Genetics Unraveled: Principles and Implications
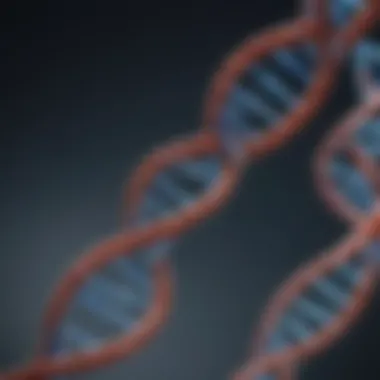
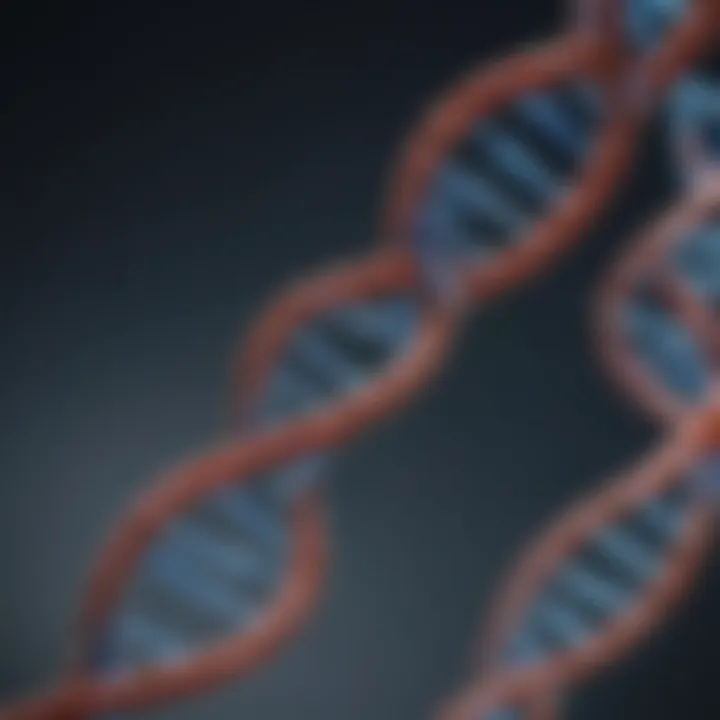
Intro
Genetics stands as a cornerstone of modern biology, providing insights into the fundamental processes of life. This field focuses on how traits are passed from one generation to the next and how biological variations arise. The complex interactions between genes and the environment shape not only individual characteristics but also species evolution and adaptation.
In recent years, advances in genetic research have contributed significantly to various domains, including medicine, agriculture, and environmental science. For example, understanding genetic mutations can lead to innovative treatments for genetic disorders, while insights into plant genetics support sustainable agriculture.
As we embark on exploring the complexities of inheritance, it is essential to consider both the historical context and current advancements. Gene editing technologies, such as CRISPR, exemplify how these innovations are transforming traditional genetic research. However, the enthusiasm for these developments must be weighed against ethical considerations that arise in their application.
This article endeavors to illuminate the multifaceted nature of genetics, emphasizing the interplay of inheritance, environmental factors, and ethical dimensions in shaping future research. Through this exploration, we aim to foster a thoughtful dialogue among students, educators, and professionals, ensuring an informed perspective as we navigate the complexities of genetic science.
Research Overview
Summary of Key Findings
Genetic research has unveiled numerous key findings that have reshaped our understanding of biology. Some prominent points include:
- The discovery of DNA as the molecule of heredity, leading to the realization of how traits are encoded.
- Insights into the role of genes in diseases, prompting genomic medicine's rise.
- Evidence of epigenetic factors influencing gene expression, emphasizing that environment interacts intricately with genetics.
Relevance to Current Scientific Discussions
The realm of genetic research continues to be highly relevant in scientific discussions today. Notable contributions include:
- The impact of genomics on personalized medicine, offering tailored treatments based on an individual's genetic makeup.
- The ongoing debates surrounding genetic privacy and the implications of utilizing genetic information, especially regarding genetic testing.
- The potential of gene editing to address environmental issues, such as climate change impacts on agriculture.
As we expand our inquiry, the comprehension of these topics becomes increasingly crucial as it encourages informed discussions in the scientific community and beyond.
Intro to Genetics
Genetics is the scientific study of heredity and variation in living organisms. It plays a foundational role in many aspects of biology, influencing how traits are passed from one generation to the next. Understanding genetics is essential not just for researchers and educators, but also for anyone interested in the biological sciences. This section aims to clarify the significance of genetics within this article, highlighting its relevance across various contexts.
The exploration of genetics is not just an academic exercise. It has tangible benefits, including advancements in medicine, agriculture, and biotechnology. By comprehending genetic principles, individuals can better appreciate how living systems function, adapt, and evolve. Additionally, genetics informs practices such as selective breeding in agriculture and the development of gene therapies in medicine.
During this article, we will uncover how genetics serves as a bridge between theoretical concepts and practical applications.
