Exploring Real-Time Quantitative PCR in Depth
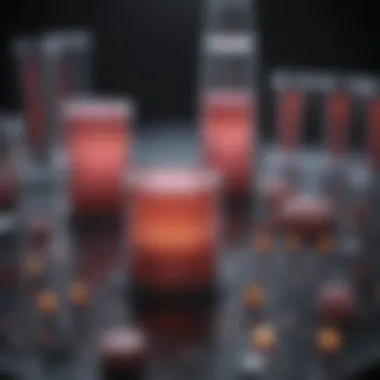
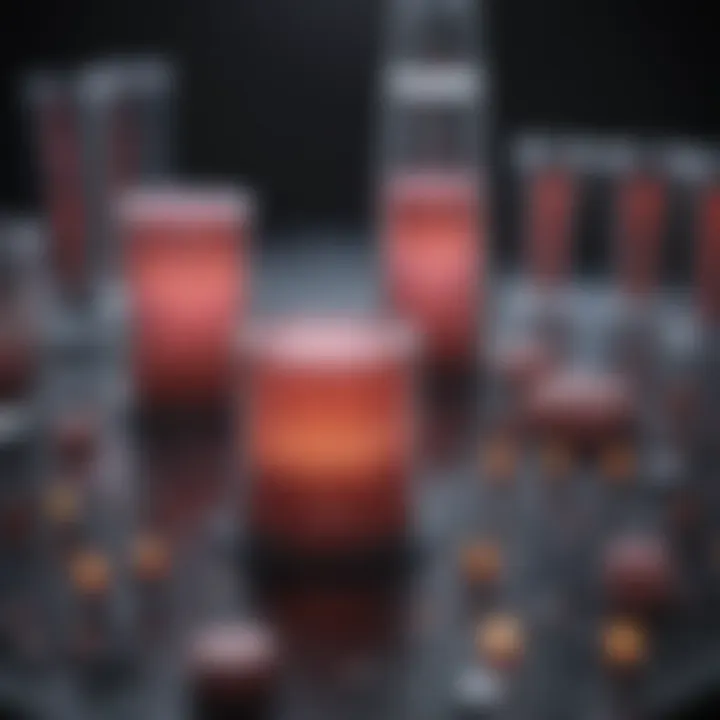
Intro
Real-time quantitative polymerase chain reaction, commonly known as qPCR, stands at the forefront of molecular biology techniques. As we explore this powerful tool, it becomes evident that its value extends well beyond mere amplification of DNA. Instead, qPCR provides a nuanced understanding of gene expression levels, allowing researchers to make significant strides in various biological investigations.
Research Overview
Summary of Key Findings
Recent studies demonstrate that qPCR excels in sensitivity and specificity, often considered the gold standard for quantifying nucleic acids. This methodology allows for the detection of minute quantities of DNA or RNA, making it indispensable in fields such as genetics, oncology, and infectious disease research. Researchers have uncovered its versatility in clinical diagnostics, where timely and accurate data can alter treatment pathways.
Among the findings, a particular emphasis has been placed on the importance of reference genes in the normalization process. Accurate interpretation of results hinges on selecting appropriate control genes, which reflect consistent expression across various sample conditions. Moreover, advancements in software and analysis techniques have optimized data reporting, ensuring precision in quantification.
Relevance to Current Scientific Discussions
In contemporary discussions of molecular diagnostics, qPCR continues to be a pivotal topic. The ongoing evolution of technology enhances its application in real-time monitoring, such as during the COVID-19 pandemic. The capacity for rapid and reliable results prompted a shift in how we approach diagnostics and research alike.
Encouragingly, qPCR applies not just to disease detection but also in research avenues like genetic engineering and synthetic biology. The ability to measure gene expression profiles lays a foundation for innovative therapies and personalized medicine.
Methodology
Research Design and Approach
The framework of qPCR encompasses several integral phases, starting from sample preparation to the final analysis of findings. A well-structured design includes rigorous controls and replicates to enhance the reliability of results. For instance, in experimental setups, researchers must consider factors like enzyme efficiencies and PCR conditions, as these directly affect data outcomes.
Data Collection and Analysis Techniques
Data collection in qPCR is underpinned by real-time detection methods, typically employing fluorescent dyes or probes. During amplification, fluorescence signals correlate with the amount of target nucleic acid present. The use of standard curves helps ascertain the concentration of target sequences in unknown samples, showcasing the technique’s precision.
