Chemical Composition of Polymers: An In-Depth Analysis
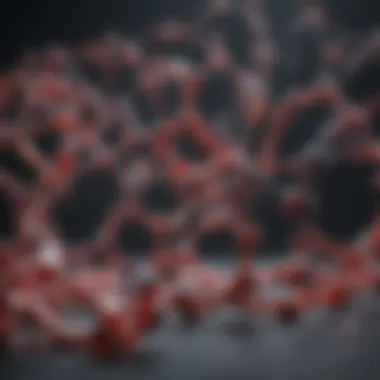
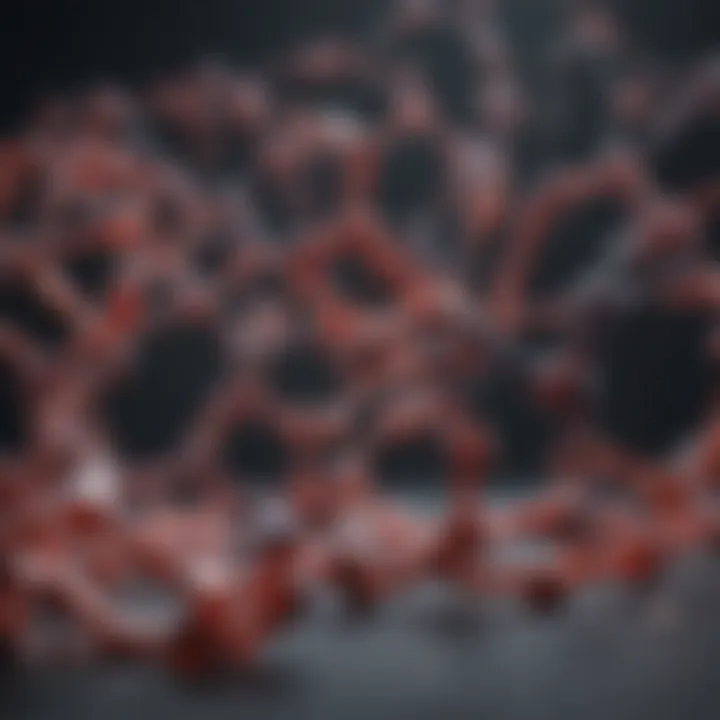
Research Overview
The exploration of polymer chemistry has gained substantial attention over the years. Understanding the chemical composition of polymers is foundational to many scientific fields, including materials science, biochemistry, and engineering. This section presents a detailed analysis of the essential points covered in this article.
Summary of Key Findings
The article elucidates several critical findings about polymer composition. Key points include:
- Molecular Structure: The arrangement of atoms within a polymer determines its mechanical properties, thermal stability, and reactivity.
- Formation Processes: Various methods, such as addition polymerization and condensation polymerization, play significant roles in defining polymer structure and properties.
- Functional Groups: The presence of specific functional groups contributes notably to a polymer's properties, leading to diverse applications across industries.
- Types of Polymers: From natural polymers like cellulose and proteins to synthetic polymers such as polyethylene and polystyrene, understanding the differences is crucial for applications.
"The relationship between a polymer's chemical structure and its physical properties is a key consideration in both research and application."
Relevance to Current Scientific Discussions
In the context of ongoing scientific discourse, the chemical composition of polymers is vital. Researchers focus on sustainable approaches to create bio-based polymers, emphasizing environmental considerations. This has led to discussions on:
- The impact of polymers on sustainability and recycling.
- Development of smart polymers for advanced applications in medicine and technology.
- Innovations in polymer synthesis to improve performance characteristics.
By analyzing these areas, this article serves as a significant contribution to the current scientific landscape, providing insights into the chemical composition of polymers and their broader implications.
Methodology
To thoroughly understand the chemical composition of polymers, a systematic approach was adopted to assess and analyze the existing literature and empirical data.
Research Design and Approach
The design of this study was focused on a comprehensive review of existing research. This approach involved:
- Collecting papers from peer-reviewed journals focused on polymer chemistry.