Defining Genetics
Genetics encompasses various biological concepts related to genes, heredity, and genetic variations. A gene is a unit of heredity that is transferred from a parent to an offspring and is responsible for a specific trait. Genes consist of DNA, which also holds instructions for the growth and development of organisms.
The study of genetics encompasses numerous subfields, including molecular genetics, population genetics, and quantitative genetics, each providing unique insight into the complexities of inheritance.
This definition highlights the fundamental aspects of what encompasses genetics, establishing a groundwork for the ensuing discussions in the article.
The Role of Genetics in Biology
Genetics is an integral part of biology that influences various biological disciplines. It provides crucial insights into how organisms develop, evolve, and interact with their environment. The role of genetics can be seen in several key areas:
- Evolutionary Biology: Understanding genetic variation is vital for studying evolution. Natural selection acts on these variations, driving species change over time.
- Medicine: Genetic research leads to enhanced understanding of diseases, paving the way for personalized medicine and targeted therapies.
- Agriculture: Knowledge of genetics facilitates genetic modification and selective breeding, which enhances crop yields and improves resistance to pests.
- Conservation: Genetic studies can assist in preserving endangered species through understanding population genetics and biodiversity.
Historical Context of Genetic Research
The field of genetics has evolved significantly over time, and understanding this historical context provides essential insights into how current methodologies and ideas have been shaped. By analyzing past advancements and the contributions of pivotal figures, it becomes clear how genetics has revolutionized biology and medicine. This background not only highlights the achievements but also raises awareness about ongoing challenges and ethical issues that researchers face today.
Key Figures in Genetics
Gregor Mendel
Gregor Mendel, often referred to as the father of genetics, conducted his groundbreaking experiments on pea plants in the mid-19th century. He established fundamental principles of inheritance through statistical analysis of trait variations. Mendel's work highlighted the idea of dominant and recessive traits, laying the foundation for modern genetic theory. His meticulous methodology and formulation of the laws of inheritance are crucial for this article, as they serve as a backdrop against which many subsequent genetic discoveries were made. Mendel's unique feature was his ability to apply mathematical models to biological phenomena, a practice that was rare at the time. This characteristic provided a strong empirical basis for his conclusions, making his contributions both reliable and significant.
James Watson
James Watson is best known for his role in discovering the structure of DNA alongside Francis Crick in 1953. The duo proposed the double-helix model, which provided profound insights into how genetic information is stored and replicated. Watson's key characteristic was his innovative approach to scientific inquiry, relying heavily on experimental data. This article benefits from discussing Watson's contributions, as he helped shift the focus of genetics from mere observational studies to a more molecular perspective. Watson’s discovery, however, raised some ethical considerations about the implications of understanding genetic material that are crucial to the ongoing dialogue regarding genetics.
Francis Crick
Francis Crick, in collaboration with James Watson, made significant strides in understanding DNA's structure. Crick's analytical abilities and creative thinking played a vital role in interpreting the findings that led to the elucidation of DNA's role in heredity. The importance of Crick's contributions to this article is immense. His work not only confirmed existing theories but also opened new pathways for research in genetics and molecular biology. The unique aspect of Crick’s legacy is the emphasis on the interdisciplinary approach; his work encompassed biology, chemistry, and physics, which is a valuable model for future genetic endeavors.
Landmark Discoveries
Mendelian Inheritance
Mendelian inheritance refers to the inheritance patterns discovered by Gregor Mendel, which govern how traits are transmitted from parents to offspring. This fundamental principle informs a vast array of studies within genetics. The key characteristic of Mendelian inheritance is that it presents a clear framework for understanding genetic variations. Its simplicity allows for applicability across diverse organisms, making it a beneficial choice for this article. The unique feature here is the predictability of inheritance based on dominance and recessiveness. This clarity aids both research and education, though it may oversimplify complex inheritance patterns found in nature.
Discovery of DNA Structure
The discovery of DNA structure in 1953 marked a monumental leap in genetic research. It provided a tangible model of how genetic information is encoded and passed through generations. The key characteristic of this discovery is the double-helix configuration, which elegantly explains the mechanism of replication and mutation in genes. This breakthrough is beneficial for this article as it links the physical structure of DNA to its functional implications in inheritance. The unique aspect of this discovery lies in its far-reaching impact on various fields, fostering advancements in medicine, biotechnology, and evolutionary biology.
Human Genome Project
The Human Genome Project, completed in 2003, was an international research initiative aimed at mapping the entire human genome. This project has had profound implications for understanding genetic predispositions to diseases and variations among individuals. Its key characteristic is its comprehensive approach, which has made vast amounts of genetic data accessible to researchers. For this article, the project's extensive data provides a crucial foundation for discussing genetic disorders and personalized medicine. The unique feature of the Human Genome Project is its collaborative nature, uniting scientists worldwide, though it has also raised concerns regarding data privacy and ethical use of genetic information.
Fundamental Principles of Genetics
Understanding the fundamental principles of genetics is essential for exploring how traits and characteristics are passed down through generations. This section provides a vital framework for interpreting complex biological concepts. Genetics is the study of heredity and variation in organisms. This discipline forms the backbone of many scientific fields, including medicine, agriculture, and evolutionary biology. By focusing on the basic principles, we allow readers to grasp the implications of genetic research on health, disease, and biodiversity.
