Exploring Crystal Transparency: Key Insights
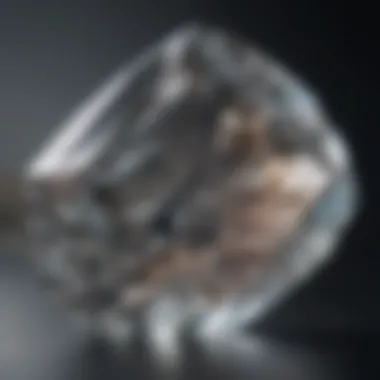
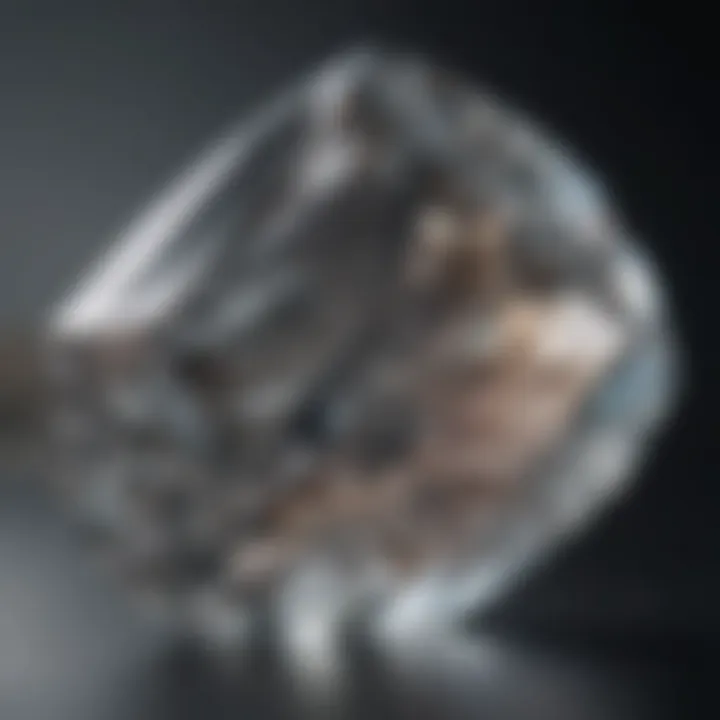
Intro
Crystals are fascinating structures that capture the attention of scientists and enthusiasts alike. Their ability to transmit light, known as transparency, provides significant insights into their optical properties. This article is designed to offer an in-depth analysis of how various crystal types allow light to pass through while exploring their unique structures. Understanding these mechanisms can push boundaries in materials science and optics, taking us deeper into practical applications that define our technological landscape.
Research Overview
Summary of Key Findings
In this section, we present the essence of transparency in crystals. Research indicates that the arrangement of atoms within a crystal lattice plays a critical role in how light interacts with it. Key findings include:
- Crystal Structure: Different structures lead to distinct light transmission efficiencies.
- Types of Crystals: Common examples such as quartz, diamond, and calcite exhibit unique transparency levels, which can be related to their atomic arrangements.
- Optical Properties: Refraction, reflection, and absorption characteristics are deeply tied to a crystal's transparency, influencing many practical applications.
Relevance to Current Scientific Discussions
The study of crystal transparency is not just an academic pursuit but a vital inquiry into the very fabric of materials science. Crystal behaviors have implications across various domains, from the development of advanced optical devices to innovative materials in technology. Recent discussions in scientific literature emphasize:
- The impact of crystal clarity on laser technology and telecommunications.
- The role of transparency in the design of precision optical instruments.
- Environmental influences that can alter the transparency of natural and synthetic crystals, such as temperature and impurities.
Methodology
Research Design and Approach
This analysis employs a comprehensive review of existing literature on crystal transparency. The approach includes theoretical frameworks and experimental findings to create a cohesive understanding of the subject. The design is systematic, ensuring all relevant information is incorporated.
Data Collection and Analysis Techniques
Data is gathered from reputable sources, including scientific journals and databases, enhancing the reliability of the findings. Analytical techniques used include:
- Comparative analyses of different crystal types.
- Review of experimental results relating to light transmission.
- Synthesis of case studies that illustrate real-world applications.
"Understanding crystal transparency is pivotal in advancing both theoretical concepts and practical applications in optics."
Finale
This exploration into crystal transparency reveals its complexity and significance. Through this analysis, one can appreciate the intricate relationship between structure and optical properties, paving the way for innovations in various fields. Understanding these characteristics will potentially lead to breakthroughs that extend our knowledge of material science and technology.