The subsequent data analysis phase is critical. Researchers utilize software applications for quantitative analyses. Key metrics include threshold cycle (Ct) values, which indicate when a fluorescent signal surpasses background levels. Proper interpretation of these values is crucial to derive meaningful conclusions.
As researchers continue to explore advancements in qPCR technology, the potentials seem vast. The integration of high-throughput platforms and machine learning algorithms could further streamline analyses, making qPCR even more accessible and efficient for a wider audience of professionals.
"The evolution of qPCR has not only enhanced molecular biology but has also paved the way for groundbreaking research applications."
Preface to Real-Time Quantitative PCR
Real-Time Quantitative PCR, commonly known as qPCR, is a pivotal technique in molecular biology that allows researchers to quantify DNA in real-time during the amplification process. This method has transformed various fields, including clinical diagnostics, genetic research, and pathogen detection. The significance of qPCR lies not only in its ability to provide quantitative data but also in its speed and sensitivity, making it an essential tool for scientists and healthcare professionals.
The implementation of qPCR presents numerous advantages. Firstly, it combines traditional PCR technology with advanced fluorescence detection methods. This combination allows for the continuous monitoring of the amplification of specific DNA sequences. As a result, scientists can determine the quantity of DNA in a sample while it is being amplified, rather than waiting until the end of the process. This real-time aspect is crucial because it minimizes contamination risks and provides quicker results.
In this exploration of qPCR, we will delve into its definition, historical milestones, underlying principles, and the technological advances that have shaped its current applications. Understanding the foundations of qPCR is vital for researchers aiming to apply this technology effectively in their studies.
Definition of Real-Time qPCR
Real-Time qPCR is a laboratory technique used to amplify and simultaneously quantify a targeted DNA molecule. Unlike traditional PCR, where the detection occurs after amplification, qPCR measures the accumulation of DNA during the PCR process. It utilizes specific fluorescent dyes or probes that emit fluorescence upon binding to the amplified DNA, allowing for real-time monitoring of the reaction.
The quantification aspect can be achieved through two primary methods: absolute and relative quantification. Absolute quantification provides a direct measure of the target DNA amount, while relative quantification assesses the target in relation to a reference gene. This flexibility makes qPCR a versatile tool in both research and clinical applications.
Historical Context
The journey of qPCR began in the early 1990s when researchers sought to improve the capabilities of traditional PCR methods. The introduction of fluorescent dyes and probes was a significant advancement that allowed for the quantification of DNA during amplification. This marked a pivotal shift from the conventional methodologies that only provided qualitative results post-reaction.
In 1996, the first real-time PCR instrument was introduced, which further broadened its use in laboratories. Over the years, various brands, such as Applied Biosystems with its TaqMan technology, emerged, enabling researchers to adopt more refined techniques. As technology advanced, the cost of qPCR systems decreased, thus paving the way for wider adoption across numerous disciplines, from clinical diagnostics to environmental monitoring.
"Real-Time qPCR has revolutionized the way researchers approach DNA quantification, greatly enhancing both speed and accuracy in molecular diagnostics."
This article will provide in-depth insights into the workings of qPCR, its essential components, optimization strategies, and its myriad applications. Understanding these elements will empower readers to leverage this technology effectively in their respective fields.
Underlying Principles of qPCR
The underlying principles of real-time quantitative polymerase chain reaction (qPCR) provide a foundational understanding essential for effective application and interpretation in various fields. This section focuses on two critical components: the DNA amplification mechanism and fluorescence detection methods. Both aspects are crucial for measuring DNA concentration and accuracy in quantitative analysis. Understanding these concepts not only enhances the effectiveness of experimental protocols but also improves the reliability of the results obtained.
