Exploring the MZM Modulator: Principles and Applications
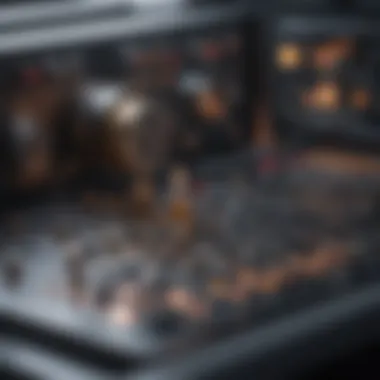
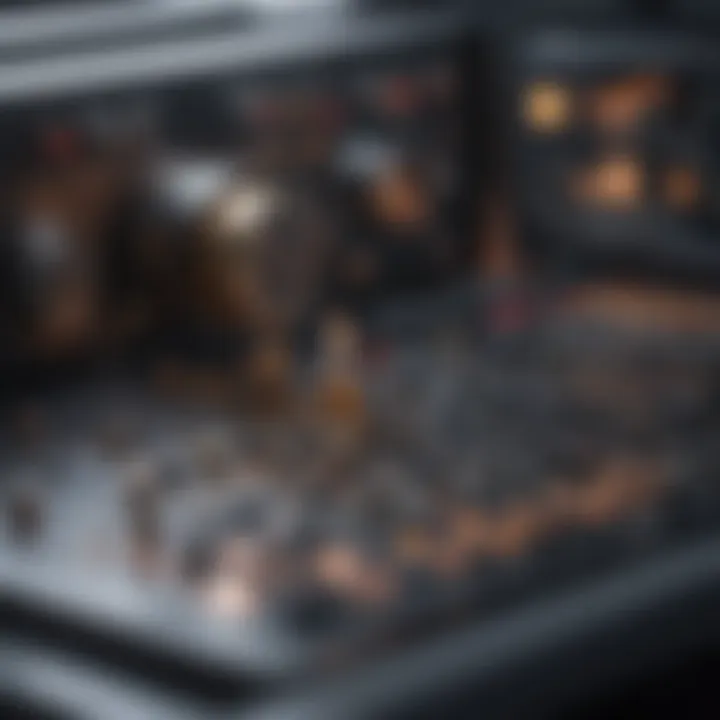
Intro
The Mach-Zehnder Modulator (MZM) plays a crucial role in optical communication systems, which is essential for modern connectivity. Understanding how it operates is more than just a technical pursuit; it is about appreciating the underpinnings of how data travels across the globe in the blink of an eye. This section sets the stage for a deeper dive into the MZM's principles, its applications, and future directions that could shape its role in the optical technology landscape.
Research Overview
Summary of Key Findings
This article examines the fundamental principles that guide the operation of the Mach-Zehnder Modulator. The device relies on interference effects, manipulating light paths to achieve modulation. Key findings reveal that MZMs are essential for high-speed data transmission, providing both amplitude and phase modulation capabilities. The analysis also covers various configurations of MZMs, each with distinct advantages and challenges that affect performance in real-world applications.
Relevance to Current Scientific Discussions
In the current landscape of optical technologies, MZMs are at the forefront. Their ability to accommodate increasing data rates and bandwidth requirements aligns with trends in telecommunications. Notably, discussions around 5G implementations have spotlighted the necessity for sophisticated modulation techniques like those provided by MZMs. Furthermore, as industries push toward greener technologies, understanding the efficiency of MZMs in various settings becomes even more pertinent.
Methodology
Research Design and Approach
The analysis presented here employs both qualitative and quantitative research methodologies. By reviewing existing literature and conducting experimental measurements, the article aims to present a well-rounded view of the mechanisms at play within MZMs. This dual approach not only underlines theoretical constructs but also addresses real-world performance metrics.
Data Collection and Analysis Techniques
Data regarding MZM performance have been collected from various vetted sources, including peer-reviewed journals and conference papers. Analysis techniques include comparative studies that assess prevailing designs against current technological standards. This not only enriches the discourse on MZM performance but also highlights areas that are ripe for innovation and development.
