Techniques and Considerations for Running DNA Gels
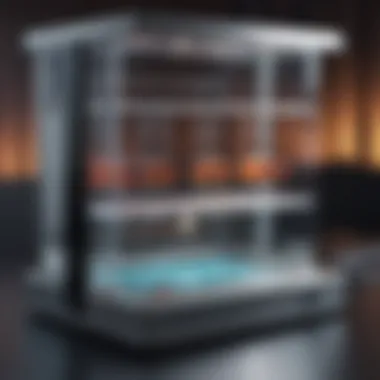
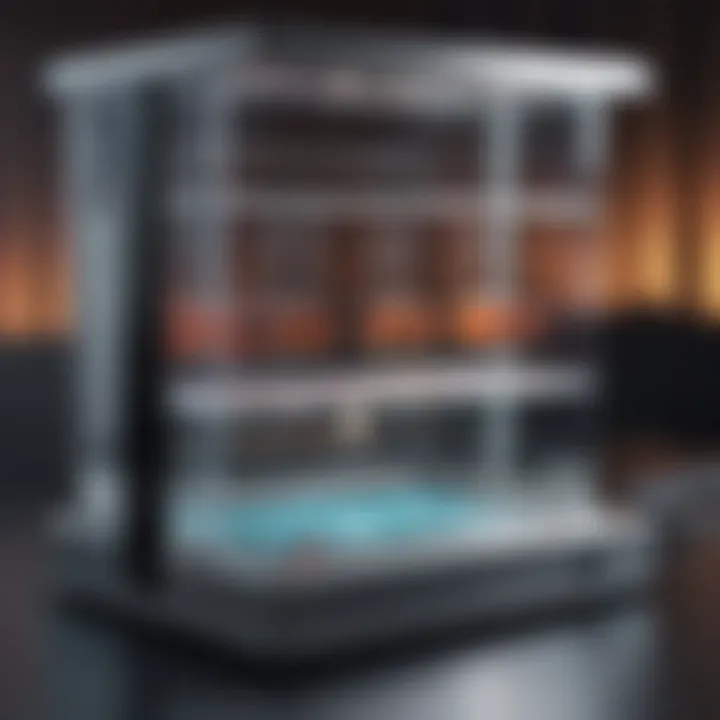
Intro
Gel electrophoresis is an essential technique in molecular biology, facilitating the separation and analysis of DNA fragments. Understanding how to effectively run a gel for DNA is critical for both research and educational purposes. The process allows for visualizing genetic material, assisting in tasks such as DNA fingerprinting, cloning, and gene expression analysis. In this article, we explore the various methods, technical nuances, and protocols relevant to running a gel for DNA.
Research Overview
Gel electrophoresis is widely utilized across laboratories for its efficiency and reliability. It separates charged particles in an electric field, with DNA moving through a gel matrix that impedes larger fragments more than smaller ones. Understanding its application can significantly enhance experimental outcomes.
Summary of Key Findings
The following points summarize pivotal aspects of running a gel for DNA:
- Types of gels: Agarose and polyacrylamide are the most commonly used gels due to their distinct characteristics. Agarose gels are generally used for larger DNA fragments, while polyacrylamide gels are preferred for smaller fragments.
- Buffer systems: A variety of buffer solutions can affect DNA mobility. Common buffers include TAE (Tris-Acetate-EDTA) and TBE (Tris-Borate-EDTA). Each has different pH values and ionic strengths, impacting the overall electrophoresis effectiveness.
- Staining agents: Ethidium bromide is frequently used to visualize DNA, although safer alternatives like SYBR Safe are becoming more popular.
- Running conditions: Voltage and running time must be optimized based on gel composition and fragment sizes to achieve desirable separation.
Relevance to Current Scientific Discussions
The relevance of gel electrophoresis extends beyond simple analysis. It plays a crucial role in various fields such as forensic science, genetic engineering, and biotechnology. As new techniques develop, such as CRISPR gene editing, understanding the separation of DNA will remain key in numerous advanced applications. The continuous evolution of the methods—especially in optimizing efficiency and safety—reflects ongoing discussions within the scientific community.
Methodology
Methodology in running a gel includes several critical steps that ensure effective outcomes. Proper approach and design are paramount for reliable results.
