Exploring Quantum Information: Principles and Impact
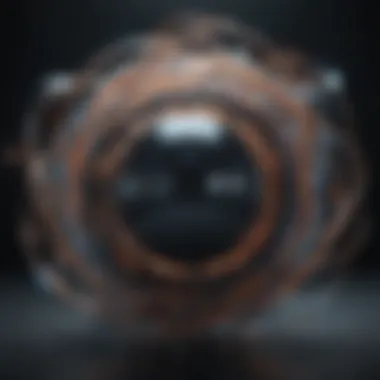
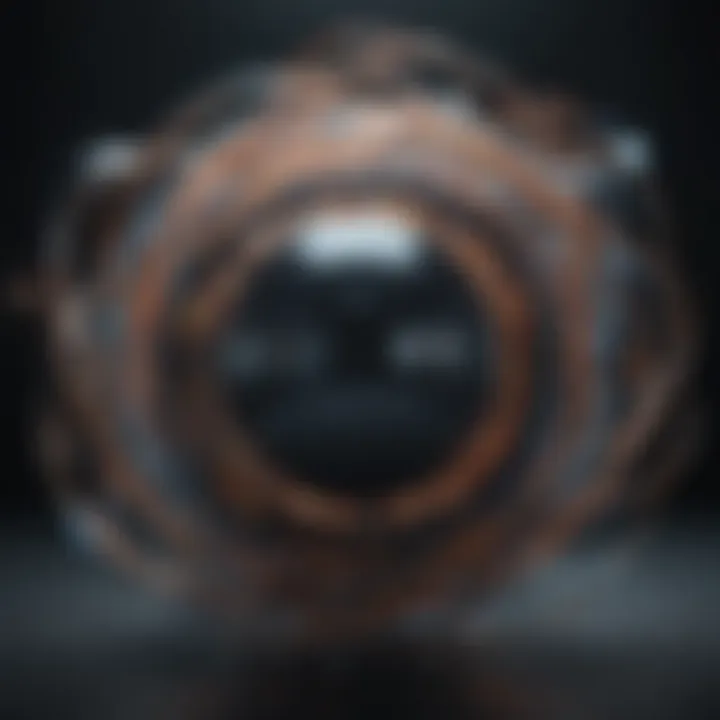
Intro
In the vast and complex tapestry of modern science, quantum information emerges as a vital thread connecting the microscopic world of quantum mechanics with the macroscopic realities of information theory. This interaction offers fascinating insights and possibilities, achieving a synthesis that can redefine how we process, store, and communicate information.
The exploration of quantum information is not just an academic exercise; it holds profound implications for technology, communication, and our understanding of the universe itself. By diving into this area, one discovers the intricate behaviors of quantum bits—commonly known as qubits—and how they fundamentally differ from their classical counterparts. The interplay of qubits and their entangled states push the boundaries of what we thought information could do.
As we sift through the layers of this topic, we will navigate a system of principles, techniques, and applications. We seek to illuminate several core dimensions in a manner that's both accessible and thought-provoking, allowing us to walk the tightrope between complexity and clarity. This discourse aims to not only inform but also inspire curiosity in a field that is burgeoning, and ripe with potential, challenging the status quo at every turn.
Let’s embark on this journey to uncover both the nuances and the revolutionary aspects of quantum information—an endeavor likely to capture the mind and spirit of students, researchers, educators, and professionals alike.
Prelude to Quantum Information
In today’s fast-paced technical landscape, delving into the idea of quantum information is crucial not just for scientists but for anyone keen on understanding the future of technology. Quantum information stands as a bridge between quantum mechanics and the theory of information, offering insights that can significantly transform classical concepts. As we explore this unique discipline, it's essential to recognize how quantum principles can swap traditional views on computing, security, and information processing altogether.
Understanding quantum information isn't just about grasping abstract concepts; it has tangible benefits. For example, industries ranging from cryptography to telecommunications are reshaping their practices around quantum theories. Knowledge in this area helps unpack the vast potential locked within the quantum realm. As a result, recognizing its importance allows enthusiasts and professionals alike to stay ahead.
Historical Context
The origins of quantum information can be traced back to the early 20th century, when physicists began unraveling the oddities of quantum mechanics. Notably, pioneers like Max Planck and Albert Einstein set the wheels in motion with their revolutionary ideas. These developments were initially confined to theoretical frameworks but eventually burgeoned into practical applications with the advent of computers in the late 20th century.
A significant turning point came in the 1980s when Charles Bennet and David Deutsch proposed new algorithms that highlighted how information could be encoded using quantum bits. Their work laid a strong foundation for further research, leading to today's thriving field.
"The bridge between quantum mechanics and information theory is paved by seminal insights and breakthroughs in the 80s."