Genes and Chromosomes
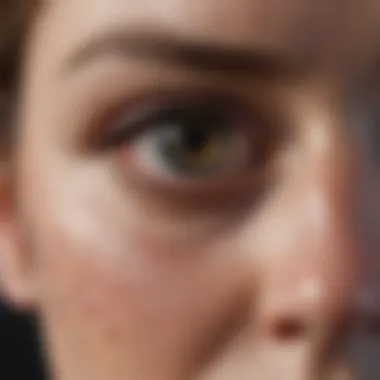
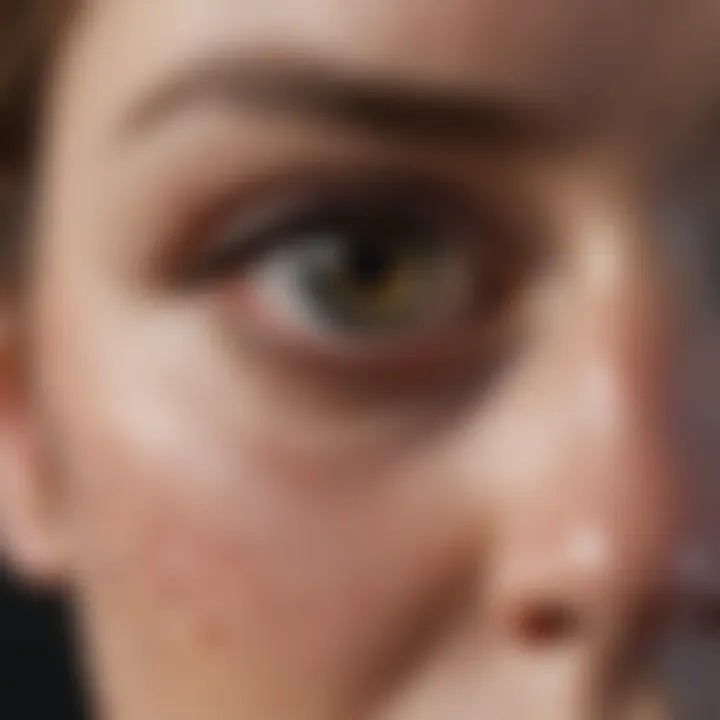
Genes are the basic units of heredity. They are segments of DNA that carry the instructions for producing proteins, which perform various functions in the body. Chromosomes are structures within cells that contain genes. Humans have 23 pairs of chromosomes, each composed of DNA coiled tightly around proteins. The organization and integrity of these structures are crucial for maintaining genetic information.
Key Characteristics:
- Structure: Genes reside on chromosomes, with each chromosome representing a distinct set of genetic instructions.
- Functionality: Genes are responsible for traits such as eye color, hair type, and susceptibility to certain diseases.
Understanding how genes and chromosomes interact provides insights into genetic disorders and guiding principles essential for genetic testing and therapies.
Inheritance Patterns
Inheritance patterns define how traits are transmitted from parents to offspring. They are categorically divided into several types, including autosomal dominant, autosomal recessive, and X-linked inheritance. Each of these patterns presents unique characteristics and implications for genetic research.
Autosomal Dominant
Autosomal dominant inheritance is characterized by the presence of a disease-causing allele on one of the non-sex chromosomes. A single copy of this allele can cause the disorder. This type of inheritance can be observed across generations, often resulting in a 50% chance that an offspring will inherit the trait from an affected parent.
Key Characteristics:
- High Penetrance: Disorders often appear in every generation.
- Benefit: Understanding this pattern is crucial for predicting risks in families and informing genetic counseling.
However, while it can be straightforward to identify in families, autosomal dominant conditions can vary significantly in expressivity, which can complicate diagnosis and management.
Autosomal Recessive
In autosomal recessive inheritance, a child must inherit two copies of a recessive allele—one from each parent—to express the disorder. If both parents carry one recessive allele but are unaffected, there is a 25% chance of their child expressing the condition.
Key Characteristics:
- Carrier Status: Many individuals can be carriers without showing symptoms.
- Benefit: This model highlights the importance of population screening and awareness of recessive disorders in specific demographics.
One challenge of this inheritance type is that traits may skip generations, complicating family history records.
X-Linked Inheritance
X-linked inheritance occurs when the gene causing the trait or disorder is located on the X chromosome. Males (XY) are more likely to express X-linked disorders because they have only one X chromosome. Females (XX) can be carriers if they have one affected X chromosome but typically do not show symptoms unless both X chromosomes are affected.
Key Characteristics:
- Male Bias: More common in males, leading to notable differences in prevalence between the sexes.
- Benefit: Understanding X-linked patterns is critical for diagnosing and managing conditions such as hemophilia and Duchenne muscular dystrophy.
This type of inheritance reinforces the idea that sex chromosomes play a unique role in inheritance, influencing disease risk and response to therapies.
Understanding the inheritance patterns is crucial in genetics. It helps in the identification of genetic disorders and the development of effective treatment strategies.
