for diagnosing and monitoring neurological disorders. Conditions such as epilepsy, sleep disorders, and traumatic brain injuries require meticulous monitoring of brain activity to inform treatment decisions. Clinicians often depend on EEG results to determine seizure types and their locations, making it a cornerstone in understanding and managing epilepsy.
Beyond immediate diagnosis, head electrodes allow for long-term monitoring of brain activity, providing valuable insights into disorders like Alzheimer’s and schizophrenia. The versatility of these electrodes enables them to be used in both inpatient and outpatient settings, facilitating ongoing patient assessment and personalized care strategies. Furthermore, innovations in electrode design, such as the use of dry electrodes, simplify the process and increase patient comfort during examinations.
Neuroprosthetics
The realm of neuroprosthetics demonstrates another significant application of head electrodes. These devices offer rehabilitation solutions for individuals with mobility impairments. By interfacing directly with the nervous system, neuroprosthetic devices can transform thought into action, effectively bridging the gap between intention and execution.
Head electrodes are used in brain-computer interfaces (BCIs) which facilitate communication for those with severe disabilities. Research continues to seek better integration of these technologies into daily life, striving for seamless control over assistive devices. Such advancements not only restore functionality but can also improve the quality of life for patients navigating challenges related to motor control.
Furthermore, as the field of neuroprosthetics evolves, ongoing research aims to explore the potential of pairing BCIs with artificial intelligence. Such combinations could result in enhanced responsiveness and personalization of neuroprosthetic devices, allowing for a more intuitive user experience.
The potential for head electrodes in applications ranging from basic research to aiding those with disabilities highlights their crucial role in bridging neuroscience and practical medical solutions.
Technological Advancements in Head Electrodes
The realm of head electrodes is constantly evolving, anchored in the principles of innovation. The technological advancements achieved over recent years have driven significant improvements in both research and clinical implications. These advancements not only enhance the performance of head electrodes but also expand their applications across various domains. The development of such technologies offers multiple benefits, including higher fidelity signal acquisition, increased patient comfort, and a better understanding of brain mechanisms.
Development of Flexible Electrodes
Flexible electrodes represent a breakthrough in comfort and usability. Traditional rigid electrodes can cause discomfort, restricting their application in long-term studies or monitoring. Flexible designs adapt better to the contours of the head, minimizing pressure and improving user tolerance. These electrodes are often made from soft materials, such as polyimide or silicone, that allow for natural movement.
The importance of flexibility extends beyond patient comfort. Flexible electrodes can maintain better contact with brain tissue, yielding more accurate readings. As research into brain function grows, these electrodes become crucial in the study of dynamic activities and changes in brain waves under varying conditions. They also have great potential in the field of neuroprosthetics, where comfortable and effective electrodes can enhance user experience.
Wireless Technology Integration
Wireless technology brings a new dimension to the functionality of head electrodes. Traditional wired systems can limit movement and create inconvenience, particularly in studies requiring mobility, such as physical activities or sleep assessments.
Integrating wireless capabilities can facilitate long-term data collection without the encumbrance of wires. This advancement allows for unobtrusive studies, which can yield more natural behavior in subjects. Additionally, wireless systems often use advanced signal processing algorithms that improve data accuracy. As such technology becomes increasingly accessible, its role in both clinical and research settings is poised to grow.
Real-Time Data Acquisition Systems
Real-time data acquisition systems represent a pivotal shift in how brain activity is monitored and analyzed. These systems enable researchers and clinicians to observe brain functions as they happen, leading to quicker insights and responses during experimental trials or clinical assessments.
The integration of real-time acquisition capabilities enhances the efficiency of data collection. Researchers can make immediate adjustments to conditions based on the data received, optimizing the experimental processes. In clinical settings, this means that immediate intervention can be provided based on patient needs, particularly in situations where neurological events occur, such as seizures or syncope.
In essence, advancements in technology will increasingly dictate the future landscape of head electrodes, supporting endeavors in neuroscience research, clinical diagnostics, and therapeutic applications.
These technological strides not only represent progress but also point towards a future where head electrodes can enhance our understanding of the brain in more nuanced ways. As we move forward, investment in these technologies can drive innovations that further bridge the gap between our understanding of brain activity and practical applications in health care and beyond.
Ethical Considerations
The field of head electrodes involves complex ethical deliberations, particularly given the sensitivity surrounding neuromodulation and neuroscience research. Ethical considerations are crucial to preserving the integrity of the research, protecting the participants, and ensuring that advancements in technology respect individual rights and societal norms. This section delves into the essential aspects of ethical considerations related to informed consent, data privacy, and implications for patient rights.