Defining Quantum Information
Quantum information can be thought of as the study of information flow and processing at the quantum level. Unlike classical information, which is conveyed through binary bits (0s and 1s), quantum information utilizes quantum bits or qubits. Qubits can exist in multiple states simultaneously due to a phenomenon called superposition, allowing for a richer form of information representation and processing.
The defining features of quantum information revolve around several core principles:
- Superposition: This allows qubits to represent multiple states at once, unlike classical bits.
- Entanglement: A peculiar connection between qubits, where the state of one qubit can instantaneously influence another, regardless of distance.
- Quantum Measurement: In contrast to classical measurement methods, measuring a quantum state alters it, making the understanding of information transfer essential.
In a nutshell, quantum information not only challenges our classical perception of bits but also opens up a landscape of possibilities for computation, making it the bedrock of next-generation technologies.
Fundamental Principles of Quantum Mechanics
The principles of quantum mechanics are the cornerstone of quantum information theory. Understanding these principles is essential to grasp the complexities of how quantum bits operate and interact. At the core lies the notion that, unlike classical physics, where objects exist in a defined state, quantum entities can exist in multiple states simultaneously. This strange behavior forms the foundation of various phenomena, including superposition and entanglement. The importance of these principles cannot be overstated as they underpin not only quantum computing but also quantum communication and cryptography, making them pivotal for future technological advancements.
Quantum States and Superposition
In quantum mechanics, a quantum state represents the condition of a particle or system, described mathematically by a wave function. A fascinating aspect of quantum states is superposition, suggesting that a quantum system can exist in various states at once until measured. This principle is often illustrated using the famous thought experiment of Schrödinger's cat, where the cat in the box is both alive and dead until someone opens the box to observe it.
Superposition allows qubits to perform many calculations concurrently. While a classical bit can be either 0 or 1, a qubit can be both 0 and 1, enabling a computational edge that classical systems simply can't match. The ability to exist in multiple states gives rise to parallelism in computation, immensely enhancing the efficiency of quantum algorithms.
"Superposition is the key to the 'quantum advantage', enabling superior processing power that shapes the future of computing."
Quantum Entanglement
The second fundamental principle, quantum entanglement, describes a scenario where two or more quantum particles become interconnected in such a way that the state of one particle instantly influences the state of the other, regardless of the distance separating them. This bizarre phenomenon challenges classical intuitions about locality and causality, leading Einstein to call it "spooky action at a distance."
Entanglement is crucial for various quantum information processes. By exploiting entangled states, quantum communication systems can perform tasks like secure information transfer through quantum key distribution, where any attempt to intercept the key would alter the state of the entangled particles, alerting the communicating parties. As the field develops, exploring practical applications of entanglement becomes increasingly important.
Measurement and Observation
Measurement in quantum mechanics differs drastically from classical interpretations. The act of measurement forces a quantum system to 'choose' one of its possible states. This is known as the collapse of the wave function. Before measurement, the system's properties aren't just unknown—they genuinely don't exist in a defined state. This phenomenon leads to various interpretations of quantum mechanics, such as the Copenhagen interpretation, which claims that physical systems do not have definite properties other than the ones that can be observed.
Upon measurement, various factors can influence outcomes, elucidating a level of unpredictability inherent in quantum mechanics that classical mechanics does not account for. This realm of uncertainty influences not only theoretical discussions but also practical implementation in quantum information technologies, where outcomes can vary based on the observer's interaction with the system.
Quantum Bits and Their Role
In the landscape of quantum information, quantum bits, or qubits, play a pivotal role that offers a fresh perspective on data storage and manipulation. Unlike classical bits that exist as either 0 or 1, qubits harness the peculiarities of quantum mechanics to represent both states simultaneously through a phenomenon known as superposition. This fundamental characteristic sets the stage for a multitude of advantages in information processing, making qubits essential to the development of quantum technologies.
The ability to exist in superposition means that a single qubit can be in multiple states at once. This opens the door to parallel processing capabilities far beyond anything classical systems could achieve. In practical terms, this means that many calculations can occur at the same time, leading to potentially exponential speedups for specific types of problems. For instance, tasks such as factoring large numbers or searching unsorted databases can be performed much more efficiently with quantum algorithms built upon qubits.
Another critical aspect is quantum entanglement. When qubits become entangled, the state of one qubit is directly related to the state of another, no matter the distance apart. This can facilitate communication protocols that are inherently secure, as any attempt to eavesdrop on the entangled qubits would disturb their state and thus alert the parties involved. The intricacies of qubits bring new dimensions to cybersecurity, which is invaluable in our increasingly digital world.