Through understanding the MZM's operational intricacies, implications for upcoming trends, and future advancements, this article aspires to provide clarity and insight that could benefit students, educators, researchers, and professionals alike. The interplay of these factors will ultimately shape the trajectory of optical communication as we know it.
Prolusion to MZM Modulators
The Mach-Zehnder Modulator (MZM) plays a crucial role in the realm of optical communication, making it a worthwhile topic of exploration in this article. Its design and function significantly influence the efficiency of data transmission over long distances. By modulating the amplitude, phase, or frequency of light signals, MZMs ensure high-speed and high-fidelity data communication, which is essential in modern networks.
Understanding MZM modulators encompasses various aspects such as
- their operational principles,
- historical development,
- types of configurations,
- performance metrics, and
- current applications.
These elements will highlight how MZMs optimize optical fibers and enhance the capability of telecommunications, data transfer, and emerging technologies like quantum computing.
Definition and Overview
A Mach-Zehnder Modulator is an optical device that employs interference principles to control light. Specifically, it consists of two beam splitters and two optical paths. The modulator can adjust the phase of the light in one path, creating a phase difference when the light beams recombine. This phase shift leads to constructive or destructive interference, thus modulating the intensity of the output light. This effect is particularly useful for generating precise optical signals that can represent binary data.
MZMs are pivotal in applications requiring high data rates, low loss, and minimal distortion. They serve as key components within optical communication systems, making them indispensable for modern technologies.
Historical Context
The concept of the Mach-Zehnder Modulator traces back to the early developments of photonics in the mid-20th century. With the advent of laser technology in the 1960s, the utility of light for communication became apparent, setting the stage for advancements in optical devices, including MZMs.
In the 1970s and 1980s, researchers began to explore various modulating techniques. The MZM quickly gained traction for its superior qualities compared to other modulation methods such as electro-absorption or direct modulation. As telecom infrastructure grew, particularly during the dot-com boom, MZMs emerged as a backbone for high-speed optical networks.
Today, MZMs continue to evolve, incorporating advanced materials and designs that push the boundaries of data transmission capabilities. This evolution reflects not only technological advancements but also the increasing demand for bandwidth in our interconnected world.
"The development of the MZM has been instrumental in shaping the landscape of fiber-optic communications since the 1970s, facilitating unprecedented data rates and network efficiency."
The history of MZM technology demonstrates a trajectory of innovation, establishing a foundation for further research and application in both existing and emerging fields.
Fundamental Principles of Operation
In the realm of optical communication, the Mach-Zehnder Modulator (MZM) serves as a cornerstone technology. Understanding the underlying principles that govern its operation reveals the intricate mechanics enabling efficient modulation of light. The MZM's utility stems from its ability to manipulate light waves, facilitating advancements in data transfer and telecommunications. This section elaborates on two critical aspects: the interferometric effect and the phase shift mechanism.
Interferometric Effect
The interferometric effect is fundamental to the operation of an MZM. This phenomenon arises when two coherent light waves, derived from a single light source, interact. In an MZM, light is split into two paths through a beam splitter, allowing it to experience different phase shifts before being recombined. The recombination occurs at a second beam splitter, where the light waves can interfere either constructively or destructively, depending on the phase difference they acquired in their respective paths.
This effect is crucial because it determines how the modulator alters the intensity of the light output based on the voltage applied to the device. As the applied voltage changes, so does the phase difference between the two light waves. This interaction results in varying output intensity, which encodes the information being transmitted. The interferometric effect thus establishes the MZM as a robust tool for encoding data onto optical signals.