DNA Amplification Mechanism
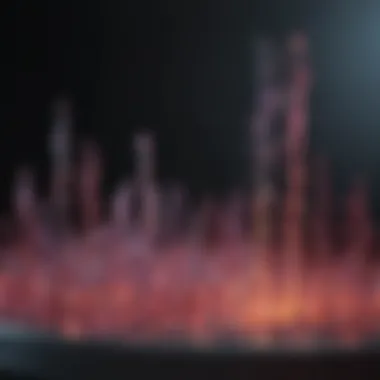
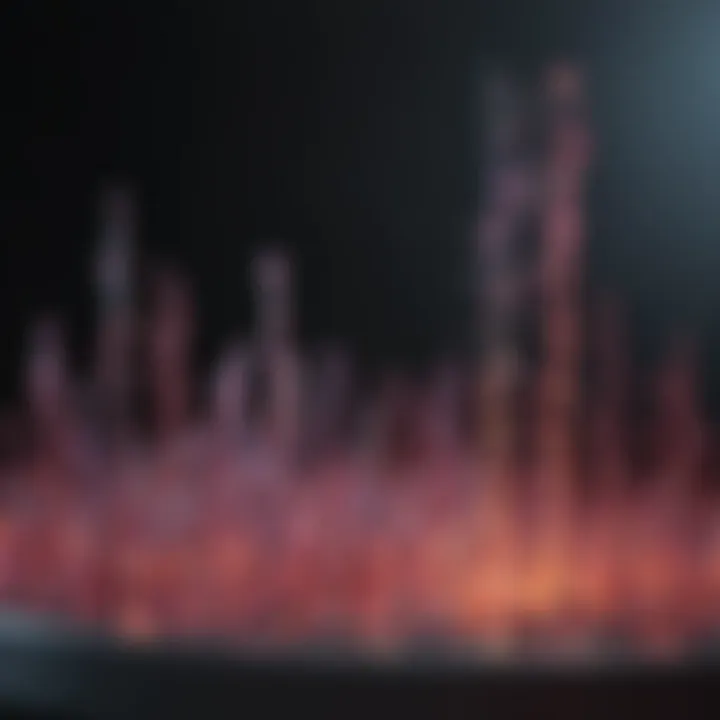
The DNA amplification in qPCR is a highly efficient process that allows for the exponential increase of a target DNA sequence. This is achieved using polymerase enzymes, which replicate the DNA. The cycle typically involves denaturation, annealing, and extension.
- Denaturation: The reaction mixture is heated to a high temperature, separating the double-stranded DNA into two single strands.
- Annealing: The temperature is lowered to enable primers to bind to the specific target sequences on the single-stranded DNA.
- Extension: The polymerase enzyme synthesizes new DNA strands from the primers, increasing the quantity of the target DNA sequence.
Through repeated cycles of these steps, the quantity of DNA amplifies exponentially. This aspect of qPCR is not only crucial for quantification but also for the specificity of the reactions, as properly designed primers facilitate selective amplification.
Fluorescence Detection Methods
In qPCR, monitoring the amplification process in real time relies on fluorescence detection methods. This is pivotal for determining quantitative values with precision. Two primary approaches are utilized: SYBR Green and TaqMan probes.
SYBR Green
SYBR Green is a commonly used fluorescent dye for qPCR. It binds to any double-stranded DNA, producing a fluorescent signal when exposed to light. This characteristic allows for the monitoring of DNA amplification as it occurs.
- Key Characteristic: SYBR Green's ability to emit fluorescence in the presence of double-stranded DNA makes it versatile.
- Benefits: Its simplicity and cost-effectiveness contribute to its popularity in laboratories.
- Unique Feature: One important feature is that SYBR Green can quantify all double-stranded DNA, which can lead to non-specific signals if primer-dimer formations occur.
- Disadvantages: This non-specific binding can introduce variability in quantification, necessitating careful experimental design.
TaqMan Probes
TaqMan probes present a more targeted approach to fluorescence detection. These are specific oligonucleotide probes designed to hybridize to a complementary sequence within the target DNA during amplification.
- Key Characteristic: TaqMan probes emit fluorescence only when they are cleaved by the DNA polymerase. This specific mechanism enhances the accuracy of quantification.
- Benefits: Their specificity minimizes background fluorescence, thus improving data reliability.
- Unique Feature: Each TaqMan probe is labeled with a fluorophore on one end and a quencher on the other. During PCR, the Taq polymerase cleaves the probe, separating the fluorophore from the quencher and leading to increased fluorescence, indicating amplification.
- Disadvantages: TaqMan probes can be more expensive and require more optimization compared to SYBR Green.
Understanding these fluorescence detection methods not only boosts the insight into qPCR mechanisms but also allows researchers to choose the right method for their specific needs.
Essential Components of a qPCR Setup
Understanding the essential components of a quantitative PCR (qPCR) setup is crucial for anyone aiming to execute this complex and powerful technique successfully. Each element plays a distinct role in the overall process. Proper selection and preparation of reagents, equipment, and conditions are imperative for obtaining accurate and reproducible results. In this section, we will explore these components to highlight their significance in the qPCR workflow.