Research Design and Approach
- Gel Preparation: Solutions are carefully measured to achieve the desired concentration based on target DNA fragment sizes.
- Electrophoresis Setup: A gel casting tray is utilized to pour the gel mixture, followed by the insertion of a comb to create wells for samples.
- Sample Loading: DNA samples, often mixed with a loading dye, are pipetted into the wells. This step requires precision to avoid cross-contamination.
Data Collection and Analysis Techniques
After running the gel, the bands are visualized, and the following techniques can be applied for data collection:
- Photodocumentation: Images are captured under UV light to analyze band patterns.
- Size Estimation: Comparison with a DNA ladder allows for estimating the size of DNA fragments.
"The accuracy of gel electrophoresis hinges on meticulous technique and attention to detail throughout the process."
Such careful attention to the methodology not only enhances experiments but also contributes significantly to reproducibility in scientific research.
Intro to Gel Electrophoresis
Gel electrophoresis is a fundamental technique in molecular biology that separates nucleic acids based on size. It serves as a crucial step in various applications, including genetic analysis, cloning, and forensic investigations. Understanding this method is necessary for students and professionals alike, providing insight into the manipulation and analysis of DNA.
Its importance extends beyond merely separating DNA fragments. This technique allows researchers to visualize genetic material, enabling them to draw conclusions about genetic sequences and variations. By employing gel electrophoresis, scientists can identify specific DNA fragments, quantify them, or assess their integrity. Thus, gaining proficiency in this technique is essential for anyone involved in genetic research or biotechnology.
Definition and Purpose
Gel electrophoresis can be defined as a technique that utilizes an electric field to migrate charged molecules through a gel matrix. This migration is influenced by the size and charge of the molecules. The purpose of this technique is multifaceted. Primarily, it is used to isolate DNA fragments, allowing researchers to analyze them individually. It also plays a vital role in confirming the success of DNA modifications such as cloning or PCR amplifications.
The gel matrix, typically made from agarose or polyacrylamide, provides a medium through which DNA can travel. Smaller DNA fragments tend to move faster and farther than larger ones, creating a distinct separation that can then be visualized. This separation is crucial for downstream applications, including sequencing and further genetic manipulation.
Historical Context
The development of gel electrophoresis has a rich history dating back to the early 20th century. Initial methods involved the separation of proteins, which laid the groundwork for the introduction of nucleic acid analysis later on. In the 1970s, the advent of agarose gel electrophoresis revolutionized genetic research, simplifying the process of DNA analysis. As technology advanced, so did the methods used for gel electrophoresis, leading to the refinement of protocols and the increased efficacy of the technique.
In the years that followed, polyacrylamide gel electrophoresis emerged as a more versatile option for separating DNA fragments, particularly useful for smaller DNA pieces. This advancement allowed for higher resolution in the separation process, providing greater detail in genetic studies. Today, gel electrophoresis stands as a cornerstone of molecular biology, impacting various fields, from medical genetics to environmental science. Understanding its background is key to appreciating its application in contemporary research.
Understanding DNA and Its Properties
Understanding DNA and its properties is fundamental in the context of gel electrophoresis. DNA molecules are the carriers of genetic information in living organisms, and their unique physical and chemical properties play a crucial role in their separation and analysis during electrophoretic techniques. This section provides insights into two key aspects: the structure of DNA and its size and charge, both of which influence how DNA behaves in a gel environment.
Structure of DNA
The structure of DNA is often described as a double helix. This shape is formed by two long strands of nucleotides twisted around each other. Each nucleotide comprises a sugar, a phosphate group, and a nitrogenous base. The four bases—adenine, thymine, cytosine, and guanine—pair specifically (adenine with thymine and cytosine with guanine) to create the rungs of the helix. The complementary base pairing is essential for DNA replication and function.