- Synthesizing information from various sources to provide a holistic view of polymer composition.
Data Collection and Analysis Techniques
Data was collected primarily through:
- Literature Review: Analysing published studies on the synthesis, structure, and applications of various polymers.
- Comparative Analysis: Examining the properties of different polymers to understand their chemical makeup and functionality.
The resultant data provided a nuanced understanding of polymer chemistry, aiding in the overall discussion about their chemical compositions and practical applications.
Prelims to Polymers
Polymers play a crucial role in our daily lives and in various industries. Understanding polymers is essential for academic and practical applications. They are used in everyday products, ranging from packaging materials to medical devices. Knowing their chemical composition allows us to tailor properties for specific uses, improving performance and sustainability. This section provides foundational knowledge that supports further exploration of polymer chemistry.
Definition and Characteristics
Polymers are large molecules composed of repeating structural units called monomers. These monomers are connected through chemical bonds, creating long chains. The most common form of bond in polymers is covalent bonding. Polymers can be either natural, such as proteins and cellulose, or synthetic, like polyethylene and polymethyl methacrylate.
Their characteristics vary significantly based on the type of monomers involved and the nature of the bonding. For instance, some polymers are flexible, while others are rigid. Their melting points, solubility, and elasticity also differ. This diversity in characteristics makes polymers valuable in many applications, including textiles, plastics, and coatings.
Historical Perspective
The study of polymers dates back to the early 19th century. Early discoveries involved natural polymers, such as rubber and cellulose. In the 1900s, scientists began to synthesize new types of polymers, a significant advancement in material science. Notably, Bakelite, created by Leo Baekeland in 1907, became the first fully synthetic polymer. This marked a turning point in industrial chemistry, leading to the rapid development of a variety of synthetic materials.
Today, polymers are at the forefront of research and innovation. A wide range of industries relies on polymers for their products, which includes automotive and medical sectors. As the understanding of polymer chemistry evolves, so does the potential for new applications and improvements in existing materials. This historical lens helps illuminate the importance of polymers in modern science and industry.
Basic Chemical Structure of Polymers
The basic chemical structure of polymers is crucial to understanding their properties and behaviors. Polymers are macromolecules composed of repeating units known as monomers, which are connected through various types of chemical bonds. This structural foundation is what provides polymers their unique characteristics, distinguishing them from simpler molecules. The study of polymer structure encompasses how the arrangement of these monomers affects both the physical properties and the functionalities of the materials. Understanding these concepts is essential for anyone involved in polymer chemistry, material science, or related fields.
Monomers and their Role
Monomers serve as the building blocks for all polymers. These small molecules can vary widely in nature, from simple hydrocarbons to more complex organic compounds. Each type of monomer brings specific traits to the final polymer. For instance, the arrangement and type of monomers determine the polymer's thermal stability, mechanical strength, and resistance to chemical degradation.
Common monomers include ethylene for polyethylene and styrene for polystyrene. Each of these monomers, when polymerized, creates distinct materials with various applications in countless industries. The ability to manipulate monomer selection allows chemists to design polymers tailored to specific needs.
Polymer Chains and Configuration
The arrangement of monomers within the polymer chain is a significant factor in influencing polymer characteristics. Chains can be linear, branched, or cross-linked. Linear polymers consist of long, straight chains where monomers link directly in a sequence. In contrast, branched polymers have side chains extending from the main backbone, which can alter their density and strength.
Cross-linked polymers feature bonds between separate polymer chains, creating a three-dimensional network. This configuration typically results in materials that are tougher and more resistant to changes in temperature and solvent exposure. For example, vulcanized rubber has a cross-linked structure that greatly enhances its durability compared to standard rubber.
Types of Polymer Bonds
The nature of the bonds between monomers plays a crucial role in defining the polymer's properties. There are three primary types of bonds found in polymers: covalent bonds, ionic bonds, and hydrogen bonds.