Molecular Genetics Techniques
Molecular genetics techniques play a critical role in understanding the foundational elements of genetics. These methods allow researchers to manipulate genetic material, enabling the exploration of genes and their functions at an unparalleled level of detail. The ability to analyze DNA and RNA sequences forms the backbone of modern genetic research. By employing these techniques, researchers can uncover the molecular basis of diseases, develop new therapies, and explore the potentials of genetic engineering.
DNA Sequencing
DNA sequencing is a fundamental technique that enables the determination of nucleotide sequences in DNA. This method is crucial for mapping genomes and identifying genetic variations that contribute to diseases. Sequencing has revolutionized genetics by enabling scientists to read and interpret the genetic code, providing insights into genetic disorders and hereditary diseases.
Sanger Sequencing
Sanger sequencing is the first widely adopted method of DNA sequencing. It is known for its accuracy and reliability. Sanger's method involves the selective incorporation of chain-terminating dideoxynucleotides during DNA synthesis. This results in DNA fragments of varying lengths, which can be separated by capillary electrophoresis for analysis. A key characteristic of Sanger sequencing is its efficacy in sequencing relatively short DNA fragments, typically up to 1000 base pairs.
Sanger sequencing remains popular due to its high fidelity and clear results. However, it is time-consuming and costlier than newer methods, limiting its efficiency for large-scale projects. Its unique capability lies in providing precise quality data essential for contexts where accuracy is paramount, yet the throughput is lower compared to next-generation sequencing.
Next-Generation Sequencing
Next-generation sequencing (NGS) represents a significant advancement over Sanger sequencing. NGS allows for massively parallel sequencing, meaning thousands of DNA fragments can be sequenced simultaneously. This technique is instrumental for whole-genome sequencing and large-scale projects. A significant advantage of NGS is its speed and cost-effectiveness, making it a preferred choice for genomic studies.
The unique feature of NGS is its ability to generate vast amounts of data in a short time. However, the complexity of data analysis can be a disadvantage, as it often requires sophisticated bioinformatics tools and expertise. The advantages of NGS make it a pivotal tool in addressing many genetic research questions, including the role of genetics in disease.
Gene Editing Technologies
Gene editing technologies have emerged as pivotal tools in molecular genetics. These allow scientists to modify an organism's DNA with precision, holding the potential to revolutionize medicine and agriculture. The ability to edit genes opens doors to innovative treatments for genetic disorders and the development of crops with desirable traits.
CRISPR-Cas9
The CRISPR-Cas9 system is a groundbreaking gene-editing technology that has transformed molecular genetics. This system uses a guide RNA to direct the Cas9 nuclease to specific sequences within the DNA. Its key characteristic is the ease with which it can be designed to target almost any gene. This flexibility makes CRISPR-Cas9 a beneficial choice for diverse applications in research and therapy.
One unique feature of CRISPR-Cas9 is its efficiency and accuracy in influencing genetic changes. The technology allows for straightforward modifications, like inserting or deleting genes. While its advantages are significant, ethical concerns regarding potential off-target effects and implications for germline editing remain vital considerations in its adoption.
TALENs
Transcription Activator-Like Effector Nucleases (TALENs) are another powerful tool for gene editing. TALENs work by utilizing a DNA-binding domain and a nuclease domain to create double-strand breaks at specific locations in the genome. This method is highly specific and can achieve precise edits.
TALENs' key characteristic is their adaptability, allowing researchers to construct tailored nucleases for targeted genes. They offer a robust option for applications requiring very specific genetic modifications. However, TALENs can be more complex and labor-intensive to design compared to CRISPR, which may hinder their wider use in some research environments.
In summary, molecular genetics techniques, including DNA sequencing and gene editing technologies like CRISPR-Cas9 and TALENs, are essential for advancing genetics research. These methodologies provide tools that not only deepen our understanding of genetic mechanisms but also pave the path for innovative applications in medicine and agriculture.
The Genetics of Disease
The genetics of disease plays a critical role in comprehending the underlying mechanisms that contribute to various health conditions. Understanding genetic factors is essential for discerning how traits and susceptibilities are inherited. This section elucidates the pathways through which genes influence the likelihood of disease, particularly focusing on genetic and environmental interplays.