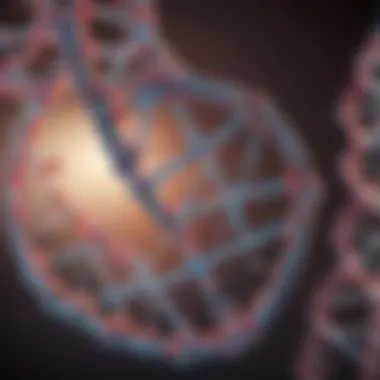
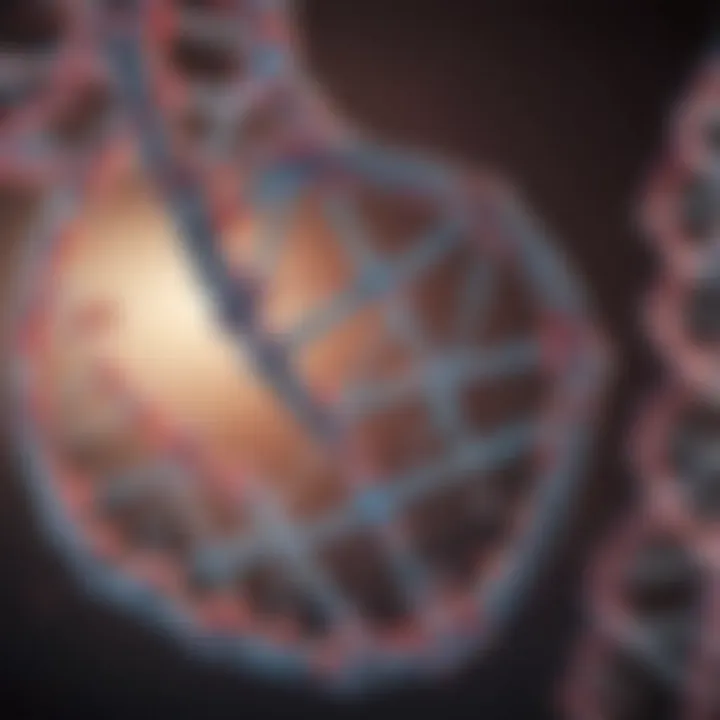
In gel electrophoresis, the double helical structure of DNA plays a pivotal role in how the molecules migrate through the gel matrix. As the gel is subjected to an electric field, the nucleotide strands that are intact tend to move differently than those that are fragmented. The intact molecules are generally longer and face more resistance. Understanding this characteristic allows researchers to design experiments that can differentiate between various DNA fragments based on size.
Size and Charge of DNA
The size and charge of DNA molecules are vital characteristics that influence their movement in gel electrophoresis. DNA is negatively charged due to its phosphate backbone. When an electric field is applied, the negatively charged DNA migrates towards the positively charged anode.
The size of DNA also significantly affects its migration rate. Smaller fragments migrate faster through the gel compared to larger ones. This size-based separation is what allows researchers to distinguish between DNA fragments after the electrophoresis run.
In summary, a solid grasp of DNA’s structure and its size and charge characteristics is imperative for effectively running gels in DNA analysis. Researchers can utilize this knowledge to achieve more accurate results and better interpret their data in various applications of molecular biology.
Understanding the properties of DNA is key for effective gel electrophoresis, as these factors directly influence migration patterns in the gel.
Components of Gel Electrophoresis
The components of gel electrophoresis play a critical role in ensuring the accurate and effective separation of DNA fragments. Understanding these components helps in optimizing conditions for gel running, which can lead to improved clarity and resolution of the resulting bands. This section delves into two primary components: the type of gels used and the buffer systems that support the process.
Agarose vs. Polyacrylamide Gels
Choosing between agarose and polyacrylamide gels is crucial for successful electrophoresis. Agarose gels, made from the agarose polymer, are widely utilized for separating larger DNA fragments, typically ranging from 100 base pairs to over 25,000 base pairs. Their low cost and ease of preparation make these gels a preferred choice for many laboratories.
On the other hand, polyacrylamide gels offer higher resolution and are better suited for smaller DNA fragments, around 1 to 500 base pairs. These gels allow more precise size discrimination. However, they require more complex handling and can be more toxic to prepare because of the acrylamide monomer, which is a neurotoxin.
When deciding which gel to use, consider the size of the DNA fragments you are working with and the required resolution.
Buffer Systems Used
The buffer systems in gel electrophoresis are equally important, as they maintain the pH and provide the ions necessary for conducting the electric current during separation. Commonly used buffer systems include Tris-Borate-EDTA (TBE) and Tris-Acetate-EDTA (TAE). Each of these buffers exhibits distinct properties that can influence the efficiency of the gel run.
- TBE: Tris-Borate-EDTA is known for providing high resolution and is useful when running gels for long durations. It can, however, lead to the precipitation of contaminants that may interfere with visualization.