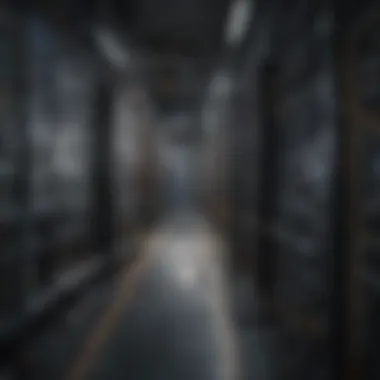
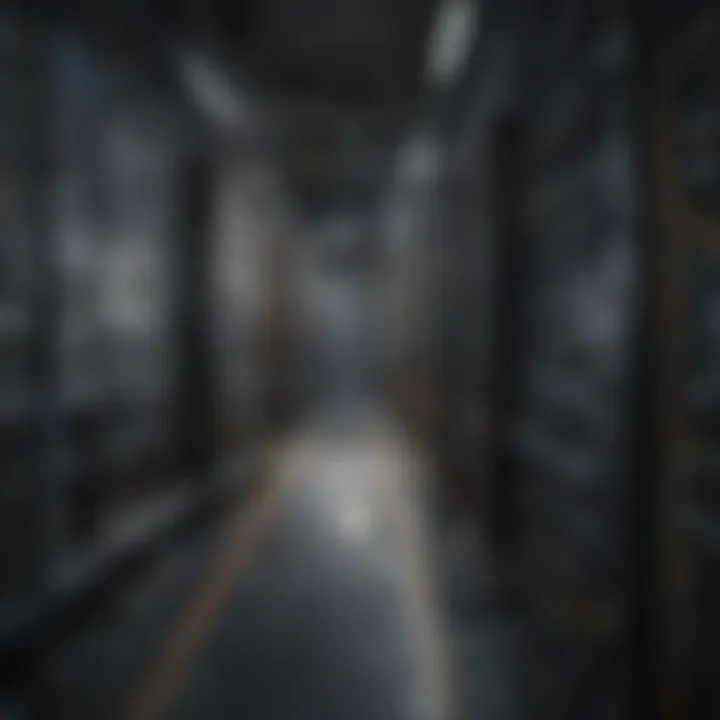
The MZMโs ability to modulate light based on phase shifts enables high-speed data transmission essential for modern communication networks.
Phase Shift Mechanism
The phase shift mechanism is intrinsically linked to the operational efficiency of the MZM. This mechanism involves the changes in the optical path length as light travels through different media or materials in the modulator. Specifically, applying an electrical voltage across the modulator alters the refractive index of the waveguide material, which in turn modifies the phase of the light propagating through that section.
Key considerations regarding the phase shift mechanism include:
- Material Selection: Various materials exhibit different electro-optic coefficients. Choosing a material with a high electro-optic coefficient allows for greater modulation efficiency. Typical examples are Lithium Niobate or Indium Phosphide.
- Voltage Application: The optimal voltage range is essential to ensure accurate phase shifts without excessive power consumption. This optimization influences both performance and thermal stability.
- Device Design: The design of the waveguide configurations and the beam splitter arrangement can affect the degree of phase shift achieved. Ensuring an optimal design promotes better performance metrics, including bandwidth and extinction ratio.
In summary, the fundamental principles of operation for MZMs not only enhance our understanding of optical modulation techniques but also provide insights into the methodologies for improving existing systems. The interferometric effect and the phase shift mechanism are critical aspects that make MZMs integral components in todayโs fast-paced optical communication landscape.
Types of MZM Configurations
Understanding the types of MZM configurations is crucial for optimizing performance in various applications of optical communication. Each configuration serves specific functions and has distinct advantages and considerations. The choice of configuration can significantly impact modulation depth, bandwidth, and overall system efficiency. Choosing the right type ensures the desired balance between complexity, performance, and application needs.
Single-Drive MZM
Single-drive Mach-Zehnder Modulators utilize one input for modulation. This type is simpler in design and easier to implement, which can make it attractive for specific applications. It operates by altering the phase of an optical signal based on the applied electric field. This configuration is often found in systems where lower bandwidth is acceptable.
Benefits of single-drive MZM include:
- Simplicity: Fewer components mean easier integration into existing systems.
- Lower cost: Typically, they require less complex circuitry, leading to reduced production costs.