Genetic Disorders
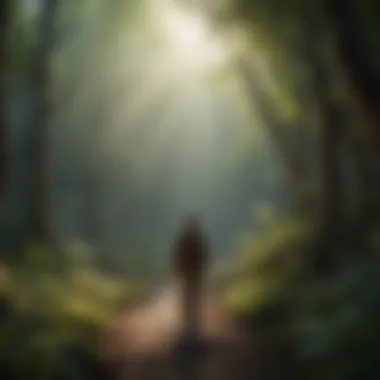
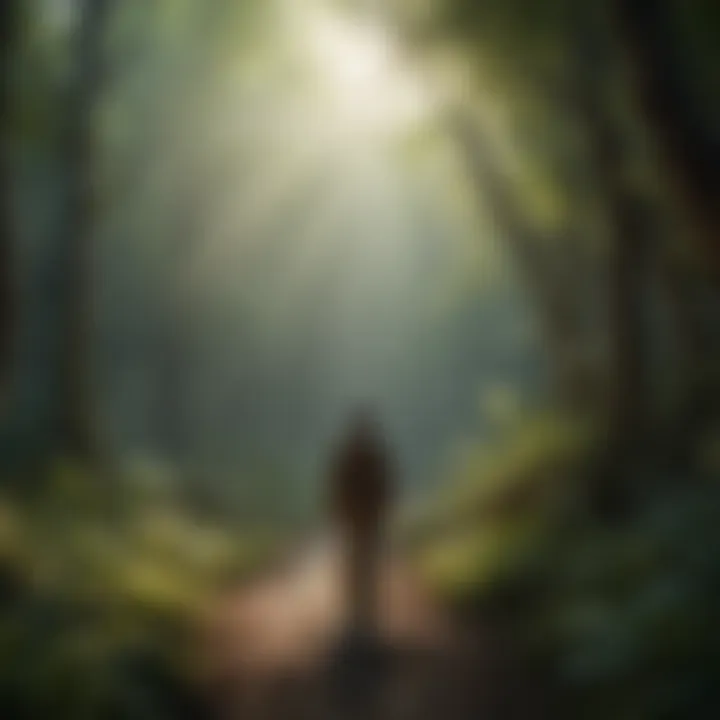
Genetic disorders arise from abnormalities within the genetic material. These disorders can be categorized into two main types: monogenic and polygenic disorders.
Monogenic Disorders
Monogenic disorders are caused by mutations in a single gene. This specificity is a key characteristic that makes these disorders easier to study within the context of genetics. The Mendelian inheritance explains how traits pass down through generations, making monogenic disorders valuable for understanding inheritance principles in genetics. Conditions like cystic fibrosis or sickle cell anemia exemplify such disorders.
A distinctive feature of monogenic disorders is their predictability. They follow clear inheritance patterns, which allows for focused research and targeted gene testing. This predictability can be beneficial for early diagnosis and treatment strategies. However, they can also have significant implications for affected individuals and their families, including potential psychological impacts and societal stigma.
Polygenic Disorders
Polygenic disorders involve multiple genes contributing to the development of the disease. This complexity introduces challenges in pinpointing specific genetic causes. Conditions like diabetes and schizophrenia illustrate such polygenic influences.
A key feature of polygenic disorders is the multifactorial nature of their inheritance. Unlike monogenic disorders, they do not follow straightforward patterns. This makes it harder to predict their occurrence solely based on family history. The interplay between multiple genetic factors and environmental influences highlights the challenge in studying these disorders. Despite these complications, understanding polygenic disorders remains essential because they account for a significant portion of human diseases.
Cancer Genetics
Cancer genetics examines how genetic variations contribute to the risk and development of cancer. This area is especially vital given that cancer is a leading cause of death worldwide. Genetic mutations, both inherited and acquired, can initiate tumorigenesis or influence how cancers progress.
Research shows that certain hereditary syndromes increase cancer risk significantly. For example, mutations in the BRCA1 and BRCA2 genes are linked with breast and ovarian cancers, indicating the importance of genetic screening for at-risk individuals. The ability to understand these genetic predispositions allows for more precise prognoses, targeted therapies, and preventive measures.
Understanding cancer genetics is essential for developing personalized medicine strategies that tailor treatments based on an individual’s genetic profile.
Overall, the genetics of disease encompasses a wide array of factors and implications. It guides research towards understanding heritable patterns and offers insight into how various disorders can be managed or prevented.
Epigenetics and Gene Expression
Epigenetics and gene expression represent crucial elements in the understanding of how genetic material translates into observable traits. This exploration goes beyond mere genetic sequences to include mechanisms that regulate when and how genes are activated. These epigenetic factors influence not just an individual organism's characteristics but also heritable traits across generations. The interactions between genes and their expression can determine susceptibility to diseases, response to therapies, and overall biological function. This section illuminates the importance of epigenetics and gene expression in comprehending genetic intricacies.
Understanding Epigenetics
Epigenetics is the study of heritable changes in gene activity that do not involve alterations to the underlying DNA sequence. This field has emerged as a vital aspect of genetics, focusing on how environmental factors and lifestyle can induce changes in the gene expression profile. One significant realization from epigenetic studies is that the same set of genes can lead to vastly different outcomes, depending on their expression at any given time.
The understanding of epigenetics has broad implications for fields such as developmental biology, cancer research, and therapeutic developments. It encourages an integrated perspective, merging genetic science with environmental interactions, and offers new avenues for research and treatment.
Factors Affecting Gene Expression
Gene expression is influenced by a variety of factors. Understanding these influences is vital for addressing genetic research challenges.
Environmental Influences
Environmental influences play a significant role in shaping gene expression. This aspect encompasses a range of factors, including diet, stress, toxins, and social interactions. The importance of these influences lies in their potential to modify genetic expression in real-time, suggesting that our environment shapes not just behavior but physiological traits as well. The unique characteristic of environmental influences is their ability to create temporary changes in gene activity, which can have both short-term and long-term effects on the organism.