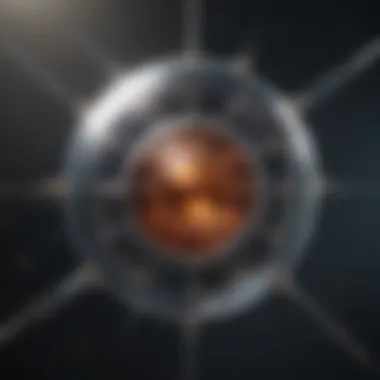
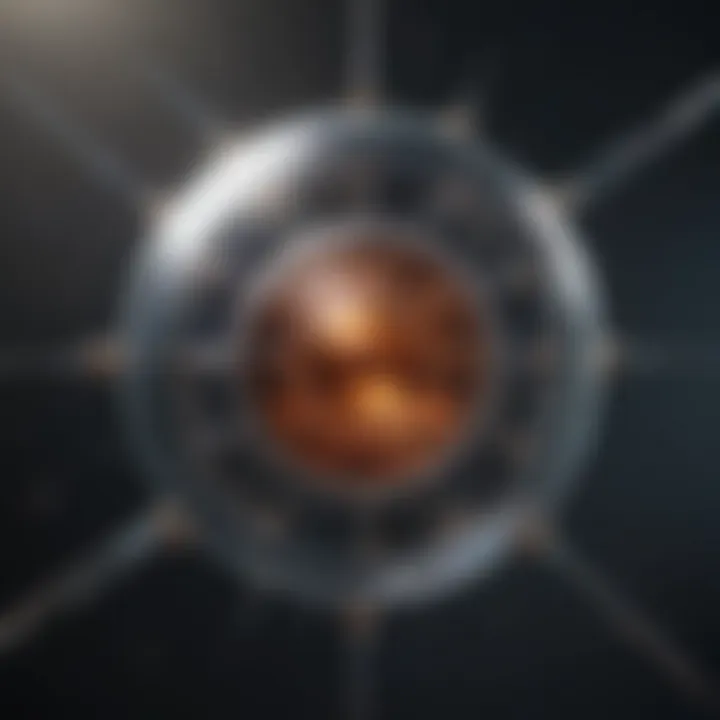
Moreover, to make use of qubits effectively, they need to be engineered with precision, considering factors such as coherence time and error correction. This engineering is crucial for building practical quantum computers, ensuring that they operate efficiently despite the delicate nature of quantum states.
Adopting quantum bits also poses unique challenges, particularly surrounding their scalability and physical implementation. Researchers are exploring various technologies for realizing qubits, such as superconducting circuits, trapped ions, and topological qubits. Each approach brings its strengths and weaknesses, making the race for the most effective qubit implementation a lively and fierce competition.
The versatility and promise of qubits point to a future where quantum computing could redefine the limits of what is computationally feasible, merging theoretical physics with practical applications.
In summary, the role of quantum bits within this dialogue is profound. They not only bridge the understanding of quantum mechanics and information theory but also shape the core of emerging quantum technologies that have the potential to revolutionize various sectors like finance, medicine, and artificial intelligence.
Understanding Qubits
A qubit fundamentally differs from its classical counterpart in several ways that enhance the functionality of quantum systems. While a classical bit can only hold a value of 0 or 1, a qubit can occupy a state anywhere along the unit circle in a two-dimensional complex vector space. This property is essential because it allows qubits to represent information more densely than classical bits.
To visualize this, imagine a spinning coin. While the coin spins, it's not in a defined state of heads or tails; instead, it's literally in a state of both until it lands. This is similar to how a qubit can be in a combination of states – a crucial advantage for quantum computing. When measured, however, a qubit collapses to one of its possible states, making the measurement process both fascinating and informative.
Further complexity comes from the fact that the mathematical representation of qubits utilizes complex numbers. Each qubit can be represented as a vector in a two-dimensional space, described with the notation |0⟩ and |1⟩, forming the basis of quantum computing.
Comparison with Classical Bits
When comparing qubits to classical bits, distinct differences emerge that highlight the technological advancements offered by quantum mechanics:
- State Representation: Classical bits are binary, meaning each can only be in one of two states. In contrast, qubits can represent multiple states concurrently due to superposition.
- Processing Power: As mentioned earlier, qubits enable parallel processing, allowing quantum computers to perform many calculations at once. Classical computers, reliant on sequential processing, can take significantly longer to solve complex problems.
- Entanglement: This is a feature unique to quantum systems. Entangled qubits can be correlated with each other in a way that classical bits cannot, enhancing communication and security in quantum networks.
- Error Correction: While classical error correction can handle various fault conditions, quantum error correction presents unique challenges because measuring a qubit often alters its state. The development of quantum error correction codes is ongoing, with researchers striving to address this obstacle.