Foreword to Crystal Transparency
The exploration of crystal transparency plays a critical role in understanding not only the physical properties of crystals but also their myriad applications in science and industry. Transparency in crystals is defined by their ability to transmit light without significant scattering or absorption. This property is essential for various fields, including optics, electronics, and materials science. A clear comprehension of crystal transparency helps to leverage these materials’ unique optical characteristics for practical use, from the production of optical devices to advancements in technology and industry.
Defining Crystal Transparency
Crystal transparency is a measure of how clearly light can pass through a crystal without significant obstruction. It involves the interplay between light and the atomic structure of the crystal. When light strikes a crystal, it can be transmitted, absorbed, or reflected depending on the crystal’s composition and structure. The degree of transparency is determined by several factors, including the crystal’s lattice structure, the type of atoms involved, and any defects or impurities present in the crystal. While some crystals exhibit high levels of transparency, allowing for clear passage of light, others may partially or entirely block light, depending on their characteristics.
Importance in Science and Industry
The significance of crystal transparency extends beyond mere aesthetics. In scientific research, transparent crystals are pivotal in the study of light interactions and the development of optical devices. Materials such as quartz or sapphire serve as substrates for lasers and optical fibers, where transparency is essential for efficient light transmission. In the field of electronics, transparent conductive oxides like Indium Tin Oxide find applications in touch screens and solar cells, exemplifying the fusion of transparency and conductivity.
- Crystals in optics enhance the performance of lenses and prisms, influencing everything from photography to spectroscopy.
- In materials science, understanding transparency can guide the creation of new composite materials with tailored optical properties.
Moreover, industries like gemology rely heavily on crystal transparency to assess the quality and value of gemstones. The visual clarity of a gemstone impacts its market value and desirability significantly. With the increasing demand for transparent materials in advanced technology, the study of crystal transparency has profound implications for innovation across various sectors.
Fundamental Concepts of Light and Matter
Understanding the fundamental concepts of light and matter is crucial to our comprehension of crystal transparency. At its core, this relationship explains how light interacts with various materials, influencing their optical properties. This section delves into two primary areas: the nature of light and the optical principles governing crystals. It is essential to recognize that the way light travels through and interacts with crystals determines their visible characteristics. For both scientific inquiry and practical applications, grasping these concepts is fundamental.
The Nature of Light
Light is an electromagnetic wave that exhibits both particle and wave characteristics, a concept known as wave-particle duality. Photons, the fundamental particles of light, travel at a constant speed in a vacuum, approximately 299,792 kilometers per second. When light encounters a crystal, several phenomena can occur, including reflection, refraction, absorption, and scattering.
Key Aspects of Light:
- Speed: Decreases when entering dense mediums, like crystals.
- Wavelength: Different wavelengths correspond to different colors and energies.
- Frequency: Frequency is inversely related to wavelength and significantly affects light's interaction with materials.
The electromagnetic spectrum encompasses a range of wavelengths, from gamma rays to radio waves. The visible spectrum, which is primarily relevant in the context of crystals, usually spans from about 380 to 750 nanometers. Transparency in crystals often relates to how well they transmit these visible wavelengths.
Optical Principles of Crystals
The optical behavior of crystals can be characterized by several fundamental principles. These principles explain how crystals can be transparent, translucent, or opaque, depending on their atomic structure and the arrangement of their atoms. The interaction of light with matter is primarily a result of these structural characteristics.
Important Optical Principles Include:
- Refraction: The bending of light as it passes from one medium to another. The degree of bending depends on the refractive index of the materials involved. For example, crystals with a high refractive index may bend light significantly, enhancing their optical properties.
- Transmittance: This refers to the amount of light that passes through a substance without being absorbed. In transparent crystals, high transmittance is a key indicator of quality.
- Scattering: As light interacts with imperfections or inclusions in the crystal structure, it may scatter. Minimizing scattering improves transparency.
- Absorption: Certain wavelengths of light can be absorbed by the crystal, which can alter the perceived color and transparency.