- Power Efficiency: Generally consumes less power compared to other configurations.
However, single-drive modulators do have limitations. These include reduced modulation depth and bandwidth, making them less suitable for high-speed applications.
Dual-Drive MZM
Dual-drive Mach-Zehnder Modulators have two independent drive inputs. This setup allows for greater control over the modulation process. By applying signals to both arms of the interferometer, these modulators can achieve higher modulation depths and improved linearity. They are particularly effective in high-speed applications where bandwidth is critical.
Advantages of dual-drive MZM include:
- Increased Performance: Higher modulation efficiency leads to better signal quality.
- Enhanced Bandwidth: Suitable for applications requiring high data rates, such as broadband communication.
- Flexible Operation: This configuration allows for optimized signal processing in diverse environments.
The complexity and cost of dual-drive MZMs are higher than single-drive options, which could be a factor in some designs.
Hybrid Configurations
Hybrid MZM configurations combine elements from both single-drive and dual-drive systems. This allows for tailored solutions that maximize advantages from each type while minimizing drawbacks. For example, hybrid models might use dual-drive principles for main operations while integrating the simplicity of single-drive configurations for specific tasks or feedback systems.
Key aspects of hybrid configurations include:
- Customizability: Designers can tailor performance characteristics suited to the application needs.
- Improved Performance Metrics: Enhances both modulation efficiency and bandwidth while retaining simplicity.
- Versatility: Can be adapted for a variety of scenarios in telecommunications and data transfer.
The flexibility of hybrid configurations makes them appealing for future advancements in optical modulation technologies.
Hybrid configurations indicate a significant advancement in the performance and application of MZMs, merging efficiency with customization.
Key Performance Metrics
Understanding the key performance metrics of Mach-Zehnder Modulators (MZMs) is essential for assessing their effectiveness in various applications. These metrics serve as indicators of how well a modulator can perform under specific conditions and in different configurations. Optimizing these parameters is crucial for enhancing the overall efficiency and reliability of optical communication systems. This section delves into the three primary performance metrics of MZMs: modulation bandwidth, insertion loss, and extinction ratio.
Modulation Bandwidth
Modulation bandwidth refers to the range of frequencies over which an MZM can effectively modulate an optical signal. This metric is vital because it determines how fast the modulator can respond to changes in the input electrical signal. A higher modulation bandwidth enables the transmission of information at greater data rates, making it essential for modern high-speed optical communication systems.
Factors that influence modulation bandwidth include the physical design of the modulator and the materials used in its construction. Recent advancements in semiconductor technology have allowed for increased bandwidth capabilities in MZMs. Typically, modulation bandwidth is measured in gigahertz (GHz), and modulator designs that exceed 30 GHz are considered high-performance.
Insertion Loss
Insertion loss is another critical metric in evaluating the performance of MZMs. It refers to the loss of optical power resulting from the insertion of the modulator into the optical path. This loss can occur due to factors such as mismatched waveguide structures, poor coupling efficiency, or intrinsic material losses.
Minimizing insertion loss is crucial since high levels can degrade the overall signal quality and lead to increased power requirements. The objective is to achieve a balance between efficient modulation and minimal power loss. Typically, good MZMs exhibit an insertion loss of less than 5 dB.