Information Theory Meets Quantum Mechanics
The intersection of Information Theory and Quantum Mechanics forms a fascinating domain with profound implications. As we probe deeper into the properties of quantum information, understanding this interplay becomes crucial. Information Theory provides the fundamental framework for quantifying information, while Quantum Mechanics introduces unique behaviors and phenomena—redefining how we think about data. In essence, this relationship serves as a bridge, facilitating advancements in various fields such as cryptography, computation, and communication.
The significance of marrying these two fields is multi-dimensional. On one hand, Information Theory equips us with tools to analyze the capacity of quantum channels. On the other, Quantum Mechanics shows us that the classical views of information do not always hold true when we enter the quantum regime. With these insights, researchers are better situated to explore applications in quantum computing and secure communications, not to mention the philosophical inquiries raised by the nature of quantum information itself.
Overview of Information Theory
Information Theory, developed chiefly by Claude Shannon in the mid-20th century, revolves around the quantification of information. Its foundational concepts include entropy—the measure of uncertainty in a set of possible outcomes—and data compression, where the goal is to reduce the size of data while maintaining its integrity. In classical contexts, the emphasis tends to be on bits, the simplest unit of information.
However, Quantum Information Theory extends these classical principles into a realm altered by quantum properties.
- Entropy in Quantum Systems: Just as in classical systems, entropy measures uncertainty, but in a quantum context, it takes on new forms like von Neumann entropy. This accounts for the quantum state of a system.
- Quantum Error Correction: Classical error correction techniques need rethinking when we're in the quantum domain. Techniques have emerged that exploit the peculiarities of qubits to protect against decoherence and other noise factors.
- Capacity of Quantum Channels: The concept of channel capacity becomes more intricate. Quantum channels can transmit information in ways that classical channels cannot, which allows for higher data rates and security measures.
When approaching Information Theory and its extension into the quantum sphere, one cannot overlook its philosophical implications either. Consider how traditional notions of information as something distinct and separable evolve under quantum constraints, reflecting a world where the observer and the observed are intertwined.
Quantum Channels and Communication
When we grapple with Quantum Channels, we’re discussing the pathways through which quantum information can be transmitted. The behavior of these channels is influenced heavily by the superposition and entanglement properties unique to quantum particles.
Quantum communication leverages these properties to enhance reliability and security.
Some Key Elements of Quantum Channels:
- Superdense Coding: This technique allows the transmission of more information than would typically be possible within the constraints of classical channels. By encoding two bits of classical information into a single qubit, it harnesses quantum entanglement to achieve a higher rate of information transfer.
- Quantum Teleportation: This phenomenon allows the transfer of quantum states from one location to another without moving the physical particle itself. It exemplifies how quantum channels can redefine our understanding of communication. The actual physical transfer of qubits does not occur; rather, the state is reconstructed at the destination.
- Secure Communication: Quantum key distribution, particularly protocols like BB84, illustrate how quantum mechanics can create a security layer impervious to eavesdropping. Any attempt at observing quantum communications inevitably alters the states being measured, signaling potential breaches in security.
"In quantum communication, the act of observation changes what is observed—redefining trust and verification in novel ways."
These channels have opened doors to explore advanced communication methods, but they also bring challenges. Research continues, highlighting the necessity of designing quantum networks that can address issues such as signal degradation and loss due to environmental interference.
In summation, understanding the convergence of Information Theory and Quantum Mechanics reveals a richer, more intricate picture of information as it stands at the cutting edge of technology. Future advancements in this field hinge on deepening our grasp of how quantum phenomena can reshape traditional information concepts, promising a landscape of unprecedented possibilities.
Quantum Computing: Revolutionizing Technology
The world is witnessing a seismic shift in computing with the advent of quantum technology. Quantum computing stands at the intersection of quantum physics and information theory, offering unprecedented approaches to problems that are otherwise intractable for classical computers. The importance of this field cannot be understated, as it stretches beyond mere theoretical exploration; it promises to revolutionize industries ranging from pharmaceuticals to cryptography.
Principles of Quantum Computing
At the core of quantum computing, we find unique principles that diverge sharply from classical computing. Classical bits, the building blocks of traditional computing, exist in a state of 0 or 1. Qubits, on the other hand, harness the power of quantum mechanics to exist in a state of superposition. This means a qubit can be in multiple states at once, allowing quantum computers to perform vast numbers of calculations simultaneously.
Another remarkable feature of quantum computing is entanglement. When qubits become entangled, the state of one qubit is dependent on the state of another, no matter how far apart they are. This intricate relationship can lead to incredibly faster processing speeds and enhanced information transfer. Moreover, quantum gates are used to manipulate these qubits in ways that classical gates cannot, providing additional layers of computational capability.