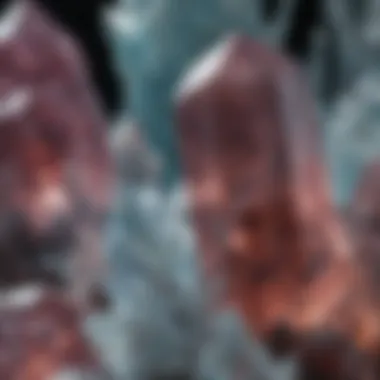
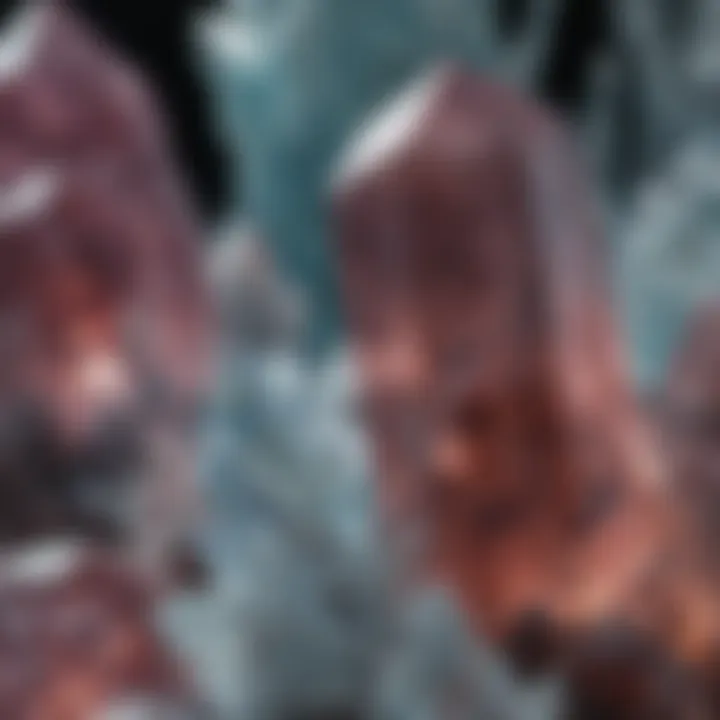
"The interaction of light with matter is pivotal to understanding optical behaviors in materials including crystals."
By understanding these foundational elements, researchers and professionals can better evaluate the transparency of various crystal types and their applications in technology and nature. This knowledge not only assists in theoretical explorations but also plays a significant role in applied sciences ranging from optical devices to material engineering.
Types of Crystals and Their Properties
Understanding the different types of crystals and their properties is essential when delving into the topic of transparency. Each type of crystal exhibits unique characteristics that influence how they interact with light. This section will provide an overview of three primary types: ionic, molecular, and covalent crystals. By examining these crystals, one can better comprehend their optical attributes, applications in various fields, and the factors that determine their clarity and transparency.
Ionic Crystals
Ionic crystals are formed by the electrostatic attraction between positively charged ions and negatively charged ions. This strong attraction creates a three-dimensional lattice structure known for its rigidity and high melting points. Common examples include sodium chloride and magnesium oxide.
The transparency of ionic crystals can vary significantly. In their pure form, many ionic crystals are transparent or semi-transparent. For instance, sodium chloride, commonly known as table salt, can transmit light effectively when processed correctly. However, impurities or defects in the crystalline structure can lead to reduced transparency.
Important Note: Ionic crystals typically display good mechanical strength, making them useful in applications requiring robust materials, such as optics and electronics.
Molecular Crystals
Molecular crystals are formed by molecules held together by weaker intermolecular forces such as van der Waals forces or hydrogen bonds. These crystals often exhibit varying degrees of transparency based on the nature of the molecules involved. Examples include ice and sugar.
The transparency of molecular crystals is generally influenced by their molecular makeup. In many cases, molecular crystals are less transparent than ionic crystals. However, some materials, like certain forms of quartz, can be quite transparent. The transparency of molecular crystals can also be affected by temperature changes. Increasing temperature may lead to changes in molecular order, thereby influencing the light transmission properties.
Covalent Crystals
Covalent crystals consist of atoms connected by strong covalent bonds in a continuous network. This structure leads to very high melting points and exceptional hardness. Common examples are diamond and silicon carbide. Covalent crystals generally exhibit high transparency, particularly in their pure form. Diamond, known for its exceptional optical clarity and brilliance, is the most famous covalent crystal.
The transparency in covalent crystals is largely maintained due to the uniformity of the atomic structure. Defects in crystal lattices, such as vacancies or foreign atoms, can disrupt the lattice and decrease transparency. Therefore, the purity of covalent crystals is critical in applications like optical lenses and light-emitting devices.
Measuring Transparency in Crystals
Measuring the transparency in crystals is a fundamental aspect of understanding their optical behavior. The clarity and light transmission properties of crystals play a vital role in various scientific and industrial applications. Knowing how to accurately assess transparency can provide insights into material quality, performance in optical devices, and potential uses in technology and research fields.