Reagents Required
At the core of any qPCR experiment are the reagents used. Key components include:
- DNA or RNA template: This serves as the target for amplification. The quality and quantity of the nucleic acid can greatly influence the results, making initial preparation critical.
- Primers: Short sequences designed to bind specifically to target regions of the nucleic acid. Proper design is essential for specificity and efficiency in amplification.
- DNA polymerase: An enzyme responsible for synthesizing new DNA strands. Taq polymerase is commonly used due to its heat stability, allowing it to function effectively during the high temperatures of the PCR cycles.
- Nucleotides (dNTPs): These are the building blocks for new DNA synthesis. A balanced concentration of adenine, cytosine, guanine, and thymine is critical for optimal results.
- Buffer and salts: These maintain the pH and provide optimal conditions for the polymerase to function. The correct salt concentration can help alleviate issues related to enzyme activity.
- Fluorescent dyes or probes: Necessary for detecting the amplified DNA. The choice between SYBR Green or TaqMan probes will influence sensitivity and specificity in the quantification process.
The successful combination of these reagents forms the backbone of a qPCR experiment, affecting the efficiency and reliability of the output.
Equipment Considerations
The choice of equipment for a qPCR experiment is just as important as the reagents. Essential pieces of equipment include:
- Thermal cycler: This device is responsible for controlling the temperature cycles required for DNA denaturation, annealing, and extension. Real-time thermal cyclers allow for the monitoring of reactions as they progress, providing immediate feedback on amplification.
- ** fluorescence detection system:** Integrated with the thermal cycler, it captures the fluorescent signals emitted during the process. The accuracy of these readings is key to reliable data interpretation.
- Pipettes and consumables: High-quality pipettes are necessary for accurate solution measurement. Consumables such as PCR plates, tubes, and tips can influence contamination and outcome.
"Proper calibration and maintenance of equipment can vastly improve the reproducibility of qPCR results."
In summary, the essential components of a qPCR setup encompass a balanced interaction between reagents and equipment. Understanding the importance of each aspect will enable researchers to optimize their experiments, improving the reliability of their findings. By diligently selecting high-quality reagents and ensuring the accuracy of equipment, any uncertainties related to qPCR can be minimized.
Optimizing qPCR Conditions
Optimizing qPCR conditions is a crucial step in ensuring the accuracy and reproducibility of results in real-time quantitative PCR experiments. The effectiveness of qPCR relies heavily on specific parameters that can greatly influence the amplification of DNA. By fine-tuning the experimental conditions, researchers can maximize sensitivity and specificity, ultimately leading to reliable quantitation of target sequences.
Temperature Cycling Parameters
Temperature cycling parameters profoundly impact qPCR performance. Each cycle of the qPCR process involves three main steps: denaturation, annealing, and extension.
- Denaturation: Typically occurs at around 95°C. This step is vital for separating the double-stranded DNA template, allowing single strands to serve as templates for amplification.
- Annealing: Generally performed at a temperature between 50°C and 65°C, this step allows the primers to bind to their complementary sequences. The specific temperature for this step should be determined by the melting temperature (Tm) of the primers to ensure optimal binding.
- Extension: Usually occurs at a temperature of 72°C, where Taq polymerase extends the primers to synthesize the new DNA strand.
Each of these steps must be carefully set according to the specific reagents and targets used in the qPCR. Small adjustments can lead to significant differences in amplification efficiency. A common strategy is to run a gradient PCR to identify the optimal annealing temperature, enhancing the overall efficacy of the experiment.
Primer Design Strategies
Proper primer design plays a key role in the success of qPCR. Primers need to be specific to the target sequence for effective amplification. Considerations for primer design include:
- Length: Typically between 18-25 nucleotides.
- Melting Temperature: Should be similar for both primers to ensure they anneal together efficiently.
- Specificity: Primers should not bind to non-target sequences. Tools like Primer-BLAST can assist in evaluating primer specificity.
- GC Content: Maintaining a GC content of 40%-60% can lead to stable annealing.
Key Recommendation: Always include a negative control in your experiments to check for non-specific amplification or contamination.