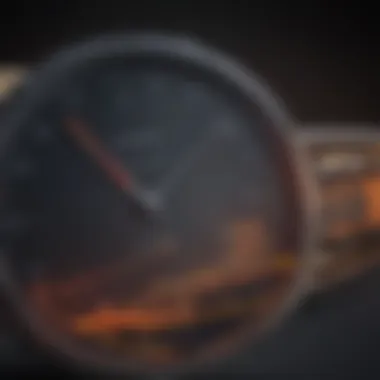
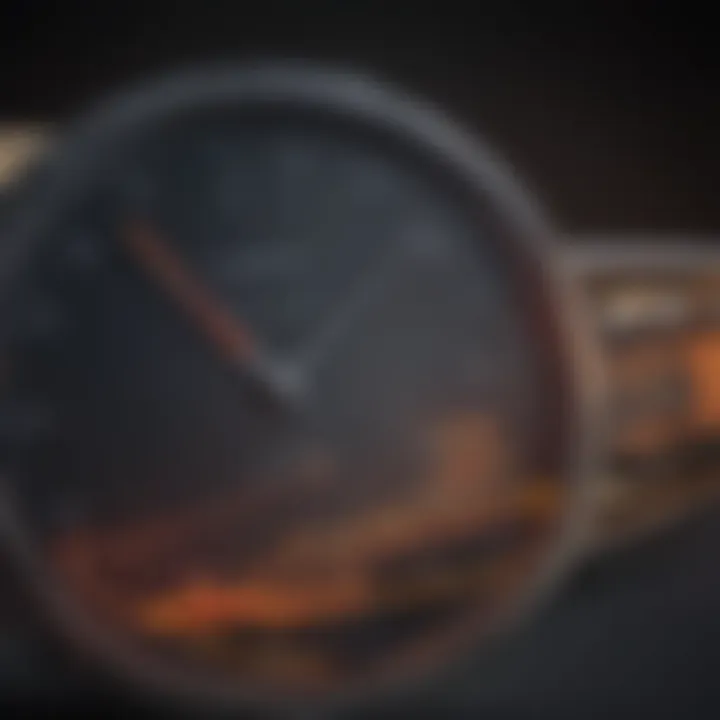
Extinction Ratio
Extinction ratio (ER) quantifies the effectiveness of the MZM in discriminating between the 'on' and 'off' states of the light signal. This metric is defined as the ratio of the optical power in the 'on' state to the power in the 'off' state. A higher extinction ratio indicates a greater ability to produce distinct states, which directly affects the clarity and quality of the transmitted optical signal.
Extinction ratio is particularly relevant in applications where signal integrity is critical, such as in telecommunications and data centers. Good performance in this area typically reflects an extinction ratio greater than 20 dB. Enhancements in the design of MZMs have aimed at achieving even higher ratios, thus improving overall system performance.
In summary, the key performance metrics of modulation bandwidth, insertion loss, and extinction ratio play a crucial role in the effectiveness of MZMs and their applicability in optical communication systems. Optimizing these parameters is essential for ensuring high data rates and reliable transmission in future optical networks.
Applications of MZM Technology
The applications of MZM technology are critical in todayโs evolving landscape of optical communication. The flexibility and efficiency of Mach-Zehnder modulators enhance signal integrity and decrease latency in various sectors. This section discusses key application areas, detailing their significance and the advantages offered by MZM technology.
Telecommunications
Telecommunications are a primary field benefiting from MZM technology. The use of Mach-Zehnder modulators allows for high-speed data transmission over long distances. With the growing demand for bandwidth, MZMs provide the necessary capacity to transfer large volumes of data with minimal distortion.
Some key benefits of MZMs in telecommunications include:
- High Modulation Rate: MZMs can operate in the gigahertz range, enabling rapid data transfer.
- Signal Quality: By reducing phase noise, these modulators ensure clearer signals, which is vital for voice and video calls.
- Scalability: They can be easily integrated into existing optical networks, allowing for future upgrades without significant infrastructure changes.
In addition to bandwidth efficiency, MZMs support advanced modulation formats. Techniques like QPSK (Quadrature Phase Shift Keying) and DPSK (Differential Phase Shift Keying) are possible, which further enhance data rates and capacity.
Data Centers
The role of MZMs extends to data centers, which are essential for storing and processing massive amounts of data. MZMs contribute to efficient optical interconnects that link servers, enabling faster communication between components.
Key considerations for MZMs in data centers include:
- Reduced Latency: Minimizing delays in data transfer is crucial for performance. MZMs facilitate swift communication, improving overall efficiency.
- Energy Efficiency: MZMs tend to consume less power compared to other modulation technologies, which is increasingly important in large-scale data processing environments.