The ability to measure transparency is particularly important when selecting materials for specific applications. For example, in gemology, the value and attractiveness of gemstones are often determined by their clarity. In other fields, such as photonics, measuring transparency is essential for ensuring optimal performance of devices like lasers and sensors. Thus, the techniques and instruments developed for measuring transparency are critical in determining the functional integrity of crystalline materials.
Techniques and Instruments
A variety of methods and instruments are employed to measure the transparency of crystals. These techniques can be broadly divided into two categories: direct and indirect measurement methods.
- Optical Spectroscopy: This technique uses a light source to illuminate the crystal and measures the intensity of transmitted light across different wavelengths.
- Transmittance Measurement: Instruments like spectrophotometers measure how much light passes through a crystal. This data is compared against known standards to determine transparency.
- Microscopic Analysis: Scanning electron microscopes (SEM) can visualize the surface and internal structures of crystals, highlighting imperfections that may impact transparency.
- Refractive Index Measurements: Tools to assess the refractive indices of crystals can help infer light transmission characteristics.
Using these instruments effectively requires an understanding of the principles involved, as well as the limitations and potential errors associated with each method. Environmental conditions during measurements can also greatly impact results.
Quantifying Light Transmission
Quantifying light transmission in crystals involves determining the amount of light that successfully passes through a sample compared to the incident light. The most common quantitative measure is transmittance, which is expressed as a percentage.
To calculate transmittance, the following formula is used:
[ T = \fracI_tI_i \times 100 ]
Where:
- ( T ) is the transmittance,
- ( I_t ) is the transmitted light intensity,
- ( I_i ) is the incident light intensity.
Transmittance can be further analyzed using spectral data, allowing for absolute transparency evaluations across various wavelengths. This is particularly important in applications like optics, where specific wavelengths interact differently with materials.
It is crucial to ensure accurate measurements by accounting for factors such as surface quality, thickness of the crystal, and even environmental elements like temperature and humidity that might affect the results. One must also consider the type of light used during measurements—it can change the perceived transmission characteristics of the material being analyzed.
In summary, measuring transparency in crystals involves using a combination of techniques and understanding various influences on light transmission. By utilizing precise quantitative methods, researchers and professionals can unlock valuable insights into the optical properties of crystal materials.
Effects of Crystal Structure on Transparency
Understanding the effects of crystal structure on transparency is critical in material science. Crystals exhibit various structures, and these architectures significantly influence how light interacts with the material. Through this section, we will explore how specific crystal formations can enhance or impede transparency.
Crystals consist of atoms arranged in a highly ordered pattern. This order affects the way light is transmitted through them. The fundamental aspect here is the lattice structure, which can vary widely between different types of crystals. In some cases, a more ordered lattice can lead to higher transparency due to reduced scattering of light. Conversely, disordered structures may cause significant light scattering, effectively reducing transparency.
Lattice Structures and Their Influence
Lattice structures are vital to understanding crystal transparency. Different lattice arrangements contribute to unique optical properties. A common example is the cubic lattice structure found in materials like diamond. Diamonds are known for their exceptional optical clarity. This quality is attributed to the perfect arrangement of carbon atoms that minimizes defects and allows light to pass through with minimal obstruction.
On the other hand, some crystal systems, such as hexagonal or tetragonal lattices, may introduce more complexity in light transmission. As the atomic arrangement becomes less uniform, the likelihood of light scattering increases. This scattering effect can be detrimental, especially in applications where clarity is crucial, such as in optical lenses or photonics.
"Crystal lattice arrangement plays a crucial role in determining the transparency and optical clarity of materials."