- Covalent Bonds: Strong bonds formed when atoms share electrons. They create robust linkages between monomers, resulting in stable polymer chains.
- Ionic Bonds: Weaker bonds that can occur between charged monomers. These are less common in synthetic polymers but can be found in natural ones, such as in certain proteins.
- Hydrogen Bonds: A type of non-covalent interaction that can provide additional stability to polymer structures. They are particularly important in biopolymers like proteins and nucleic acids.
Understanding these bonds helps predict how polymers will behave under different environmental conditions, guiding their use in applications ranging from everyday materials to specialized industrial products.
The structure of polymers, from monomer types to chain configurations and bonding, directly influences their properties and applications. A thorough knowledge of these elements is vital in materials science, engineering, and technology.
Types of Polymers Based on Composition
Understanding the types of polymers based on their composition is crucial in the field of polymer chemistry. This classification allows for a better grasp of their properties, how they are synthesized, and their applications in various industries. The significance arises from both natural and synthetic origins, each type exhibiting distinct characteristics and functionalities. This knowledge benefits researchers, educators, and professionals alike, presenting insights into effective applications and innovations.
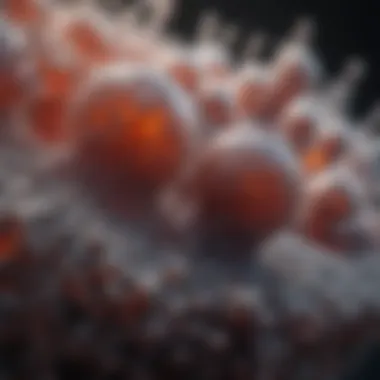
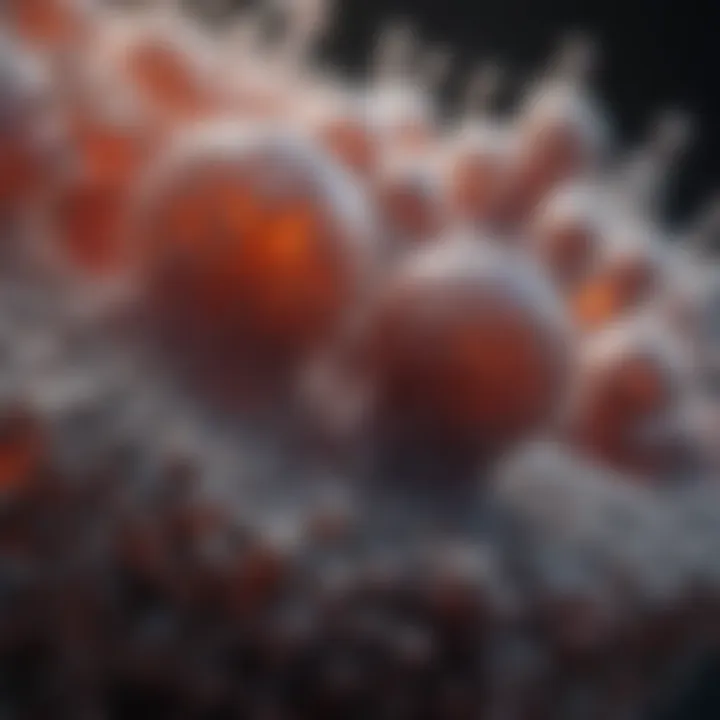
Natural Polymers
Natural polymers are derived from biological sources. They play essential roles in nature, offering unique properties that have been utilized by humans for centuries.
Cellulose
Cellulose is a primary component of plant cell walls. Its structure consists of long chains of glucose molecules, linked by beta-1,4-glycosidic bonds. A key characteristic of cellulose is its rigidity and strength, making it a significant structural material in plants. In this article, it is important to highlight cellulose for its biodegradability and abundance. Cellulose fibers can be utilized in various applications such as paper and textiles. However, its insolubility can limit its use in some chemical processes.