- Density: Their compact design allows for high-density configurations. This is crucial as data centers aim to maximize space usage while minimizing heat production.
Integrating MZMs into data center architecture is not merely advantageous; it is rapidly becoming a necessity as businesses rely more heavily on cloud services and real-time data processing.
Optical Signals in Quantum Computing
In the realm of quantum computing, MZMs are gaining traction due to their ability to handle optical signals with precision. The development of quantum technologies often requires modulation of light signals to achieve effective data encoding and transfer.
MZMs contribute to quantum computing applications by:
- High Fidelity: MZMs ensure that the coherence of quantum states is maintained, which is vital for the success of quantum algorithms.
- Pulse Shaping: They allow for the precise shaping of light pulses needed for quantum information processing.
- Interfacing Technologies: MZMs can interface well with various optical components, making them versatile tools in quantum communication setups.
The emergence of quantum technologies signifies a shift in how data is processed and transferred. MZMs play an integral part in pioneering such advancements, facilitating faster and more secure information exchanges.
"MZM technology is not just transforming data transmission; it is a foundation for future advancements in fields like quantum computing."
Technological Advances in MZM Design
Technological advancements in Mach-Zehnder Modulator (MZM) design have transformed its role in optical communication systems. Understanding these improvements is critical for those engaged in both developing new technologies and implementing existing systems. The integration of MZMs into diverse applications relies heavily on these innovations, which offer enhanced performance and functionality. This section evaluates two main areas of advancement: integration with photonic circuits and improved fabrication techniques.
Integration with Photonic Circuits
The integration of MZMs with photonic circuits represents a significant milestone in optical technology. This synergy facilitates miniaturization and increases the operational efficiency of devices. Photonic integrated circuits (PICs) combine multiple optical functions on a single chip. Integrating MZMs into these circuits enables a compact design that minimizes space without compromising performance.
Some key benefits of this integration include:
- Reduced Signal Loss: MZMs embedded within photonic circuits can lower insertion loss due to shorter optical paths.
- Enhanced Functionality: These systems can perform several functions, such as modulation and filtering, simultaneously.
- Cost-Effectiveness: Reduced material usage and sophisticated design lead to lower overall costs.
Photonic integrations allow for the marriage of optical and electronic functionalities, enabling more extensive applications in telecommunications and data processing. The ability to produce highly efficient modulators in smaller footprints is pivotal in satisfying the rising demand for high-speed optical communications.
Improved Fabrication Techniques
The improvement of fabrication techniques has played a crucial role in the evolution of MZMs. Traditional methods have constraints. New approaches enhance the manufacturing process, making it more efficient and flexible.
Recent developments focus on several aspects:
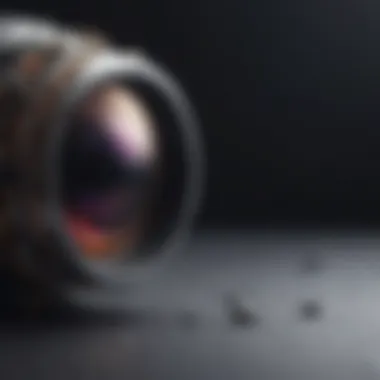
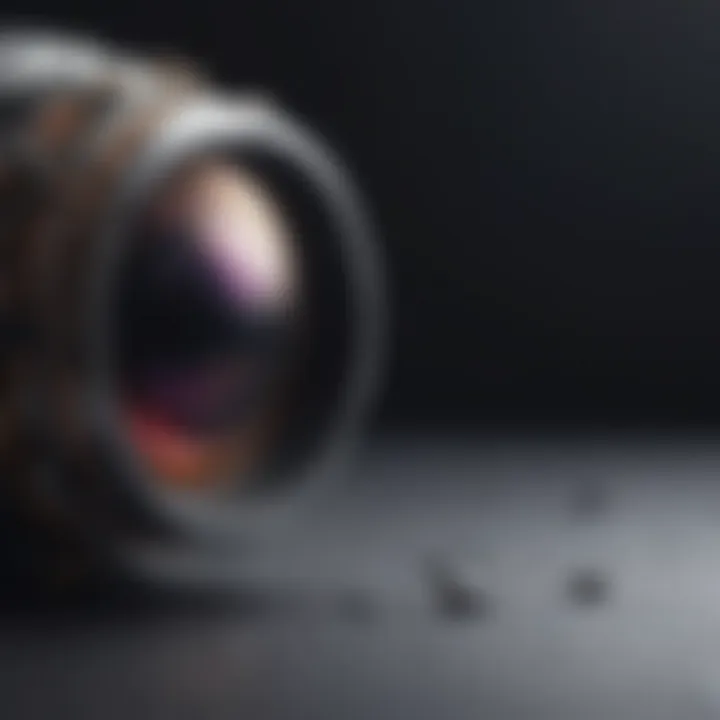
- Material Innovation: New materials, such as silicon photonic materials, improve performance characteristics. They provide better confinement of light and higher modulation speeds.