Defects and Impurities
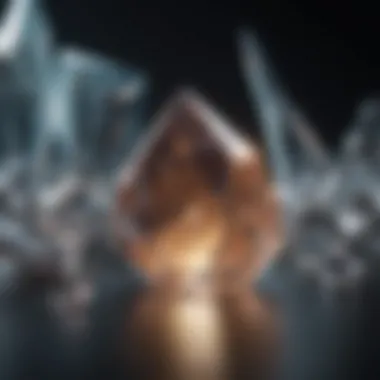
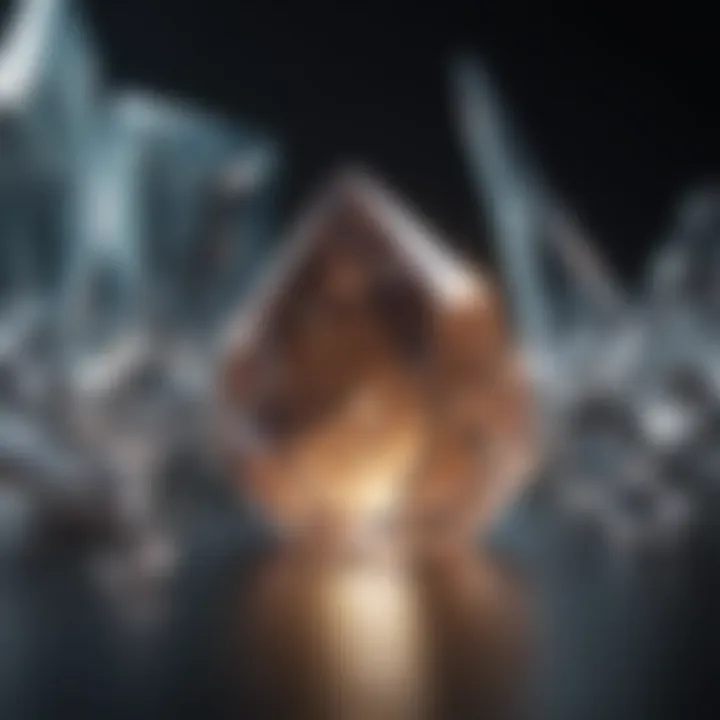
Defects and impurities within a crystal structure can greatly alter its transparency. These imperfections may result from the crystal growth process or external factors during formation. A crystal can harbor vacancies, interstitials, or substitutional impurities. All these contribute to a disruption of the ideal lattice structure.
Even small amounts of impurities can have a pronounced effect on light behavior within the crystal. For instance, the presence of certain color centers can lead to significant changes in transparency, often shifting it toward opacity. In many industrial applications, especially in electronics and optics, minimizing these defects is essential. Crystals like quartz are prized for their purity, which directly correlates to their transparency.
Moreover, the conditions during crystal growth, such as temperature and atmosphere, can introduce additional variables affecting transparency. Understanding and controlling these factors allows researchers to optimize crystal structures for desired optical properties, thus enhancing their practical applications.
In summary, both lattice structures and the presence of defects and impurities play significant roles in determining the transparency of crystals. Their analysis is key to advancing the field of material science and improving the utility of these materials in various applications.
Environmental Factors Affecting Crystal Transparency
Understanding the environmental factors that influence crystal transparency is crucial for both scientific study and practical application. Crystals do not exist in a vacuum; their optical properties can change based on temperature, pressure, and their surrounding chemical environment. This section will explore these factors in detail, providing insight into how they impact the clarity and light transmission properties of various crystals.
Temperature and Pressure Dependencies
The transparency of crystals can be significantly affected by variations in temperature and pressure. Temperature changes can lead to alterations in the crystalline lattice, affecting how light interacts with the material. For example, as the temperature increases, the atomic vibrations within a crystal may increase, potentially leading to a reduction in transparency due to scattering effects.
Pressure, on the other hand, can modify the bonds within the crystal lattice. Higher pressures often lead to denser packing of atoms, which can enhance or diminish the crystal's optical properties. In some instances, applying pressure may cause a transition to a different crystal phase that has better transparency characteristics. Research into materials like silicon carbide shows how pressure adjustments can change optical clarity, making it a significant area of study.
"Temperature and pressure play pivotal roles in determining how well crystals transmit light, impacting practical applications from electronics to gemology."
Chemical Environment
The chemical environment surrounding a crystal is another vital consideration affecting its transparency. When impurities or different chemical constituents are introduced, they can either enhance or obscure the transparency of a crystal. For instance, the presence of sodium ions in a potassium chloride crystal can change light absorption characteristics, resulting in a dimmer appearance.
Chemical reactions can also alter the crystal structure over time. For example, exposure to certain gases or liquids may result in surface defects or degradation of the crystal quality, leading to reduced clarity. This interaction is especially relevant in industrial applications, where the longevity and optical performance of crystals in environments with varying chemical compositions are pivotal.
In summary, the interplay between temperature, pressure, and chemical environments plays a critical role in defining the transparency of crystals. Understanding these factors ensures that scientists and engineers can optimize materials for their intended uses in various fields, from optical devices to advanced semiconductor technologies.