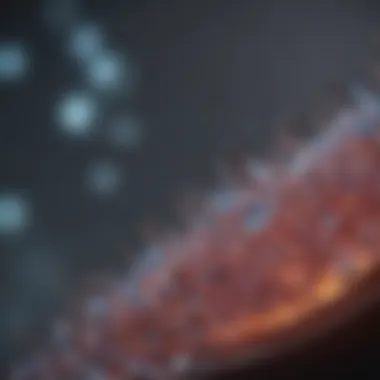
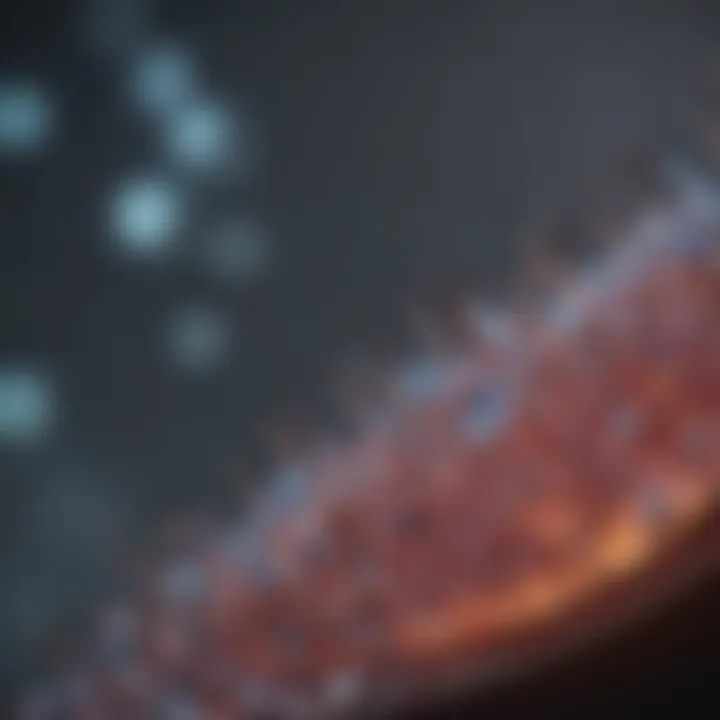
The design phase is not just about producing an amplifiable sequence but also ensuring that the design minimizes any possible extension from non-target sequences. Ultimately, optimizing these parameters leads to enhanced quantification performance and decreases variability, thereby providing more robust data for analysis.
Data Analysis and Interpretation
Data analysis and interpretation stand as crucial pillars in the realm of real-time quantitative PCR (qPCR). The precision and reliability of qPCR results hinge significantly on how data is processed and understood. The meticulous examination of data leads not only to quantitative measurements but also to solid conclusions on biological phenomena.
One of the primary elements of data analysis in qPCR is the threshold cycle (Ct) value. This metric serves as the fundamental parameter for measuring the amount of target DNA present in the sample. Accurate determination of the Ct value allows researchers to discriminate between positive and negative samples effectively, thus enhancing the credibility of the experimental results. Moreover, interpreting the Ct values involves understanding several factors that can affect results, including efficiency of amplification and variability in sample preparation.
Another vital component of data interpretation involves quantification methods, namely absolute and relative quantification. These methodologies provide different approaches to quantify the amount of target nucleic acid. Each method has its own distinct advantages and challenges, accommodating various research needs. A comprehensive understanding of these methods enables researchers to choose the best approach based on their experimental design and objectives.
In qPCR, it is also essential to consider the biological and technical replicates. Replicates ensure consistency and accuracy in data obtained from experiments. The presence of variability, whether from sample preparation or environmental conditions, must be acknowledged and addressed. This enhances the overall quality of data and ensures reliable conclusions can be drawn.
Ultimately, the process of data analysis and interpretation in qPCR is not a mere routine task. It incorporates a rich interplay of scientific expertise, informed decision-making, and advanced computational techniques. As the field of molecular biology evolves, the significance of rigorous data analysis will remain paramount, ensuring that the findings from qPCR studies are robust and impactful.
Threshold Cycle (Ct) Value
The threshold cycle, or Ct value, is pivotal in the analysis of qPCR data. The Ct value is defined as the PCR cycle number at which the fluorescence signal of the amplified DNA crosses a predetermined threshold level. This cycle number is inversely proportional to the initial amount of target nucleic acid in the sample. A lower Ct value indicates a higher initial quantity of target DNA, whereas a higher Ct value suggests a lower initial amount.
Analyzing the Ct values provides insights into the efficiency of the PCR reaction and the quality of the samples. Factors such as primer efficiency, reaction components, and sample integrity influence the accuracy of Ct measurements. Understanding these influences is critical for reliable interpretation.