- Nanofabrication Techniques: Using advanced lithography and etching methods, manufacturers can create smaller and more precise structures. This leads to better control over light propagation and modulation.
- Automation and Quality Control: Advanced automated systems increase production reliability and reduce human error, ensuring uniformity in MZM performance across batches.
These improved fabrication techniques are vital for reducing costs and increasing the scalability of MZM production. As the demand for optical components expands, these advancements enable manufacturers to keep pace with industry requirements.
"The future of MZMs lies in their integration with photonics and improved manufacturing processes, paving the way for next-generation optical devices."
Through the examination of technological advances in MZM design, one can appreciate how they enhance both the capabilities and applications of these modulators in various domains. As optical networks evolve, these innovations will ensure MZMs remain at the forefront of communication technologies.
Challenges in MZM Deployment
The deployment of Mach-Zehnder Modulators (MZMs) in various optical communication systems presents unique challenges that must be addressed to ensure optimal performance and reliability. Understanding these challenges is essential for researchers, engineers, and decision-makers involved in the deployment of MZM technology. Two significant areas of concern include temperature sensitivity and cost efficiency.
Temperature Sensitivity
Temperature sensitivity is a notable challenge for MZMs, impacting their performance and reliability. MZMs operate based on precise phase shifts induced by the applied electric field. However, variations in temperature can lead to fluctuations in the refractive index of the materials used in the modulator. This sensitivity can cause unwanted phase shifts and, consequently, distort the modulated optical signal.
The temperature-induced changes can result in:
- Degradation of signal quality: Fluctuating phase shifts can lead to variations in the intensity and quality of the signal, affecting overall communication performance.
- Increased error rates: As the signal quality diminishes, the likelihood of bit errors can increase, which is detrimental in high-speed data applications.
To combat temperature sensitivity, robust thermal management solutions must be integrated into the MZM design. This can include the use of temperature sensors, thermal insulation, and active temperature control systems to maintain optimal operating conditions.
Cost Efficiency
Cost efficiency is another critical factor when considering the deployment of MZMs. The initial cost of fabricating and integrating MZR components can be substantial. Alongside fabrication costs, ongoing operational costs must also be taken into account. Cost efficiency impacts the overall viability of MZM deployment in commercial settings.
Several factors contribute to cost efficiency in MZM deployment:
- Material selection: The choice of materials used in the production of MZMs can significantly affect fabrication costs. Advanced materials may offer better performance but may also increase expenses.
- Manufacturing processes: Innovations in fabrication techniques can reduce costs while maintaining the desired performance levels. Streamlining manufacturing processes through automation and better design can lead to significant cost savings.
- Economies of scale: Increasing production volume can help reduce per-unit costs, making MZMs more accessible for widespread use in various applications.
To address cost efficiency, stakeholders must balance the benefits of advanced technologies with budget considerations. Collaborations between manufacturers and researchers can lead to innovations that enhance both performance and cost-effectiveness in MZM technology.