Moreover, the implications of environmental influences are profound. They highlight that while genetic predispositions exist, these can be modulated by subsequent environmental factors, emphasizing a dynamic view of genetics. However, one disadvantage is that this variability makes it complex to predict outcomes based solely on genetic information.
Cellular Context
Cellular context is another key factor impacting gene expression. Each cell type can express different genes despite having the same DNA, depending on its specific environment and developmental stage. This characteristic defines the role of epigenetics on a cellular level, showing how gene expression can differ in various tissues or organs.
It is a beneficial feature for this article as it illustrates the sophisticated regulation of gene expression. Recognizing that not all cells respond uniformly to environmental cues allows researchers to personalize approaches in medicine and other fields. The disadvantage here is that understanding cellular context requires extensive research and a high level of specificity, complicating broader applications of genetic findings.
Genetics and Environment
The relationship between genetics and environment is crucial in understanding how traits are expressed and how organisms adapt to their surroundings. This section highlights the significant interactions between genetic predisposition and environmental factors, addressing key points such as the fundamentals of nature versus nurture, gene-environment interactions, and their implications in various fields.
Nature vs. Nurture
The age-old debate of nature versus nurture explores how much of an individual’s characteristics come from genetic inheritance versus environmental influences. On one side, genetics provide the blueprint for physical features, personality traits, and susceptibility to certain diseases. On the other side, the environment shapes these inherent traits through experiences, culture, and social context. Scientific studies indicate that while genetics set limits on development, environmental factors can modify, enhance, or restrict those genetic potentials.
Here is a brief overview of some core aspects involved:
- Genetic Influence: Certain traits, like eye color, are solely determined by genetic makeup, while many behavioral traits arise from a complex interplay of genes and the environment.
- Environmental Impact: Factors such as nutrition, climate, and societal conditions contribute heavily to health outcomes and behavior patterns.
- Complex Interplay: Recognizing that neither genetics nor environment works in isolation is vital; both interact in a dynamic manner throughout an individual’s development.
By exploring these dynamics, researchers gain insight into areas such as psychology, public health, and education.
Gene-Environment Interactions
Gene-environment interactions refer to the ways in which an individual's genetic predispositions can be influenced by environmental factors. This interplay shows how external conditions can shape genetic effects. It is important to understand these interactions because they offer insights into disease mechanisms, behavioral traits, and overall well-being.
Some significant aspects include:
- Variability in Response: Different individuals with the same genetic makeup may react differently to environmental factors. For example, some may become stressed in certain situations, while others do not.
- Epigenetic Changes: Environmental factors can lead to epigenetic modifications, affecting how genes are expressed without altering the underlying DNA sequence. This can include changes due to diet or exposure to toxins.
- Research Implications: Understanding gene-environment interactions is crucial for developing personalized medicine approaches that consider both genetic and environmental influences when treating conditions like cancer or mental health disorders.
In summary, the ongoing exploration of the genetics and environment relationship aids in dissecting the complexities of inheritance and variation. Recognizing how traits emerge from these interactions presents opportunities for advancements in medicine, psychology, and much more.
"To fully understand human behavior, considering both genetic backgrounds and environmental contexts is essential. Ignoring either aspect leads to an incomplete picture."
This comprehensive assessment highlights the necessity of integrating various perspectives when analyzing genetic expression and its implications in diverse fields.
Applications of Genetic Research
Genetic research has profound implications across various fields. Advances in this area lead to developments that address some of the most pressing questions in modern science, health, agriculture, and beyond. The application of genetic principles and techniques is not only transformative but also necessary for improving quality of life and understanding complex biological systems.
In medical settings, genetics allows for better diagnosis, treatment, and prevention of diseases. The insights provided by genetic research help identify mutations and genetic predispositions that may lead to certain conditions. This knowledge can guide clinicians in crafting personalized treatment plans based on an individual’s genetic profile. Furthermore, advancements in gene therapy open doors to potential cures for genetic disorders, which previously remained unmanageable.
In agriculture, genetics plays a critical role in enhancing crop yield, improving resistance to pests and diseases, and increasing nutritional value. Through genetic engineering and selective breeding, scientists can create crops that are better suited to varying environmental conditions. This not only aids in food security but also in sustainable agricultural practices.