Quantum Algorithms
The landscape of algorithm development represents a significant frontier in quantum computing. Quantum algorithms are designed to leverage qubits effectively, enabling tasks at speeds impossible for classical methods. One of the most notable quantum algorithms is Shor's Algorithm, which can factor large integers exponentially faster than the best-known classical algorithms. This poses a potential threat to traditional encryption methods, which rely on the difficulty of factoring numbers.
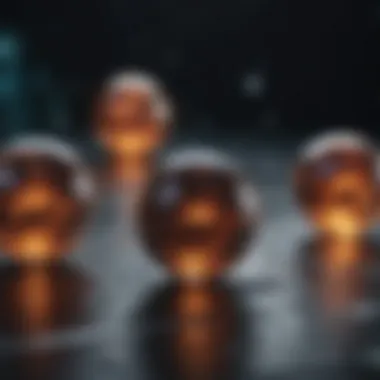
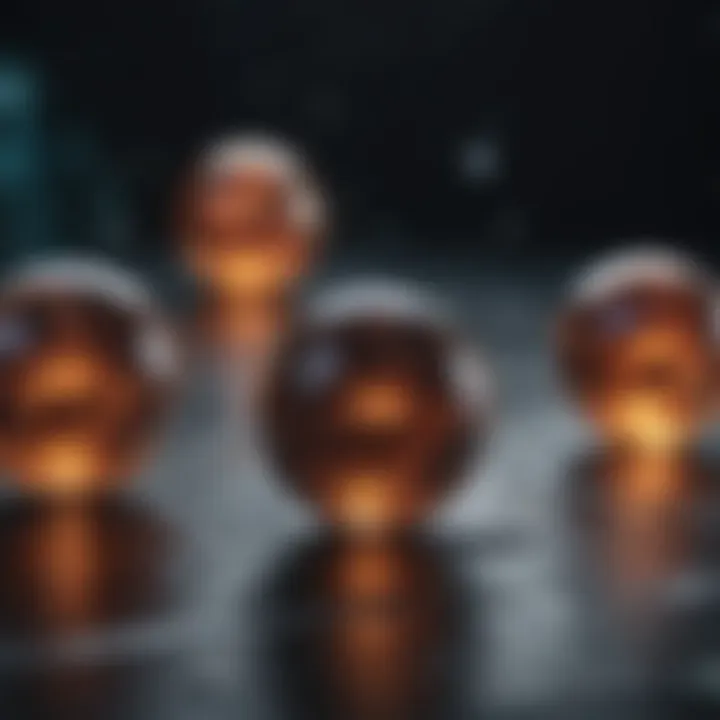
Similarly, Grover's Algorithm offers a groundbreaking approach to searching unsorted databases in quadratic time, a marked improvement over classical algorithms. These developments illustrate that while quantum computing is still in its nascent stages, the potential applications in optimization, simulation, and cryptography could reshape entire fields.
Advantages over Classical Computing
Quantum computing is not just a mere enhancement of classical computing; it fundamentally changes the way we think about processing information. Here’s why it stands out:
- Speed: Quantum computers can process information at extraordinary speeds due to superposition and parallelism. This shift means that tasks like complex simulations will be resolved much faster.
- Capacity to Handle Complexity: Quantum systems can naturally model complex phenomena in physics, chemistry, and biology where classical systems struggle. This feature enables breakthroughs in drug discovery and material science.
- Enhanced Security: In terms of cyber security, quantum computing can lead to secure communication through quantum key distribution methods, reducing vulnerabilities that traditional systems face.
The transition to quantum computing is not just an evolution; it's a revolution that pushes the boundaries of scientific capabilities into uncharted territory.
As the field develops, the integration of quantum principles into existing technologies may yield a plethora of innovative solutions, enhancing efficiency and opening new avenues for research.
By exploring quantum computing, we unlock possibilities that could redefine the way societies function and process information. As barriers are torn down, a new age of technology awaits us.
Applications of Quantum Information
The realm of quantum information is not merely confined to theoretical exploration; it esteems its significance through practical applications that revolutionize diverse fields. From secure communications to computations that eclipse our classical capabilities, these applications serve an indispensable role in understanding how quantum mechanics reshapes the landscape of technology. This section will navigate through key applications that elucidate the profound impact of quantum information on modern society.
Cryptography
In an age characterized by digital information exchange, cryptography stands as a cornerstone for securing data. Quantum cryptography, notably the use of quantum key distribution, utilizes the principles of quantum mechanics to create communication channels that are fundamentally secure. For instance, consider a scenario where two parties share a secret key via quantum entanglement. Any attempt by an outsider to intercept the key would disturb the quantum state, alerting the involved parties to the breach. According to a recent study, quantum cryptography is expected to enhance the security of transactions in sectors ranging from finance to national security.
Some essential points regarding quantum cryptography include:
- Unconditional Security: Unlike classical cryptographic methods that rely on mathematical complexity, quantum cryptography's security depends on the laws of physics.