Proteins
Proteins are polymers made from amino acids and play vital roles in biological functions. The specific aspect of proteins that stands out is their diverse functionalities, ranging from enzymatic catalysis to structural support in cells. Their unique characteristic is the ability to fold into complex three-dimensional structures. This property allows proteins to perform highly specialized functions. In the context of this article, their importance stems from their application in bioengineering and pharmaceuticals, although the complexity of protein synthesis can pose challenges.
Nucleic Acids
Nucleic acids, composed of nucleotide monomers, are another essential category of natural polymers. They are integral to genetic information storage and transfer, making them fundamental to all living organisms. A notable characteristic is their helical structure, which facilitates the coding of genetic data. The uniqueness of nucleic acids lies in their role in biotechnology and gene therapy. However, their susceptibility to degradation can be a disadvantage in some applications.
Synthetic Polymers
Synthetic polymers have revolutionized numerous industries with their customizable properties and wide range of applications. These polymers are created through artificial processes, providing options that nature does not offer.
Polyethylene
Polyethylene is perhaps the most common synthetic polymer, widely used in packaging and containers. It is formed from the polymerization of ethylene monomers and is characterized by its flexibility and low density. Polyethylene's significance in this article is its prevalence in consumer goods and its recyclability potential. However, its environmental impact due to plastic waste is a considerable downside.
PVC
Polyvinyl chloride, commonly known as PVC, is synthetically produced for various construction and healthcare applications. The key feature of PVC is its durability and resistance to environmental degradation. PVC's widespread use in pipes, windows, and medical devices highlights its benefit as a versatile material. Nonetheless, concerns regarding the toxicity of some additives and its recycling challenges pose notable disadvantages.
Polyesters
Polyesters are a type of synthetic polymer characterized by the presence of ester functional groups in their long-chain structure. Their primary benefit lies in their versatility, used in textiles, films, and some biodegradable plastics. Polyesters are notable for their aesthetic qualities and durability. However, the synthesis process can have a substantial ecological footprint, which is an important consideration in contemporary polymer research.
Chemical Composition of Natural Polymers
Natural polymers are fundamental to life and the environment. Their chemical composition directly influences their functions and properties. These polymers are primarily made of bio-based materials and play essential roles in biological systems. Studying their composition provides insights into their mechanisms and potential applications in various fields, including medicine and materials science. Understanding how different elements and structures contribute to the properties of natural polymers can lead to innovations in sustainable alternatives to synthetic materials.
Carbohydrates
Carbohydrates are one of the most common classes of natural polymers. They are composed of carbon, hydrogen, and oxygen, usually in a ratio of 1:2:1. Their basic units are monosaccharides, such as glucose and fructose, which combine to form larger polymeric structures like starch, cellulose, and glycogen.
Importance of Carbohydrates:
- Energy Storage: Starch and glycogen serve as energy reserves in plants and animals, respectively. This storage capacity is vital for maintaining metabolic functions.
- Structural Functions: Cellulose provides rigidity in plant cell walls, contributing to their structural integrity. This characteristic also makes cellulose crucial for paper and textile production.
- Functional Diversity: Carbohydrates are often linked to proteins and lipids, forming glycoproteins and glycolipids, which are important in cellular recognition and signaling processes.
Proteins
Proteins are polymers made from amino acids, which are the building blocks of life. Each protein consists of one or more chains of amino acids linked by peptide bonds. The sequence of amino acids determines a protein's specific shape and function.
Key Attributes of Proteins:
- Diversity of Functions: Proteins serve various roles, including catalysts (enzymes), structural components (collagen), and transporters (hemoglobin).
- Composition Variability: Proteins can contain different functional groups, allowing for a wide array of biochemical activities and interactions. Common elements include carbon, hydrogen, oxygen, nitrogen, and sometimes sulfur.
- Thermodynamic Stability: The three-dimensional structure of proteins is maintained by various interactions, including hydrogen bonds, ionic bonds, and hydrophobic interactions. This stability is crucial for their biological function.