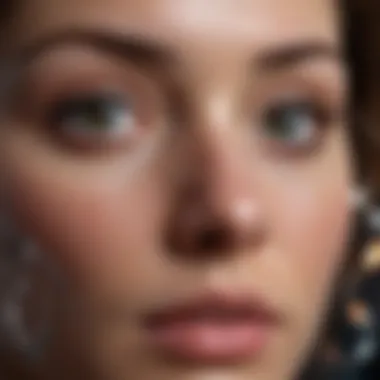
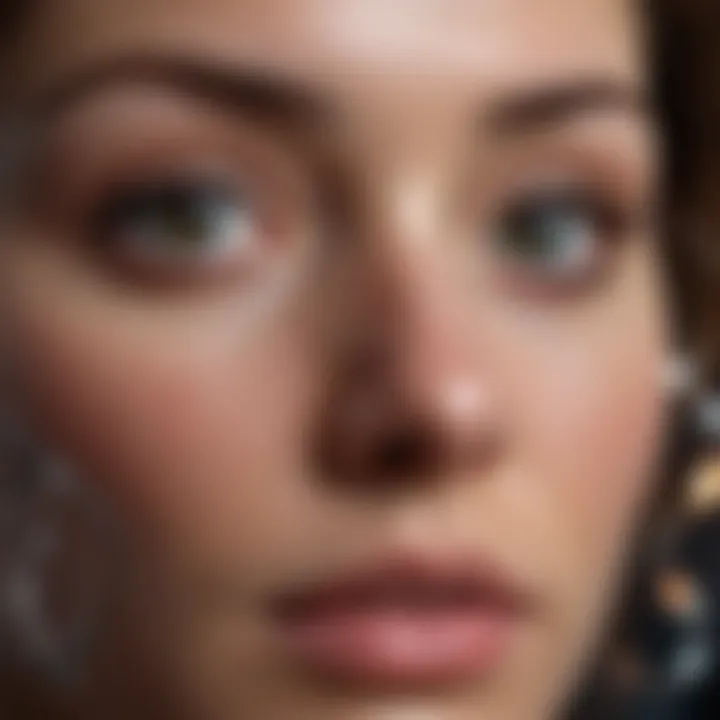
"The field of genetic research serves as a bridge between complex science and practical applications that can yield real-world benefits."
Both sectors highlight the necessity of integrating genetic research into existing systems, such as healthcare and food production. Yet, these applications are not without their challenges and ethical considerations. The complexities involved require a careful approach that balances innovation with responsibility.
Medical Genetics
Medical genetics revolves around the study of hereditary diseases and their genetic underpinnings. One significant area is genetic testing, which can identify inherited disorders before symptoms appear. This facilitates early intervention and treatment, ideally mitigating the impact on patients. Genetic counseling also plays an essential role in helping individuals understand their risks and the implications of testing.
The advent of precision medicine, which tailors treatment to the individual's genetic makeup, is a critical development in this field. Pharmacogenomics, for instance, examines how genes affect a person’s response to drugs. This ensures that individuals receive medications that are both effective and safe for them, minimizing adverse effects.
Agricultural Genetics
Agricultural genetics focuses on the genetic enhancement of plants and animals to improve yield and resistance. Techniques such as recombinant DNA technology have allowed geneticists to isolate and manipulate specific genes, leading to the development of genetically modified organisms (GMOs). These organisms often exhibit traits such as drought resistance or enhanced nutritional profiles that are vital for food security as global populations expand.
Another aspect is marker-assisted selection, which accelerates traditional breeding by identifying and selecting plants with desired traits at the genetic level. This method combines conventional breeding with modern genetic techniques, ensuring a higher success rate in producing robust crops.
Ultimately, the applications of genetic research are extensive, promising, and essential for progress in contemporary society. As science continues to advance, the implications of these applications will only grow, opening new avenues for exploration and innovation.
Ethical Considerations in Genetics
The realm of genetics is not merely a compilation of scientific discoveries; it also embodies a complex web of ethical considerations that have profound implications for individuals and society. As advancements in genetic research continue to evolve, interest in ethics becomes increasingly pertinent. This section examines critical aspects of gene editing, genetic testing, and the privacy of genetic information, providing insights that are essential for students, educators, and professionals alike.
Gene Editing Ethics
Gene editing technologies, particularly CRISPR-Cas9, have revolutionized the ability to modify genetic material. While these technologies offer tremendous potential for curing genetic disorders and improving agricultural efficiency, they raise significant ethical dilemmas. Questions of "Should we intervene in human germline editing?" emerge as researchers and practitioners navigate the moral landscape.
One of the primary concerns is the potential for designer babies, where genetic modifications could be used not just for disease prevention but also for enhancing desirable traits. This prospect prompts a debate about equity. If gene editing becomes accessible only to the affluent, it could exacerbate social inequalities. Moreover, there is uncertainty surrounding the unforeseen consequences of altering the human genome. Thus, the ethical principle of precaution advocates for rigorous oversight in gene editing practices.
Another critical aspect is obtaining informed consent, particularly when it involves editing genes of embryos. Parents’ decisions may be influenced by societal pressures or misconceptions about the capabilities of gene editing. Failures in this area can lead to ethical breaches that compromise individual autonomy and parental rights.
Genetic Testing and Privacy Issues
Genetic testing has become a vital tool for diagnosing inherited conditions and informing treatment options. However, this powerful tool bears ethical implications, particularly concerning privacy. The data generated from genetic tests can reveal sensitive information not only about the individual but also about their relatives.
Concerns about data protection and consent are paramount. Who owns the data? How is it used? Public trust in genetic testing is crucial; if individuals fear misuse of their genetic information, this can deter them from seeking necessary tests. The law currently lags behind technological advancements, creating gaps in regulations that must be addressed.