Informed Consent in Research
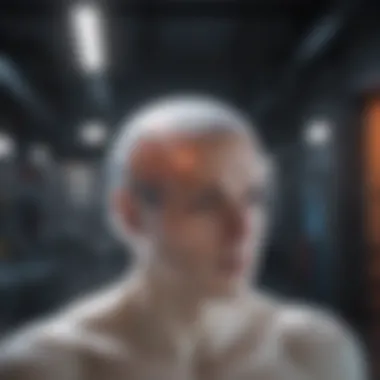
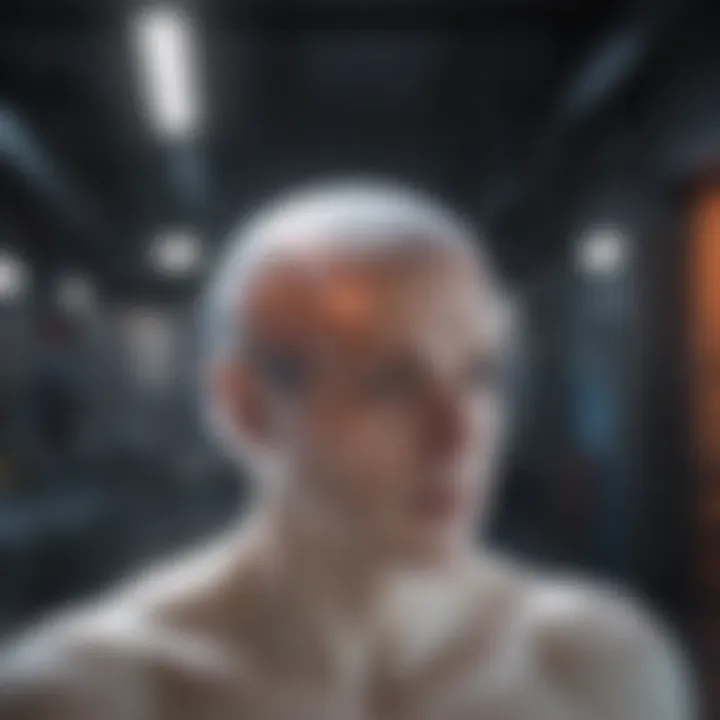
Informed consent is a cornerstone of ethical research practices. For studies involving head electrodes, obtaining informed consent is not merely a formality; it requires a comprehensive understanding from the participants about the nature and potential risks of the procedures. Researchers must ensure that participants are fully aware of what is being studied, how the data will be used, and any possible consequences of their involvement. This means conveying information in a clear and accessible manner, avoiding overly technical jargon that may confuse participants.
Moreover, ongoing consent is vital. Participants should be kept updated about changes in the study that may affect their decision to remain involved. They should also feel entitled to withdraw consent at any point without facing repercussions. This practice enhances trust between researchers and participants, ultimately benefiting the quality of the research.
Data Privacy and Security
The collection of neural data poses significant challenges regarding data privacy and security. With head electrodes recording sensitive information related to brain activity, ensuring stringent data protection measures is paramount. Data should be anonymized whenever possible to protect participant identities and safeguard personal information.
Researchers must comply with relevant regulations and frameworks, such as the General Data Protection Regulation (GDPR) in the European Union, which governs data privacy. This includes implementing robust security protocols, conducting regular audits, and training personnel in data protection practices. Failure to uphold these standards can lead to legal ramifications and a loss of public trust in scientific research.
Implications for Patient Rights
Patient rights must be central to discussions around head electrode technologies. As these devices often cross into medical applications, the implications for patient autonomy and agency are significant. It is essential that patients have a clear understanding of how head electrodes may affect their health and what their rights are in this context.
Furthermore, patients should have access to real-time information regarding their care, particularly when advancements in neurotechnology could influence treatment options. Informed decision-making is essential, empowering patients to participate actively in their healthcare choices. Respecting patient rights not only builds trust but also encourages a collaborative relationship between healthcare providers and patients.
In summary, ethical considerations in the domain of head electrodes are fundamental for both research integrity and the protection of individuals. By prioritizing informed consent, data privacy, and patient rights, the field can advance responsibly.
These elements underscore the importance of embedding ethical principles in the development and application of head electrodes to foster a responsible scientific landscape.
Challenges in Head Electrode Development
The development of head electrodes presents several challenges that are crucial to address. Overcoming these obstacles not only improves the functionality and reliability of the electrodes but also enhances their applications in neuroscience and clinical settings. Understanding these challenges is essential for guiding future innovations in technology that support brain research and therapeutic interventions.