Nucleic Acids
Nucleic acids, such as DNA and RNA, are polymers made from nucleotide monomers. Each nucleotide comprises a sugar, a phosphate group, and a nitrogenous base. The sequence of these nucleotides encodes genetic information.
Characteristics of Nucleic Acids:
- Information Storage: DNA serves as the hereditary material in almost all organisms, encoding the instructions for development and function.
- Protein Synthesis Role: RNA is essential in translating genetic information into proteins, playing a pivotal role in the central dogma of molecular biology.
- Structural Variability: The structure of nucleic acids can vary. For example, DNA is typically double-stranded, while RNA is usually single-stranded. This structural diversity impacts their roles in the cell.
Understanding the chemical composition of natural polymers enhances insights into their ecological and biological significance, leading to innovative applications and sustainable practices.
Chemical Composition of Synthetic Polymers
Synthetic polymers are fundamental materials in various industries. Understanding their chemical composition is vital. It helps to discern how these materials can be manipulated for specific uses. While natural polymers are formed from biological processes, synthetic polymers result from human intervention through chemical reactions.
The importance of synthetic polymers lies in their versatility. They can be engineered to exhibit specific characteristics tailored to fit diverse applications. This ranges from durability and flexibility to resistance to chemicals and heat. Recognizing the chemical components involved is essential to optimize these properties and enhance polymer performance.
Addition Polymers
Addition polymers are a class formed by the direct linking of monomers with unsaturated bonds. This process typically involves the breaking of double bonds in the monomers, allowing them to join together in long chains. A common example of addition polymers is polyethylene. Polyethylene is utilized extensively in packaging due to its lightweight and resilient nature.
The chemical process of forming addition polymers is characterized by a few notable features:
- Chain Growth: The reaction continues as long as there are monomers available. This leads to very long polymer chains, which contribute to the mechanical strength of the material.
- Initiators: Usually, a free radical or an ion is needed to start the reaction. This initiator can be a chemical compound that generates radicals when subjected to heat or light.
- Termination: The process stops when two growing polymer chains combine or when an active chain reacts with an impurity.
The ability to control the molecular weight of addition polymers is significant for customizing their physical properties, making these polymers exceedingly important in industrial applications.
Condensation Polymers
Condensation polymers form through a different mechanism. These involve reactions that yield small molecules, often water, as by-products. Typically, these polymers require two different types of monomers, each with at least two functional groups. A well-known example is polyester, widely used in textiles and films.
Key aspects of condensation polymers include:
- Step Growth: Unlike chain growth, the formation of the polymer occurs in multiple steps. This results in a polymer with a more complex structure and potential for varied properties.
- Diverse Functional Groups: The presence of different functional groups allows for the formation of intricate branching within the polymer, influencing the final material properties.
- Cross-Linking: This can occur between chains, making the resulting material more robust and heat resistant.