- TAE: Tris-Acetate-EDTA has a lower buffering capacity than TBE, but it provides higher sensitivity for detecting DNA, which can be beneficial in applications like Southern blotting.
Selecting the appropriate buffer system significantly impacts the results of electrophoresis. The buffer should align with the specific requirements of the experiment and the types of analysis to be performed.
Understanding the components of gel electrophoresis is essential. It ensures successful separation of DNA fragments, which is indispensable for reliable results across a variety of applications in molecular biology.
Preparation of Gel and Samples
The preparation of gel and samples is a critical stage in the process of DNA analysis through gel electrophoresis. It requires precision and attention to detail, as the quality of the gel and the samples directly impacts the results obtained from the electrophoresis run. An inadequately prepared gel can lead to poor resolution of DNA bands, making subsequent analysis ineffective. The goal here is to ensure that both gel and samples are optimally prepared to facilitate accurate and reliable results.
Gel Casting Techniques
In gel casting, the selection of agarose or polyacrylamide is essential. Agarose is preferred for larger DNA fragments, while polyacrylamide is suitable for smaller fragments due to its tighter control of pore sizes. The concentrations of the gel can be adjusted based on the size of the DNA being analyzed. Higher agarose concentrations create smaller pores, which can hinder larger DNA migration. Conversely, lower concentrations allow larger fragments to travel more freely.
The gel casting process involves melting the agarose in a buffer solution until it becomes a clear, uniform liquid. Subsequently pouring the liquid into a mold with a comb creates wells for sample loading. It is crucial to allow the gel to solidify completely before proceeding; otherwise, the integrity of bands may suffer during the run.
Tip: Always ensure the gel has cooled appropriately to avoid distortion of band patterns.
Once the gel is solidified, the comb is removed carefully to avoid damaging wells. Proper gel casting also includes minimizing air bubbles, which can interfere with migration. Techniques such as slowly pouring the agarose or using a pipette aid in achieving a smooth surface.
Sample Loading and Dilution
Loading samples into the gel requires precision to ensure that bands are distinct and easily distinguishable during visualization. Samples should be diluted adequately, as overly concentrated samples may lead to smearing rather than well-defined bands. Standard practices involve mixing samples with a loading buffer, which helps to increase density and provides color, allowing for real-time monitoring of sample progression during the run.
It is essential to load samples into separate wells without cross-contaminating them. Utilizing a micropipette for loading helps to achieve this. The angle at which samples are loaded is also important; samples should be added at a low angle to the well, minimizing disturbance to the gel.
A common challenge in this stage is maintaining consistent loading. Variability can arise from different volumes being loaded into wells, leading to uneven results. Developers can employ a ladder or marker DNA alongside their samples. The ladder allows for the size estimation of the DNA bands post-run, thereby aiding in interpreting the results accurately.
In summary, the preparation of gel and samples is not a mere formality but a foundational step that has significant implications on the outcomes of electrophoresis. This detailed attention ensures that subsequent results reflect the true nature of the DNA samples, contributing to the overall success of the experimental analysis.
Running the Gel
The running phase of gel electrophoresis represents a critical step in the overall process of DNA analysis. This is where the separation of DNA fragments takes place based on their size and charge. By understanding the nuances involved in this phase, one can greatly enhance the efficiency and accuracy of the outcomes. The specifics of electrophoresis conditions must be meticulously monitored to avoid any variability in results, which could lead to misinterpretation of data. This section will delve into the essential electrophoresis conditions and how to effectively monitor the run, ensuring optimal performance.