- Real-world Implementations: Organizations like ID Quantique are already pioneering quantum cryptography systems that are being deployed in various industries.
Quantum Teleportation and Networking
Quantum teleportation captures the imagination with its almost science-fictional possibilities. It describes a process where the state of a quantum particle is transmitted from one location to another, without the physical transfer of the particle itself. Despite its daunting name, teleportation in this context does not imply a sci-fi like instant travel but involves the clever use of quantum entanglement and classical communication channels.
Practical implications of quantum teleportation are profound. In a network scenario, if two quantum computers are entangled, they can share quantum states instantaneously. This could allow data transmission speeds that far exceed current classical systems.
Key aspects include:
- Potential Applications: Teleportation could facilitate quantum networks that enhance the capabilities of technologies such as the Internet of Things (IoT).
- Challenges: The integration of quantum teleportation into existing infrastructures poses challenges, particularly in maintaining entanglement over long distances.
Quantum Simulations
Quantum simulations offer an opportunity to model complex quantum systems that classical computers struggle to handle effectively. With their unique capabilities, quantum simulators can mimic the behavior of molecules, materials, and complex physical systems, thereby enabling breakthroughs in fields such as material science and pharmaceutical research.
Consider the potential of quantum simulations in drug discovery. Traditional methods to test drug interactions are time-consuming and expensive. Quantum computers can simulate molecular interactions at an unprecedented level of detail, significantly speeding up the drug development process. Here are a few advantages of quantum simulations:
- Enhanced Accuracy: They provide results that are closer to real-world conditions, thereby reducing the experimental overhead.
- Broader Applications: This method may lead to discoveries in areas such as superconductivity and quantum chemistry, which have wide-ranging implications.
By leveraging quantum arguments, researchers are not only aiming to solve today’s pressing issues but also to discover phenomena that were previously invisible, poised to unveil a new era of scientific inquiry.
Challenges and Limitations
Quantum information advances our understanding dramatically, yet it does not come without twinges of complication. It's vital to explore the challenges and limitations we face as researchers plunge into the quantum realm. Addressing these obstacles is essential, as it informs future innovations and safeguards application robustness in a rapidly evolving technological landscape.
Technical Limitations
The technical limitations surrounding quantum information primarily stem from the very nature of quantum mechanics itself. Consider quantum bits, or qubits; they exhibit quirky properties like superposition and entanglement, which make them powerful but also demanding in terms of maintenance.
- Quantum Decoherence: This is one of the main technical hindrances. Qubits are extremely sensitive to their environment. Even slight interactions with external factors can cause decoherence, where they lose their quantum state, rendering calculations unreliable. The need for extreme temperature conditions and isolation from outside noise poses significant challenges.
- Error Rates: The manipulation of qubits must be precise. Due to their susceptibility to noise, error rates can be higher than in classical systems, necessitating complex error correction strategies. This not only demands additional resources but also complicates the logical frameworks needed for quantum computation.
Addressing these technical limitations demands a multidisciplinary approach, requiring input from physics, engineering, and computer science to develop solutions that ensure stability and maintain quantum integrity.
Scalability Issues
Scalability is a pressing concern in quantum information technology. As researchers aim to build larger quantum systems capable of performing complex computations, several issues arise that need tackling.
- Physical Implementation: The current methods to create and manage qubits are varied, ranging from trapped ions to superconducting circuits. Each has unique scaling characteristics, and reconciling these differing technologies into a unified framework is no small feat.
- Resource Demands: Scaling up quantum systems often means increased resource consumption. Cooling systems, shielding from electromagnetic interference, and refinement processes can elevate operational costs and design complexities. As quantum networks expand, so do the requirements for optimal functioning.
- Interconnectivity: For a quantum network to be truly practical, incorporating numerous qubits that can communicate efficiently is an uphill battle. Quantum entanglement is not something you can just plug and play; it requires meticulous setup and maintenance, which can hinder scalable integration.
As the race for quantum supremacy is underway, addressing these scalability issues becomes paramount not just for theoretical advancement but also to make the technology viable for real-world applications. Without overcoming these barriers, it’s like trying to build a skyscraper on sand - the foundations must be solid.
"The challenges in quantum information are the same type of beasts found in any pioneering field; they require ingenuity, collaboration, and an unyielding commitment to finding solutions that open new doors for technology."