"The Ct value serves as a direct measure of target DNA in qPCR, making its analysis a cornerstone of the technique."
Quantification Methods
Absolute Quantification
Absolute quantification is a methodology whereby the exact quantity of a target sequence is determined. This technique relies on a standard curve generated from known concentrations of the target DNA. The unique feature of absolute quantification is that it allows researchers to report the actual number of molecules present in the sample. This is particularly beneficial when precise quantification is necessary, such as in clinical diagnostics or viral load measurements.
The main advantage of absolute quantification lies in its ability to provide explicit numerical data, which can directly relate to biological significance. However, it requires careful calibration and validation of standards, which can introduce variability into the results. As such, absolute quantification is a popular choice among researchers who require accuracy in their measurements.
Relative Quantification
Relative quantification, in contrast, focuses on comparing the amount of target nucleic acid in different samples, using a reference or control sample as a baseline. This method calculates the relative changes in gene expression levels based on the comparison with reference genes or control samples. One key characteristic of relative quantification is its simplicity in design, making it less demanding in terms of reagent preparation.
This method is beneficial in studies focused on analyzing differential gene expression or cellular responses to stimuli. However, its reliance on reference genes means that suitable controls must be used to ensure validity of results. Relative quantification can provide meaningful insights but must be interpreted carefully to avoid misrepresentation of data.
Applications of Real-Time qPCR
The applications of real-time quantitative PCR (qPCR) span a range of fields, notably in clinical diagnostics, gene expression analysis, and pathogen detection. Understanding these applications highlights not just the technique’s versatility but also its critical role in advancing research and healthcare.
Clinical Diagnostics
In clinical settings, qPCR is pivotal for diagnosing various diseases, especially infectious diseases. The technique enables the quantification of specific DNA or RNA sequences, allowing for the detection of pathogens with high sensitivity and specificity. This is particularly crucial in cases where rapid diagnosis can impact patient outcomes.
For instance, qPCR is widely used to detect viral infections, including HIV and SARS-CoV-2. The ability to detect even minute quantities of viral genetic material helps in confirming infections in the early stages, often before symptoms appear. Moreover, the real-time aspect of the technique allows healthcare providers to obtain results quickly, facilitating timely treatment decisions.
Key points in clinical diagnostics using qPCR include:
- High Sensitivity: Ability to detect low levels of nucleic acids.
- Speed: Rapid turnaround time for results.
- Specificity: Precise identification of pathogen strains.
Gene Expression Analysis
Real-time qPCR is extensively used to study gene expression patterns. By measuring the levels of RNA in a sample, researchers can infer which genes are actively expressed and to what extent. This is especially useful in understanding cellular responses to various stimuli or conditions, such as during development or in response to treatment.
The data obtained from gene expression studies can provide insights into disease mechanisms, developmental biology, and responses to therapies. In cancer research, for example, qPCR helps identify upregulated or downregulated genes associated with specific tumor types, potentially leading to targeted therapies.
Considerations in gene expression analysis using qPCR are:
- Normalization: Use of reference genes to ensure accurate quantification.
- Replicates: Performing experiments in duplicates or triplicates to enhance reliability.
Pathogen Detection
In the realm of pathogen detection, qPCR is a game changer. It has redefined how microbiologists identify and quantify pathogens in environmental, food safety, and clinical laboratories. By harnessing the power of PCR amplification, researchers can detect bacterial and viral pathogens quickly and accurately.
For example, in food microbiology, qPCR can be utilized to monitor the presence of pathogens such as Salmonella or Listeria in food products. This level of testing supports food safety by enabling rapid response to contamination events. Environmental monitoring for pathogens in water supplies also exemplifies the technique's importance in public health.