Applications of Transparent Crystals
The applications of transparent crystals are both diverse and impactful, extending across various fields such as optics, gemology, and scientific research. Understanding these applications enhances the comprehension of their significance in modern technology and theoretical studies. Transparent crystals serve as essential components in several technological innovations, making them invaluable for advancements in both industrial and academic settings.
Optical Devices
Transparent crystals are vital in the realm of optical devices. Many common devices, such as lenses and prisms, consist of these crystals. Their ability to bend and transmit light makes them fundamental in crafting optical systems. For example, materials like quartz and sapphire are often used due to their superior optical clarity and durability.
Some key uses in optical devices include:
- Lenses: In cameras and microscopes, transparent crystals allow precise manipulation of light.
- Laser systems: Crystals like potassium titanyl phosphate are used in frequency doubling of lasers, improving their output.
- Optical fibers: Fine silica fibers, drawn from transparent crystals, are integral to telecommunications, allowing for high-speed data transfer.
These applications highlight the crucial role that crystal transparency plays in enhancing optical technologies, leading to improved quality in imaging, communication, and various scientific fields.
Gemology
In gemology, the transparency of crystals significantly influences how gemstones are valued. Gems like diamonds, emeralds, and sapphires are not only prized for their color but also for their clarity. The degree of transparency affects the stone's aesthetic appeal and market price.
Factors in gemology linked to transparency include:
- Clarity: The presence of inclusions or flaws can greatly reduce a gem's transparency, affecting its value.
- Cut quality: The way a gemstone is cut can enhance or diminish its light-transmitting properties.
- Color: Transparent crystals can display vivid colors when light passes through, a desirable trait in many gemstones.
Thus, understanding crystal transparency is key for professionals in gemology, guiding both the appraisal and marketing of valuable stones.
Research and Development
The field of research and development increasingly relies on transparent crystals for innovative applications. In areas like materials science and telecommunications, researchers explore the unique properties of transparent materials to create new devices and technologies.
Key trends in research and development involving transparent crystals include:
- Nanocrystals: Research into nanostructured transparent materials has opened doors for new applications, including enhanced optical devices and sensors.
- Transparent conductors: Innovations in materials like indium tin oxide are paving the way for devices in solar energy and flexible electronics, redefining the interface between electronics and optics.
By investing in the study of transparent crystals, scientists aim to develop new methodologies, leading to breakthroughs that could revolutionize multiple sectors.
"Understanding the applications of transparent crystals is not just an academic exercise. It forms the backbone of numerous technologies, driving innovation in varied fields."
Transparent crystals are undeniably integral to advancements in science and technology. Their applications extend beyond traditional uses, inspiring new research and ushering in future breakthroughs.
Recent Advances in Crystal Transparency Research
Recent advances in the field of crystal transparency research have opened new avenues for applications in technology, materials science, and optics. This section highlights the significance of these breakthroughs and delves into two particularly noteworthy areas of investigation: nanocrystals and transparent conductors. These recent developments are enhancing our understanding of optical behaviors and the potential for improved materials, which are crucial in various industries.
Nanocrystals and Their Innovations
Nanocrystals have emerged as a focal point of research due to their unique optical properties and their size-dependent behaviors. At the nanoscale, the interaction of light with matter changes significantly. These advancements offer numerous benefits, including:
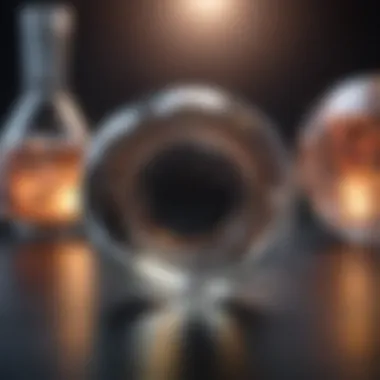
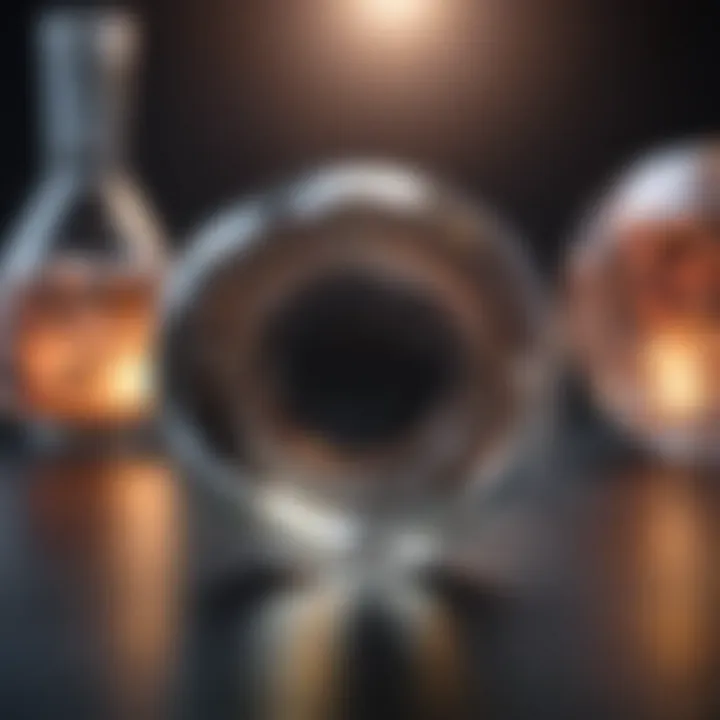
- Enhanced Optical Properties: The small size of nanocrystals allows for size-dependent tuning of their optical characteristics. This means that by simply altering the size, one can achieve different transparency levels or light absorption rates.