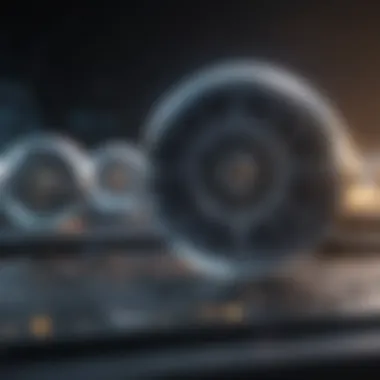
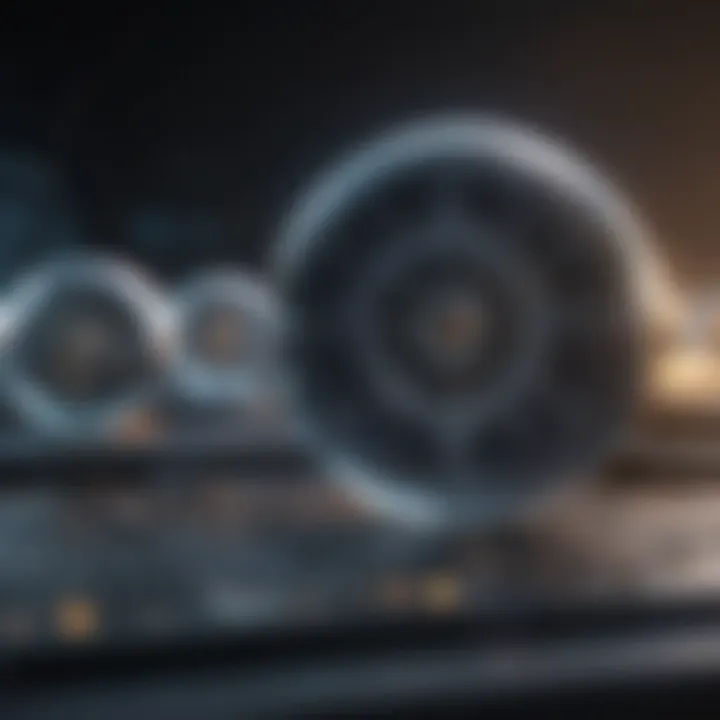
The road ahead is certainly not paved in gold, yet it teems with promise, and overcoming these challenges will undoubtedly unlock the true potential of quantum information in the broader technological landscape.
Future Directions in Quantum Information
The trajectory of quantum information science is set to leave its mark not only in technology but in various aspects of everyday life. The rapid advancements in this field could influence the realms of security, computing, and even social structures. Understanding these future directions gives insights into how society may evolve in response to quantum breakthroughs. It's more than just a trend; it’s a transformative journey with potential that seems limitless.
Research Innovations
As researchers tackle the complexities of quantum systems, we stand at the threshold of significant breakthroughs. Innovations are bubbling up from laboratories and academic institutions around the world, each promising to expand our grasp of quantum mechanics and information theory. Here are some key areas of active research:
- Quantum Algorithms: Researchers are refining algorithms that leverage quantum properties. This includes developments in Shor's algorithm for factoring large numbers and Grover's algorithm for search problems. Both could dramatically change the landscape of cryptography and data processing.
- Quantum Networks: Efforts to establish quantum networks, which use entangled particles to securely share information, are gaining ground. Initiatives like the Quantum Internet Alliance are forging a path towards practical quantum communication systems.
- Quantum Error Correction: This area aims to address the fragility of qubits. Innovations in error-correcting codes are essential for building stable quantum computers that could perform complex calculations without succumbing to decoherence issues.
These pioneering research areas are not isolated. They interlink, creating a web of knowledge that supports each other. For instance, advancements in quantum algorithms often lead to new insights in practical quantum network implementation.
Integrating Quantum Information in Society
As quantum technology becomes more ubiquitous, its integration into society poses several considerations. Here’s where it gets particularly interesting:
- Education and Awareness: With quantum information becoming more prominent, there's a pressing need for education. Understanding these concepts should not be limited to specialists. Programs that demystify quantum principles for the general public are crucial. Initiatives could include workshops or MOOCs that explore the basics of quantum computing alongside their implications.
- Policy and Regulation: Governments will need to establish clear policies regulating the use of quantum technologies, particularly in areas like cryptography and telecommunications. Legislative frameworks should address both the potential for misuse and the implications on privacy.
- Industry Adaptation: Companies across various sectors must adapt to the quantum revolution. Industries such as finance, healthcare, and telecommunication stand to benefit immensely from quantum computing. A proactive approach in these sectors could lead to groundbreaking applications and services.
The fusion of quantum technology into the societal fabric will not be without challenges. The balance between fostering innovation and regulating its applications is delicate. Understanding this integration is essential for shaping a future that leverages quantum advancements while mitigating risks.
"The next steps in quantum information could very well dictate the terms of our technological interactions for decades to come."
To sum it up, future directions in quantum information reveal a landscape rich with possibilities. Innovations in research will shape the next generation of technology, while thoughtful integration into society ensures that these advancements serve the greater good.