Furthermore, there are scenarios where genetic information may be utilized against individuals. Employers or insurers could potentially discriminate based on genetic predispositions. Establishing robust laws to ensure that genetic information remains confidential and protected is essential in maintaining ethical standards.
The ethical terrain in genetics is not static; it demands continuous engagement from society, policymakers, and researchers.
In summary, as we delve into genetic research, the importance of ethical considerations increasingly becomes clear. Topics such as gene editing ethics and genetic testing privacy form the foundation of discourse necessary for responsible use of genetic technologies. Addressing these concerns is crucial in ensuring that scientific advancements benefit humanity while respecting individual rights.
Future Directions in Genetic Research
Genetic research is at a critical juncture, presenting numerous avenues for inquiry and potential breakthroughs. Understanding future directions in this field is paramount. It not only influences academic discourse but also shapes real-world applications. As we observe ongoing advancements, it remains essential to recognize how these innovations can address pressing health issues and improve agricultural practices.
Emerging Technologies
Emerging technologies are transforming the landscape of genetic research. One notable technology is CRISPR-Cas9. This gene-editing tool enables precise alterations to DNA sequences. The implications are vast, ranging from correcting genetic disorders to enhancing crop resistance. Another significant advancement is single-cell sequencing. This technique allows researchers to examine genetic variations at the individual cell level, providing insights into cellular diversity and disease progression.
A few more noteworthy technologies include:
- Synthetic Biology: This involves designing and constructing new biological parts and systems. The applications range from renewable energy production to biopharmaceuticals.
- Whole Genome Sequencing (WGS): This technology facilitates a complete analysis of an organism’s entire genome. It is vital for personalized medicine, enabling tailored treatment plans based on individual genetic makeup.
The integration of these technologies paves the way for innovative solutions in healthcare and agriculture. The complexities of genetic research demand a careful approach to ensure ethical consideration while harnessing such powerful tools for the betterment of society.
Interdisciplinary Approaches
The future of genetic research increasingly involves interdisciplinary collaboration. Crossing traditional boundaries leads to holistic insights. Collaborative efforts among geneticists, bioinformaticians, and environmental scientists yield a deeper understanding of gene-environment interactions. Additionally, incorporating perspectives from social sciences can guide ethical practices in genetic engineering and testing.
Interdisciplinary research facilitates:
- Broader Perspectives: Understanding the implications of genetic research requires insights that span multiple fields.
- Innovative Solutions: Collaboration often leads to novel approaches for complex biological problems, driving progress in various industries.
- Ethical Frameworks: Understanding societal implications ensures that research is conducted responsibly.
As genetic research continues to evolve, the integration of diverse disciplines will be crucial. This collaborative approach not only enriches scientific inquiry but also prepares the scientific community to tackle emerging challenges more effectively.
"The collaboration between different scientific domains is essential for realizing the full potential of genetic discoveries."
Closure
The conclusion serves as a critical reflection on the insights gained from the various discussions on genetics throughout this article. This section synthesizes the key learnings, emphasizing the complex interplay of genetic principles and their real-world implications.
In summary, genetics is not just a branch of science concerned with heredity; it is the very foundation of biological identity, influencing everything from physical traits to susceptibility to diseases. By understanding the mechanisms behind inheritance, we can better appreciate how genes shape the diversity of life and how they interact with environmental factors. This knowledge has profound implications for fields such as medicine and agriculture, leading to innovations that can improve human health and food security.
Key takeaways from the article include:
- The historical milestones that have shaped our understanding of genetics.
- The techniques and methodologies currently in use, such as CRISPR and next-generation sequencing.
- The ethical considerations surrounding genetic testing and editing, which require careful thought.
Ultimately, the importance of this conclusion lies in its potential to inspire further research and dialogue. Genetics is continually evolving, and the ongoing advancements highlight the need for ethical awareness. It also calls for interdisciplinary collaboration to navigate the complexities of genetic research in future developments.
Summary of Key Insights
The exploration of genetics highlights several vital points:
- Inheritance Patterns: Understanding how traits are passed from one generation to another is essential for grasping the biological basis of diversity.
- Molecular Techniques: Advances in sequencing and editing technologies are revolutionizing how we study genetics, thus opening new avenues for research.
- Ethical Frameworks: As we harness genetic knowledge, ethical considerations must guide our practices to ensure that we protect individual rights and societal values.
The interconnectedness of these topics demonstrates that the study of genetics is dynamic and requires ongoing scrutiny and reflection.
The Ongoing Importance of Genetics
Genetics plays a crucial role in numerous sectors beyond basic biological science. Its implications stretch into medicine, environmental science, and even sociology. For instance, understanding one’s genetic makeup can inform personalized medicine approaches, which tailor treatments to individuals based on their genetic profile. Clinical research increasingly relies on genetic data to identify disease predispositions, allowing for earlier interventions and better patient outcomes.
Moreover, advancements in agricultural genetics promise to optimize crop yields and reduce the impact of pests. These applications showcase not only the versatility of genetic research but also its potential to address significant global challenges. As we progress into an era where gene editing technologies become more prevalent, their responsible use must remain a focal point of governance and public discourse.