Electrophoresis Conditions
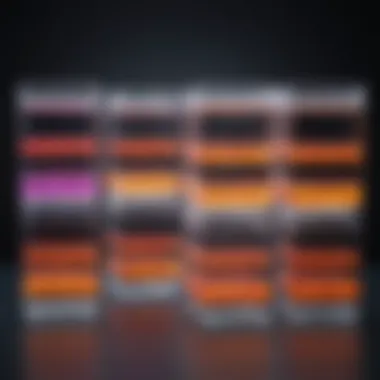
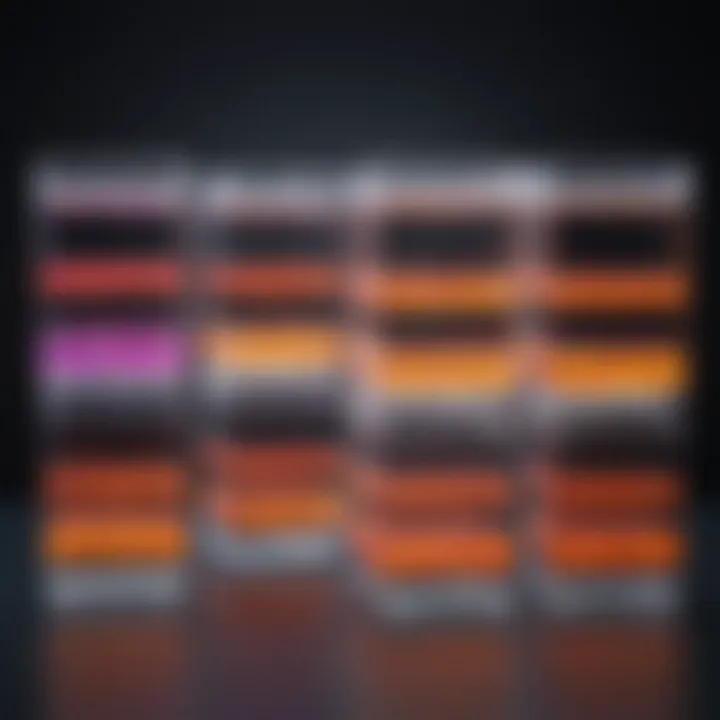
Electrophoresis conditions play a significant role in the behavior of DNA fragments within the gel. Key factors include the voltage applied, the running time, and the temperature of the gel. Each of these elements must be carefully considered to achieve reliable results.
- Voltage: The voltage determines the rate at which DNA travels through the gel. Higher voltages can lead to faster runs but may also cause overheating or distortion of bands. A more moderate voltage often yields clearer separation.
- Running Time: This must be adjusted according to the total length and size of the DNA fragments. Too short of a run may result in overlapping bands, while too long can lead to diffusion and poor resolution.
- Temperature: Gel temperature can affect the viscosity of the buffer, which in turn influences DNA migration. Monitoring and maintaining a stable temperature is crucial for reproducibility of results.
The careful calibration of these factors ensures that DNA separation is both efficient and accurate, resulting in high-quality data for further analysis.
Monitoring the Run
Monitoring the electrophoresis run is essential for both troubleshooting and ensuring the protocol is correctly executed. Key aspects include watching for band migration and overall gel integrity. Several methods can aid in this process:
- Visual Inspection: Regularly check the gel for the movement of DNA bands towards the positive electrode. This can be crucial to identifying any immediate issues.
- Sample Tracking Dyes: Using loading dyes can provide visual cues during the run. These dyes travel alongside the DNA, enabling easy tracking of progress.
- Documentation: Keeping detailed notes on the run's conditions, including voltage, time, and any observed anomalies, can be invaluable for both analysis and future experiments.
By paying attention to these vital aspects during the running of the gel, researchers can not only improve the reliability of their results but also facilitate a smoother workflow in their molecular biology applications.
In summary, mastering the running gel process requires an understanding of the critical electrophoresis conditions and effective monitoring techniques, promoting accurate and reproducible outcomes.
Visualization of DNA Bands
Visualization of DNA bands is a crucial phase in gel electrophoresis, serving as a bridge between the technical execution of the experiment and the interpretation of results. This step allows scientists to confirm the presence, size, and quantity of DNA fragments, providing valuable insights into genetic material. Without proper visualization, even the most meticulously prepared gels and samples may yield inconclusive or misleading information.
The significance of effectively visualizing DNA bands stems from its implications for various biological applications. For instance, in genetic analysis, it facilitates the identification of specific genes or mutations. In forensic science, it can help in matching DNA to solve criminal cases. Moreover, accurate visualization can assist in determining the success of molecular cloning experiments. Overall, the information obtained from properly visualized bands directs subsequent steps in research, whether confirming a hypothesis or guiding experimental modifications.
Staining Techniques
Staining techniques are a fundamental aspect of visualizing DNA bands after running a gel. These methods enhance contrast, allowing for clear differentiation of DNA fragments. Several staining agents are commonly used, each with particular benefits and considerations:
- Ethidium Bromide: This is one of the most traditional stains. It intercalates between DNA bases and fluoresces under UV light. However, it is a mutagen, presenting safety concerns that require careful handling.