Material Limitations
Material selection plays a critical role in the effectiveness of head electrodes. One of the main challenges is finding materials that provide optimal signal transmission while being biocompatible. The most common materials used, such as metals and conductive polymers, often face limitations. For example, metals can cause inflammatory responses when implanted in the body, while conductive polymers may degrade over time, affecting their performance.
A balanced choice of material should consider both the electrical performance and the long-term stability in biological environments. Current efforts are directed toward the development of nanomaterials and bioinspired materials that promise enhanced integration with biological tissues. The adoption of graphene and carbon nanotubes are making strides in this area due to their excellent conductivity and biocompatibility. However, more research is required to optimize these materials for widespread use.
Interference from External Factors
External factors can significantly affect the performance of head electrodes. Electromagnetic interference, for instance, can distort signals captured by the electrodes, leading to inaccurate data collection. This is particularly problematic in clinical environments, where many devices operate simultaneously.
Furthermore, environmental noise, such as electrical equipment in hospitals, can hinder the clarity of recorded signals. Strategies to mitigate these interference issues include the design of shielding mechanisms or the use of signal processing algorithms. Employing better isolation techniques can also help reduce these interferences, ensuring that data from the electrodes are reliable and valid.
Regulatory Hurdles
Navigating the regulatory landscape for head electrode technologies poses another set of challenges. With the increasing complexity of these devices, regulatory bodies require comprehensive testing for safety and efficacy before they can be utilized in clinical practice. The long approval timelines can hinder innovation and delay the clinical application of new technologies.
There is also a need for harmonization among different regions regarding regulations. Variability in approval processes can confuse manufacturers and slow down the introduction of novel electrode systems. Thus, greater dialogue between industry stakeholders and regulatory agencies is essential for enabling accelerated pathways without compromising safety.
Addressing these challenges in head electrode development will enhance their performance, safety, and broader applicability across scientific and medical fields.
Future Directions in Head Electrode Research
Research into head electrodes is evolving rapidly. This growth stems from the need for enhanced monitoring and therapeutic techniques in neuroscience and clinical applications. Understanding future directions is critical for researchers and practitioners. It highlights innovations in materials, expands clinical use, and integrates technology with artificial intelligence. Each of these elements plays a role in advancing the field, addressing current limitations, and improving patient outcomes.
Innovative Materials Exploration
The exploration of new materials is pivotal for enhancing the functionality of head electrodes. Traditional materials often limit performance due to issues like biocompatibility, long-term stability, and signal fidelity. One promising area is the use of nanomaterials. These materials can offer superior electrical properties and can be engineered for specific functions
- Graphene: Known for its conductivity and flexibility. Potentially enhances the sensitivity of electrodes.
- Conductive Polymers: These can improve biocompatibility while retaining good electrical conduction. They may reduce inflammation when used in invasive applications.
- Hydrogels: These materials provide excellent skin contact for non-invasive sensors. They can adapt to body movements, increasing comfort and data accuracy.
"The selection of materials determines the capability of head electrodes, influencing both research and therapy outcomes."
Each new material can lead to advancements in how electrodes interact with brain tissue. Ultimately, this could facilitate better data acquisition and long-term use in patients.
Expanded Clinical Applications
The potential clinical applications for head electrodes is vast and growing. As technologies improve, two primary areas of focus emerge: diagnostics and treatment. In diagnostics, electrodes play a role in improving the accuracy of electroencephalograms and other brain imaging techniques. Enhanced designs could allow for greater spatial resolution and signal clarity.
In terms of treatment, electrodes are increasingly significant in neurostimulation therapies. Areas for expanded use include:
- Epilepsy Management: More precise electrode placements may help control seizures better than current methods.
- Neurofeedback: Applications for mental health, aiming to teach patients self-regulation of brain activity, may benefit from advanced electrode technologies.
- Rehabilitation: For stroke patients, using electrodes to monitor brain activity can enhance recovery protocols and tailor therapy accordingly.
These applications show potential for improving quality of life, highlighting the critical need for ongoing research and development in this area.
Integration with AI and Machine Learning
The integration of AI and machine learning in head electrode research presents opportunities to revolutionize data analysis and application outcomes. These technologies can process vast amounts of data collected from electrodes. This processing could lead to more accurate interpretations of brain signals, identifying patterns invisible to the human eye.
- Predictive Analytics: AI algorithms can analyze trends in brain activity, predicting possible health issues before they manifest as symptoms.
- Automated Diagnostics: Machine learning frameworks can assist in diagnosing conditions based on real-time data from electrodes, reducing reliance on subjective interpretations.
- Tailored Treatments: Integrating patient data with AI-driven models enables personalized treatment plans based on individual responses, improving overall effectiveness.
AI continues to transform how we understand and interact with brain activity data, making the future of head electrodes even more promising.