- Increased Surface Area-to-Volume Ratio: Nanocrystals have a larger relative surface area, which enhances their interaction with surrounding materials. This feature can lead to better performance in applications like photonic devices and sensors.
- Versatility in Composition: Researchers are experimenting with various materials to create nanocrystals that can exhibit desired properties. For instance, quantum dots made of cadmium selenide have shown promise for use in displays and solar cells due to their ability to emit specific wavelengths of light.
As researchers continue to innovate, the potential applications of nanocrystals in improving transparency in various devices is substantial. The incorporation of these materials can lead to lighter, more efficient optical devices, contributing to advances in both consumer electronics and scientific instruments.
Transparent Conductors
The development of transparent conductors is another significant area of progress, particularly for their applications in electronics and optoelectronics. Transparent conductors are materials that simultaneously possess electrical conductivity and optical transparency. They are essential in devices such as touch screens, solar cells, and LED displays. Key considerations in this field include:
- Material Selection: Traditionally, indium tin oxide (ITO) has been the most widely used transparent conductor. However, its high cost and limited availability prompt research into alternatives. Materials like graphene, metallic nanowires, and conductive polymers are being explored for their potential advantages.
- Improved Performance: Innovations aim to enhance the performance of transparent conductors, including optimizing their electrical conductivity while maintaining high transparency levels. This balance is critical for effective device operation, particularly in applications demanding high light transmission and efficient electrical properties.
- Scalability and Cost Efficiency: One of the primary challenges in using new transparent conductors is their scalability for mass production. Researchers are working to develop methods that allow for efficient manufacturing without compromising performance.
These advances in transparent conductors indicate a pivotal shift in design and functionality for modern electronic devices. With every incremental improvement, the role of transparency in crystals evolves, underpinning a wide range of future technologies.
In summary, exploring the realm of nanocrystals and transparent conductors signifies important strides in crystal transparency research, ultimately enhancing technological capabilities.
Through ongoing research and innovation, these areas not only elucidate the complexities of crystal behavior but also contribute significantly to the development of practical applications that impact everyday life.
Challenges in Achieving Desired Transparency
Understanding the challenges in achieving desired transparency in crystals is crucial for both scientific exploration and practical applications. Transparency defines how light interacts with materials, which is foundational in fields such as optics, materials science, and photonic engineering. Despite extensive research, several obstacles persist in both the selection of materials and their manufacturing processes.
Limitations of Current Materials
One major challenge involves the limitations of current materials used for crystals. While numerous substances can exhibit transparency, not all possess the necessary optical clarity across various wavelengths. For instance, some materials may appear transparent in visible light but show significant absorption in ultraviolet or infrared regions. This restricts their applications especially in specialized lighting, sensors and telecommunications.
Additionally, many transparent materials are brittle, leading to difficulty in shaping and integrating into practical applications. The mechanical properties often dictate how they can be utilized in devices like lasers or fiber optics. Certain crystal types, such as quartz or sapphire, demonstrate high transparency but struggle with internal defects that can scatter light, reducing overall performance.
Ultimately, these limitations mean that the quest for alternatives continues. Researchers examine combinations of materials or experimental approaches to enhance optical clarity while maintaining structural integrity.