Ethics and Implications of Quantum Technology
When we think about the future shaped by quantum technology, one can’t turn a blind eye to the ethical implications that arise. The leaps made in quantum computing and information processing are not just altering how we process data but also reshaping the foundational ideas of privacy, security, and the very nature of trust in information systems. As these technologies become more prevalent, discerning their ethical dimensions is not merely prudent; it is essential.
With quantum technology holding the potential to disrupt existing structures of power, we must grapple with the implications of its applications. There are several specific elements worth considering, such as:
- Accountability: Who bears responsibility when quantum systems go awry?
- Access and Inequality: Will advanced quantum computing deepen the divide between those who can afford this technology and those who cannot?
- Manipulation of Information: Can quantum capabilities lead to unforeseen exploitation of data?
These nuances lay the groundwork for understanding how quantum technology fits into a broader societal framework – one that ensures ethical progress accompanies technical advancement.
Ethical Considerations
Delving deeper, when we speak of ethical considerations in quantum technology, we are addressing a range of dilemmas. Quantum algorithms, capable of solving problems inaccessible to classical computers, raise questions of fairness as they can yield significant advantages in various sectors.
One key aspect is transparency. Many quantum algorithms operate in ways that are not fully understood even by the experts. As a result, there's a clear need for clarity in how these systems function and make decisions. This opacity can cloud accountability; if things go sideways, understanding who's liable becomes complicated.
Moreover, issues like informed consent come to the forefront, especially if quantum systems interact with user data. Are individuals aware of how their information is being processed, and are they truly giving consent? Questions like these form the crux of a responsible approach to quantum technology implementation.
Impact on Security and Privacy
Once we glaze over the ethical considerations, we just can’t ignore the colossal impact quantum technology has on security and privacy. The field is a double-edged sword, presenting both challenges and opportunities. On one hand, quantum computing is set to render traditional encryption methods virtually obsolete.
"As quantum computers advance, encryption techniques in use today may become mere relics of a bygone era, exposing personal and sensitive information like never before."
Consider the realm of encryption. Algorithms that safeguard data today—like RSA and AES—are based on complex mathematical problems that classical computers struggle to solve. Quantum computers, however, would crack these codes with ease, thereby jeopardizing the security of sensitive communications.
Yet it isn’t entirely doom and gloom. Quantum Key Distribution, for example, offers a framework for secure communication that could thwart eavesdroppers effectively. Still, the transition to such methods necessitates caution and careful oversight.
In sum, navigating the ethical landscape of quantum technology compels us to expand our conversations around privacy and security. Society must engage in these dialogues, cultivating an environment where technical prowess does not supersede human rights. Only by acknowledging and actively addressing these concerns can we harness the power of quantum technology responsibly.
End
The conclusion of our discourse on quantum information serves as a crucial element in solidifying the framework established throughout this article. It encapsulates not just the diverse topics summarized but also underscores the profound significance of understanding quantum mechanisms in an increasingly digital world. Quantum information merges physics and information theory, paving the way for revolutionary applications in computing, cryptography, and beyond.
Summation of Key Points
In distilling the central themes discussed, we begin with quantum bits, or qubits, which embody the essence of quantum computing, distinguished from classical bits by their ability to exist in multiple states simultaneously thanks to superposition. We also highlighted quantum entanglement, a phenomenon where particles become interconnected, allowing information to be shared in ways that challenge traditional notions of communication.
Moreover, we engaged with the implications of quantum mechanics on information theory, revealing how the two fields converge to reshape our understanding of security through quantum cryptography. This was essential for recognizing the ethical dimensions and potential risks associated with applying quantum technologies in real-world scenarios.
Finally, the challenges of scalability and technical limitations we discussed are equally vital, as these hurdles play a significant role in the pace of future development. Recognizing these issues allows researchers and practitioners to strategize effectively, ensuring that advances in quantum information are not just theoretical but also practicable.
The Path Ahead
Looking forward, the future of quantum information stands as a promising frontier. Continuous research innovations are pivotal in enhancing our collective grasp on its intricacies, suggesting that as we delve deeper, avenues for integration into everyday use will emerge. Educational initiatives that demystify quantum computing principles and technologies will be essential for nurturing a new generation of researchers and engineers.
Moreover, the collaboration across disciplines—merging insights from physics, computer science, and ethical studies—will enrich the development of quantum technologies. As we tread into this uncharted territory, it is imperative to remain vigilant of the ethical implications of quantum computing. Not only must we address the technical facets but also reflect on the societal impacts, which could echo through time.
"The blend of quantum mechanics and information theory does not merely promise to change technology; it reshapes our very conceptions of information, reality, and progress."
In summary, the journey through quantum information provides a multifaceted perspective that highlights the current status and future potential of this revolutionary field. As the old adage goes, "The sky is the limit," but in quantum information, the limits are yet to be defined.