- SYBR Green: This stain is preferred for its sensitivity and lower toxicity. It binds to DNA with high affinity and provides a clearer signal than ethidium bromide.
- GelGreen: This is a safer alternative that also offers a strong fluorescence signal. It does not pose significant environmental harms compared to traditional dyes.
Selecting the appropriate staining technique hinges on factors like sensitivity requirements, safety, and potential downstream applications. Understanding the chemistry of these stains and their interaction with DNA enables better choices tailored to specific experimental needs.
Imaging Methods
Once DNA bands are stained, the next step is imaging, a process that captures the visual data of the gel. Imaging methods translate fluorescence into quantifiable data, allowing researchers to analyze their results rigorously. Different techniques can be employed for this purpose:
- UV Transilluminators: A standard approach in many laboratories, UV transilluminators provide a straightforward method for visualizing DNA. The gel is placed on a light box that emits UV light, causing the stained DNA to glow.
- Gel Documentation Systems: These systems often combine UV light with high-resolution cameras. They provide a more user-friendly and safer environment than traditional UV exposure.
- Laser Scanners: Advanced imaging options involve laser-based systems. These can offer increased sensitivity and resolution, allowing for precise measurements of band intensity and size.
Selecting an imaging method involves a balance between available resources and specific research goals. Depending on the sensitivity required, different imaging options can provide complementary data, advancing understanding of experimental results.
Proper visualization techniques are critical for accurate interpretation of DNA fragments in gel electrophoresis, influencing research outcomes across multiple disciplines.
Data Analysis from Gel Results
Data analysis of gel results is a pivotal step in the process of DNA gel electrophoresis. This phase involves interpreting the patterns formed by DNA fragments after they have been subjected to an electric field. The clarity and reliability of the analysis directly correlate with the quality of experimentation conducted prior. Proper analysis can yield significant insights into the molecular weight, integrity, and even the potential functions of the DNA under examination.
Interpreting Band Patterns
The interpretation of band patterns is essential in assessing the outcomes of gel electrophoresis. Each band represents DNA fragments that have migrated through the gel matrix, usually based on size. When analyzing these bands, several considerations come into play:
- Size Estimation: Comparing the position of DNA bands to a standard DNA ladder allows researchers to estimate the sizes of unknown fragments. This is fundamental for identifying specific genetic markers.
- Band Intensity: The intensity of bands indicates the abundance of DNA present. Starker bands suggest higher concentrations, which may correlate with the effectiveness of the initial sample preparation or PCR amplification.
- Specificity: Bands should be analyzed for their specific location. Secondary bands or smear may indicate non-specific reactions or degraded samples.
- Replicability: Observing consistent banding patterns across multiple runs can validate the reliability of the experimental setup. Variations in patterns may necessitate further investigation into procedural anomalies.
Using these guidelines, researchers can derive meaningful conclusions from gel images, allowing for a deeper understanding of the results.
Software for Analysis
Advancements in technology have led to the development of software tools designed for the analysis of gel electrophoresis results. These tools can streamline the process, offering benefits such as increased accuracy, detailed statistical analysis, and enhanced visualization of results.
Common software options include:
- Image Lab: Developed by Bio-Rad, it allows for straightforward analysis of band patterns and densities.
- GelAnalyzer: A free tool that provides comprehensive analysis functionalities, from measuring band sizes to calculating molecular weights.
- TotalLab: This provides sophisticated options for managing and analyzing multiple gel images simultaneously.
When choosing software, consider the following:
- User-Friendliness: Ease of use can reduce the learning curve, especially for students.
- Functionality: Ensure it meets specific analytical needs relevant to your research.
- Support and Updates: Check for ongoing support and software updates to address any issues that may arise.