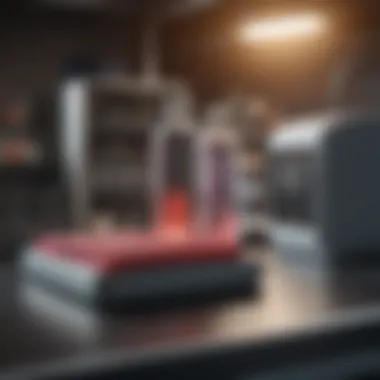
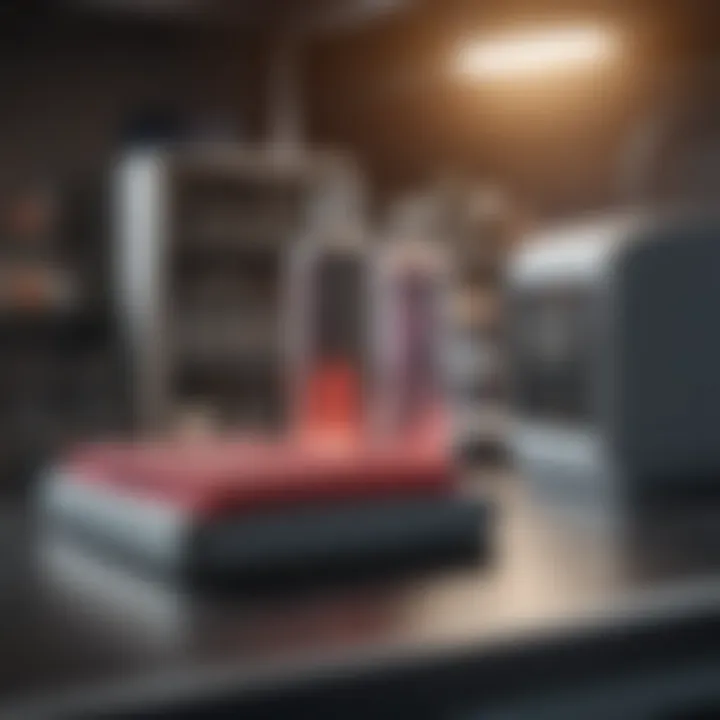
Pathogen detection highlights include:
- Scalability: qPCR can be adapted for high-throughput screening.
- Diversity: Capable of detecting a wide range of pathogens.
"Real-time qPCR not only revolutionizes diagnostics but is also integral in genomics and microbiology, providing deep insights into both health and disease."
The application of real-time quantitative PCR across these domains underscores its invaluable utility in modern science and medicine. As technology advances, its capacity to contribute to diagnostics, research, and public health will likely expand even further.
Challenges in Real-Time qPCR
Real-time quantitative PCR holds tremendous promise in molecular diagnostics and research. However, several challenges can impede its effectiveness. Recognizing these challenges is crucial for ensuring the reliability and accuracy of results. This section explores key challenges that practitioners may encounter when utilizing real-time qPCR, emphasizing specific factors that may compromise the system's performance.
PCR Inhibition Factors
PCR inhibition is one of the most significant obstacles affecting the accuracy of real-time qPCR. Various substances can interfere with the PCR reaction, leading to underestimation or complete failure in amplification. The sources of inhibition are diverse and can include:
- Biological Factors: Components like proteins, salts, or other cellular materials from samples can impede enzyme function.
- Contaminants: Organic compounds from sample processing, like phenol or ethanol, can also inhibit the reaction.
- Inhibitory Substances: Specific to certain samples, like environmental samples that may contain humic acids or other polyphenolic compounds, are notorious for causing inhibition.
Mitigating these effects is essential for improving the reliability of results. Techniques such as dilution of samples, the use of purified nucleic acids, or adding specific inhibitors can assist in overcoming these hurdles. Proper validation and optimization of the qPCR assay are critical to its success.
Variability in Results
Another challenge in real-time quantitative PCR is the variability in results. This variability can stem from multiple sources, impacting both reproducibility and reliability. Key factors include:
- Primer Efficiency: Different primers may perform differently, affecting amplification efficiency and leading to inconsistent Ct values.
- Sample Quality: The quality and integrity of the nucleic acid can vary widely depending on the source and method of extraction.
- Instrument Variability: Differences in qPCR machines can yield variability in data output. Calibration and regular maintenance of equipment are crucial.
To combat variability, it is vital to standardize protocols, use reference materials, and implement rigorous quality control measures. In addition, employing statistical analyses during data interpretation can aid in identifying outliers and ensuring the robustness of the findings.
Addressing these challenges is not only about enhancing the methodology but also about reinforcing the integrity of the results obtained from real-time qPCR. Collaborating within a community of researchers can aid in sharing best practices and troubleshooting common pitfalls.
Future Directions in qPCR Technology
The evolution of real-time quantitative PCR (qPCR) has been marked by rapid advancements that continue to push the boundaries of molecular diagnostics and research. The future of qPCR technology holds significant promise, informed by ongoing innovations in detection methods and integration with complementary technologies. This section will explore critical advancements and the implications of these developments for various scientific and clinical applications.