"Innovation in manufacturing processes is essential for ensuring that Mach-Zehnder Modulators remain a competitive choice in the ever-evolving landscape of optical communication systems."
In summary, overcoming challenges related to temperature sensitivity and cost efficiency is paramount for the successful deployment of MZMs in optical communication networks. Addressing these hurdles will enable the full potential of MZM technology to be realized in various applications, ensuring reliable and efficient signal modulation.
Future Trends in MZM Modulation
The future of MZM (Mach-Zehnder Modulator) modulation promises to drive significant advancements in optical communication technologies. Understanding these trends is crucial for researchers and professionals focused on improving transmission systems and enhancing data rates. As we explore the next steps in MZM modulation, we will see its essentiality in addressing the growing demands for bandwidth and connectivity.
Next-Generation Optical Networks
Next-generation optical networks are set to redefine the landscape of telecommunications. The shift towards more sophisticated and efficient systems is imperative. These new networks leverage MZM technology to enable faster data transmission with lower latency. Key attributes that define the role of MZMs in these networks include:
- Higher Data Rates: As bandwidth demands increase, MZMs facilitate higher data rates, supporting the advancement of 5G networks and beyond.
- Improved Signal Integrity: Enhanced modulator designs contribute to maintaining signal quality over longer distances. This helps reduce errors in data transmission.
- Integration with Software-Defined Networking: MZMs are increasingly integrated into software-defined networks (SDN), allowing for dynamic bandwidth allocation and improved network management.
The integration of MZMs into next-generation optical networks speaks to their adaptability and importance in future telecom infrastructures.
Advancements in Data Rate Capabilities
MZM technology continues to evolve, especially in terms of data rate capabilities. This evolution is vital as digital communication demands grow exponentially. Developments are being made in several areas:
- Next-Gen Modulation Formats: New modulation schemes, such as quadrature amplitude modulation (QAM), are being implemented with MZMs to enhance the bit rate without requiring an increase in bandwidth.
- Miniaturization Techniques: Advances in fabrication are reducing the physical size of MZMs, which allows for more compact designs while boosting performance and efficiency.
- Thermal Management Innovations: Improved thermal management technology helps maintain optimal performance of MZMs, reducing the susceptibility to temperature variations and ensuring reliability over time.
To summarize, advancements in data rate capabilities through MZM improvements hold significant implications for the future of optical networks.
"The drive for higher data rates and lower costs in telecommunications will significantly influence the landscape of MZM technology."
Epilogue
The conclusion of this article underscores the pivotal role that the Mach-Zehnder Modulator (MZM) plays within optical communication systems. Understanding the MZM is crucial for grasping the advancements and challenges of modern telecommunications and data transfer technologies. The insights gained throughout the article not only highlight the distinct operational principles of MZMs but also their diverse applications in fields such as telecommunications and quantum computing.
Summary of Key Insights
Key insights from the previous sections showcase the complexity and versatility of MZM technology. We explored the fundamental principles of operation, including the interferometric effects and phase shift mechanisms that dictate performance. The examination of different configurations, from single-drive to hybrid designs, illustrated how engineers optimize these devices for various applications. Furthermore, we discussed metrics like modulation bandwidth, insertion loss, and extinction ratio, which are essential for evaluating MZM performance in real-world scenarios. In terms of applications, the MZM plays a central role in enhancing communication infrastructures, especially in telecommunications and data centers.
Implications for Future Research
The discussion on future trends reveals significant avenues for research and development. As telecommunications demands continue to grow, researchers must focus on next-generation optical networks that leverage MZM technology for higher data rates. Additionally, innovations in material science and nanofabrication techniques may pave the way for more efficient and cost-effective modulators. These advancements allow better integration with photonic circuits, which is vital for the evolution of optical devices. Future research can also explore the challenges of temperature sensitivity and cost-efficiency, which directly affect deployment in practical scenarios. A strong emphasis on these aspects will ensure that the MZM continues to meet the escalating demands of the digital age.