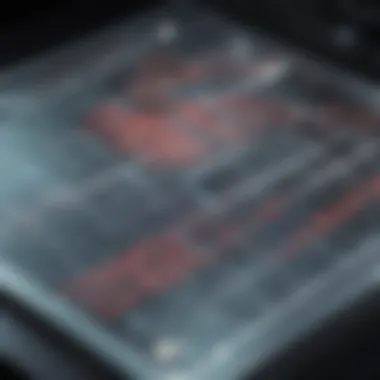
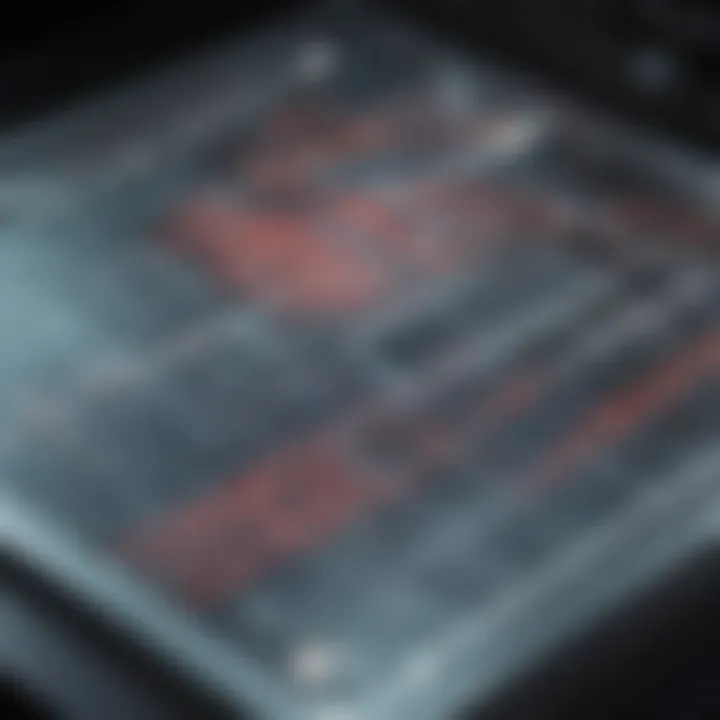
Utilizing software in conjunction with traditional analysis techniques can substantially enhance the quality of the interpretation, thereby leading to more accurate scientific conclusions.
Common Pitfalls and Troubleshooting
Understanding the common pitfalls in gel electrophoresis is essential for obtaining reliable results. Troubleshooting is part of the scientific process. Identifying issues early can save time and resources. Addressing problems during gel casting and when interpreting results is crucial for effective DNA analysis.
Issues During Gel Casting
The gel casting process is foundational in gel electrophoresis. Poor casting can lead to numerous complications. Common issues include air bubbles, inconsistently mixed agarose, and improper temperature control. Air bubbles can create disturbances, leading to uneven lanes or blank spots in the gel. To avoid this, carefully pour the melted agarose and allow it to settle. Ensuring that the agarose is mixed thoroughly with the buffer is equally vital. Any unmixed agarose will give rise to variability in gel consistency.
Moreover, temperature must be monitored closely while preparing the gel. If the agarose solution is too hot or too cold when poured, it may solidify improperly. This can cause the gel to crack or form holes. Using a thermometer to check the temperature can help maintain the right consistency.
Additionally, using the right concentration of agarose is essential. Different DNA fragments require specific concentrations for optimal separation. Higher concentrations might be beneficial for smaller fragments, while lower concentrations work better for larger DNA pieces. Therefore, selecting the right agarose type and concentration is a decision that should not be overlooked in the gel-preparation phase.
Problems with Results
Once the gel is run, various issues can arise that affect the quality and interpretability of the results. One frequent problem is the presence of smeared bands, which can indicate several underlying issues. Contamination of samples, overloading wells, or running the gel for too long can all contribute to this. To maintain clarity in the bands, ensure samples are diluted appropriately and avoid overfilling wells.
Another common problem is the fainess of DNA bands, which may occur due to insufficient staining or poor imaging conditions. Inadequate staining could lead to low sensitivity, making it difficult to visualize bands. Paying attention to staining protocols, such as the duration and concentration of the staining solution, is important. Furthermore, using suitable imaging methods is critical to enhance the visibility of DNA bands under UV light.
In the analysis phase, interpreting band sizes accurately can be challenging if a DNA ladder is not used as a reference. Always include a DNA marker to aid in sizing the fragments properly.
Maintaining a systematic approach to troubleshooting will ensure replicability and accuracy in results, ultimately enhancing the reliability of gel electrophoresis as a technique in molecular biology.
By recognizing these common pitfalls and implementing preventive measures, researchers can significantly improve their chances of obtaining clear and interpretable results from their gel electrophoresis experiments.