Advancements in Detection Techniques
As the demand for accurate and high-throughput analysis grows, laboratories are investing in new detection techniques that enhance sensitivity and specificity. One such advancement is the development of advanced fluorescence detection systems. These innovations include newer dyes with improved binding properties and signal-to-noise ratios. For instance, the optimization of SYBR Green and TaqMan probe systems has led to more precise quantification of nucleic acids, resulting in better data reliability.
Moreover, multiplex qPCR technology is gaining traction, allowing for the simultaneous quantification of multiple target sequences within a single reaction. This streamlining of workflows significantly reduces sample handling time and conserves reagents. Consequently, researchers can achieve more comprehensive data in less time, making qPCR an even more attractive tool for applications ranging from gene expression analysis to pathogen detection.
In addition, the rise of digital PCR represents a groundbreaking shift in how qPCR results are interpreted. Digital PCR enables absolute quantification of DNA without the need for standard curves. It provides greater precision in measuring low copy numbers, addressing some common limitations faced by traditional qPCR approaches.
Integration with Next-Generation Sequencing
The intersection of qPCR and next-generation sequencing (NGS) illustrates a significant direction for future advancements. By integrating these two powerful technologies, researchers can enhance genetic analyses in multiple ways. For one, qPCR can serve as a pre-screening method to identify specific sequences of interest before sequencing. This approach streamlines the analysis process, allowing for more targeted sequencing efforts which can be particularly beneficial in large-scale genomic studies.
Furthermore, the combination of qPCR and NGS enables comprehensive investigations into gene expression dynamics. Researchers can use qPCR to validate findings from NGS, ensuring the reliability of their data. This validation is crucial for experimental work in oncology, genetic disorders, and infectious disease studies.
In summary, the future of qPCR technology is bright, driven by advancements in detection techniques and synergistic integration with next-generation sequencing. These developments enhance the precision, efficiency, and applicability of qPCR in both research and clinical settings. The ongoing innovations will undoubtedly shape molecular biology practices, paving the way for breakthroughs in diagnostics and therapeutic strategies.
"The future of qPCR promises not just improved sensitivity and efficiency, but a fundamental shift in how we understand genetic materials and their expressions."
Thus, looming challenges will continue to spur developments in the qPCR landscape, encouraging researchers and clinicians alike to explore new horizons in molecular diagnostics.
End
The conclusion holds significant weight in any scientific discourse as it encapsulates essential insights derived from the preceding analysis. In the context of real-time quantitative polymerase chain reaction (qPCR), the conclusion serves to crystallize the vast array of information explored throughout the article. Understanding the nuances of qPCR is crucial, given its implications in various fields such as molecular diagnostics and research.
Summary of Key Points
- Real-time quantitative PCR is a vital technique that allows for the precise measurement of DNA amplification. This method is pivotal in clinical diagnostics, pathogen detection, and gene expression analysis.
- The understanding of amplification mechanisms and fluorescence detection methods, including SYBR Green and TaqMan probes, forms the foundation of successful qPCR experiments.
- Optimizing conditions like temperature and primer design is necessary for accurate and reproducible results.
- Data analysis, particularly through threshold cycle (Ct) values and quantification methods, is essential for interpreting results correctly.
- While repositories of knowledge continue to emerge, challenges like PCR inhibition and variability in results remain pertinent to practitioners.
- Future advancements in detection techniques and integration with next-generation sequencing highlight the evolving landscape of qPCR technology, paving new pathways for understanding genetic material.
Implications for Future Research
Looking ahead, the landscape of research surrounding real-time qPCR is ripe with possibilities. As technology continues to advance, the integration of high-throughput sequencing with qPCR can potentially unlock novel analytical approaches to genomics and metagenomics, enhancing our understanding of genetic variations and interactions. Moreover, exploring innovative methods to mitigate PCR inhibition can lead to more robust and reliable results in complex biological samples.
Overall, future research must not only refine existing methodologies but also explore novel applications that can further elevate qPCR's relevance in the evolving scientific arena.
"The continuing evolution of technology will dictate the trajectory of research in qPCR and its applications."
As this domain grows, it is imperative for researchers to stay abreast of developments. Emphasis should be placed on interdisciplinary approaches that merge computational techniques with molecular biology to foster new insights.