Cost and Manufacturing Issues
Financial constraints and manufacturing complexities also pose significant challenges. The costs associated with producing highly transparent crystals can be prohibitive. Specialized sourcing of raw materials, along with sophisticated fabrication techniques, significantly raises the overall expenses. For example, the synthesis of high-grade synthetic sapphire crystal involves advanced processes like the Verneuil process or Czochralski method, which require strict environments and equipment.
Moreover, scaling up production while ensuring uniform transparency proves difficult. Many manufacturing methods often result in batch-to-batch variations, which affect the reliability of the end product. The lack of consistent quality increases costs through waste and rework during production cycles.
As the demand for transparent materials rises in sectors such as electronics and renewable energy, addressing these economic and practical concerns becomes increasingly urgent. Collaborations across disciplines, focusing on innovation and efficiency, are necessary to navigate the future landscape of crystal transparency.
The convergence of transparency, cost, and effective production techniques will shape future advancements in crystal applications.
The ongoing quest for transparency in crystals reveals a complex interplay of material limitations and economic pressures. A deeper understanding of these challenges will pave the way for innovations that capitalize on the potential of transparent crystals in advanced applications.
Future Directions in Crystal Transparency Studies
The realm of crystal transparency research is evolving. Understanding transparency is not simply an academic pursuit; it has practical implications across various industries. As we consider future directions in this field, it is essential to think about how advancements can lead to new materials and applications that drive innovation forward.
Researching novel materials could pave the way for breakthroughs in crystal transparency. Emerging synthetic methods allow for the creation of bespoke crystals. These tailored materials can exhibit specific transparency properties required for advanced optical devices. Moreover, the quest for environmentally sustainable and efficient materials remains paramount in today's manufacturing landscape.
"The quest for innovation in crystal transparency can redefine industries ranging from telecommunications to medicine."
Emerging Research Areas
Currently, several emerging research areas are capturing the attention of scientists and engineers alike. One notable area is the exploration of 2D materials, such as graphene, which exhibit exceptional optical properties. These materials could complement traditional crystal applications and open doors for flexible photonic devices.
Another promising avenue is the study of nanostructured crystals. Researchers are focusing on how altering crystal structures at the nanoscale can influence light transmission and enhance transparency. This could lead to advancements in smart coatings for various surfaces, allowing them to adapt based on environmental conditions.
There is also increasing interest in organic-inorganic hybrids, combining the advantageous properties of both organic and inorganic crystals. These hybrids are anticipated to provide unique transparency characteristics suitable for lightweight and multifunctional applications, such as solar cells and sensors.
Interdisciplinary Approaches
To maximize the potential for innovation, adopting interdisciplinary approaches is crucial. Collaborations across fields such as physics, chemistry, materials science, and engineering can lead to enriched perspectives and breakthrough ideas. For instance, physicists can provide insights into the fundamental theories governing light interactions with matter, while chemists can develop new synthesis routes for advanced materials.
Additionally, the integration of computational modeling with experimental studies has significantly advanced the understanding of crystal behavior. By simulating light interactions at a molecular level, researchers can predict how alterations in structure influence optical properties before actual materials are synthesized. This not only saves time but also reduces costs associated with trial and error in material production.
Ultimately, the exploration of crystal transparency from diverse angles will be essential in driving the field into uncharted territories, where many practical implications await discovery.
Closure
The conclusion of this article highlights the profound importance of crystal transparency in various scientific and industrial fields. As we examined the properties of crystals, their types, and the principles governing their transparency, it becomes clear that understanding these aspects is critical for advancements in technology. Transparent crystals are not only essential components in optical devices but also play significant roles in fields such as semiconductor technology and photonics.
Summary of Key Insights
In summary, we have explored several key insights throughout this article:
- Crystal transparency is influenced by their crystal structure and the presence of defects or impurities. This understanding allows scientists to engineer materials with desired transparency levels.
- Environmental factors such as temperature and chemical composition can affect crystal clarity, which is a significant consideration for their applications in real-world scenarios.
- The rise of nanocrystals and transparent conductors indicates a pathway towards innovative technologies that could transform the future of electronics.
Furthermore, the challenges of cost and manufacturing must be addressed to realize the full potential of transparent crystals in various applications. The developing technologies and methods in transparency measurement provide exciting prospects for future research.
Final Thoughts on Crystal Transparency
Reflecting on the nature of crystal transparency, it is crucial not to overlook its implications in the broader context of materials science. As we continue to advance in our understanding and manipulation of crystal properties, the significance of transparency can lead to breakthroughs in energy efficiency and optical clarity.
"The study of crystal transparency not only enriches our knowledge but also drives technological advancements across many fields."