Applications of Gel Electrophoresis
Gel electrophoresis serves as a critical tool in molecular biology. Its applications are broad, impacting various fields such as genetic analysis, molecular cloning, and forensic science. The ability to separate DNA fragments accurately enables researchers to visualize genetic information, manipulate DNA for cloning, and even solve criminal cases. Each application presents specific considerations that reinforce the technology's relevance in modern science.
Genetic Analysis
In the realm of genetic analysis, gel electrophoresis is indispensable. It allows scientists to separate DNA fragments by size, which aids in identifying genetic variations. This capability is essential for applications like polymerase chain reaction (PCR) product assessment, genetic fingerprinting, and haplotyping. The visualization of distinct bands on a gel after electrophoresis can reveal information regarding alleles, mutations, or genetic diseases.
"Gel electrophoresis is a fundamental technique that can elucidate genetic diversity and hereditary diseases, making it vital for modern genetics research."
Moreover, the technique can be used to confirm the presence of genetically modified organisms (GMOs) in food products. By running specific primers in a gel, researchers can determine if GMO markers are present or absent with a high degree of accuracy. This aspect further highlights the significance of gel electrophoresis in ensuring food safety and regulatory compliance.
Molecular Cloning
Molecular cloning relies on gel electrophoresis for several critical processes. When isolating DNA fragments, researchers utilize this technique to separate desired sequences from unwanted ones. After restriction enzyme digestion, running a gel enables the identification of the correct fragments, ensuring the right pieces are used during the cloning process.
In addition, gel electrophoresis assists in checking the quality and quantity of the insert DNA before ligation into vectors. The precise control it offers in size separation ensures that only intact DNA fragments are selected, improving cloning efficiency and success rates. The process becomes streamlined when gel extraction techniques are employed, allowing scientists to retrieve DNA bands of interest cleanly.
Forensic Science
The application of gel electrophoresis in forensic science has revolutionized criminal investigations. This technique facilitates the analysis of DNA samples, which can be pivotal in solving crimes. By comparing DNA profiles from crime scenes with known samples, forensic experts can ascertain potential suspects with high accuracy.
Additionally, gel electrophoresis plays a role in paternity testing and mass disaster victim identification. The ability to separate and analyze DNA fragments allows for conclusive links between individuals, contributing to legal proceedings or providing closure to families affected by tragedies. Through these facets, gel electrophoresis demonstrates its importance not only in scientific research but also in societal applications.
Future Directions in Gel Electrophoresis
As technology evolves, the field of gel electrophoresis experiences significant transformations. Understanding future directions in this area is essential for practitioners of molecular biology. These innovations not only enhance the efficiency and accuracy of DNA analysis but also expand the applications of gel electrophoresis across various scientific disciplines. By anticipating these developments, researchers can better plan their experiments and integrate new methods into their workflows.
Innovations in Gel Materials
The materials used to create gels are constantly being refined. New types of gels are emerging, designed for enhanced separation and resolution of DNA fragments. For instance, the development of bioengineered agarose and novel polyacrylamide formulations aim to improve the clarity and reproducibility of results.
- High-resolution Gels: Innovations have led to gels that allow for the separation of even smaller DNA fragments. This could prove crucial in applications such as SNP detection and genetic fingerprinting.
- Eco-friendly Materials: As sustainability becomes a priority, research on biodegradable gel materials is gaining traction. Such materials reduce environmental impact while maintaining the efficacy of traditional gels.
These advancements represent significant leaps forward but also bring forth considerations about their reliability and compatibility with existing protocols.
Advancements in Imaging Technology
Alongside improvements in gel materials, imaging technologies are also advancing rapidly. High-resolution imaging systems are now being developed, providing clearer and more detailed images of DNA bands. This is particularly beneficial for accurate band quantification and analysis.
- Real-time Imaging: New systems can capture images in real-time, allowing researchers to monitor their gels as they run. This capability provides immediate insights into the progression of electrophoresis, facilitating more informed decision-making.
- Digital Analysis Tools: Along with improved imaging, the integration of sophisticated software for image analysis enhances the interpretation of results. These tools can automate band detection and quantification, significantly reducing analysis time.
These advancements underscore the importance of staying updated with technology in the biochemical investigation landscape. They can substantially influence the reliability and accuracy of experiments.