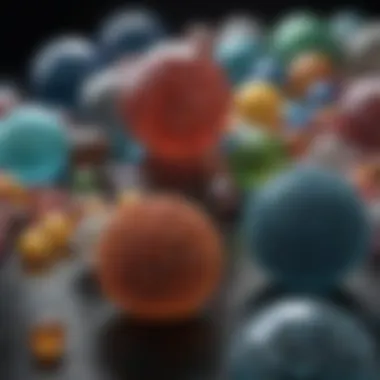
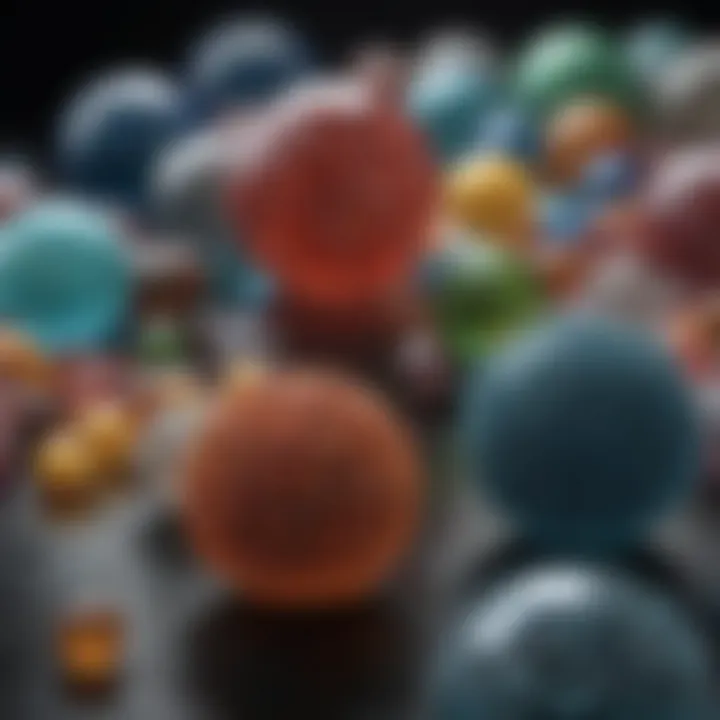
The chemical composition of synthetic polymers provides the basis for understanding how these materials function in their applied contexts. Through careful manipulation of their chemical structure, scientists and engineers can create innovative solutions that cater to the needs of various industries.
Elements in Polymer Chemistry
The study of polymer chemistry heavily relies on understanding the specific elements that constitute these materials. The fundamental elements, primarily carbon, hydrogen, oxygen, and nitrogen, play crucial roles in defining the properties, behaviors, and functional capabilities of polymers. Beyond these core elements, additional elements such as sulfur and phosphorus are also significant. Given their diverse range of applications in industry and research, comprehending these elements is essential.
Carbon
Carbon is the backbone of polymer chemistry. Its ability to form long chains and stable bonds with other carbon atoms leads to the creation of diverse polymer structures. Carbon atoms can bond in various configurations, such as linear, branched, or cross-linked forms, which influence the physical traits and chemical properties of the polymer. For instance, polymers like polyethylene derive unique properties from the arrangement of their carbon chains.
Hydrogen
Hydrogen, while a simple element, plays a vital role in polymers. It typically bonds with carbon, filling the valence shell and stabilizing the structure. The number of hydrogen atoms attached to a carbon chain can influence the polymer's density and flexibility. High hydrogen content generally leads to lower density, making the resulting polymer more pliable. This characteristic is particularly observed in polyolefins, widely used in packaging materials and various consumer products.
Oxygen and Nitrogen
Oxygen and nitrogen are vital in enhancing the functionality of polymers. Oxygen, present in many functional groups such as hydroxyl and carboxyl, affects a polymer's reactivity and stability. Nitrogen is often found in amine groups, contributing to polarity and enabling hydrogen bonding. This makes polymers containing these elements more water-soluble and adaptable for specific applications, such as in drug delivery systems or biodegradable plastics. Moreover, the presence of these elements can significantly alter mechanical properties, thermal stability, and chemical resistance.
Other Elements (Sulfur, Phosphorus, etc.)
Other elements like sulfur and phosphorus also contribute to polymer chemistry, albeit in less common ways. Sulfur, for example, is often used in vulcanization of rubber, which enhances durability and elasticity. Phosphorus compounds are critical in producing flame-retardant materials, offering safety and protection in many applications. Furthermore, transition metals sometimes participate as catalysts in polymerization processes, creating unique material profiles depending on their oxidation states.
The intricate dance of elements in polymer chemistry allows for the tailoring of polymer properties, making it essential for scientists and engineers to understand these compositions.
Understanding the roles of these elements is critical for researchers, students, and professionals alike. This knowledge not only underpins current applications but also guides innovations in polymer science, ensuring that advancements in the field are both practical and revolutionary.
Functional Groups in Polymers
Functional groups play a significant role in defining the chemical behavior of polymers. These groups influence the physical and chemical properties of various polymeric materials. Understanding functional groups is vital for researchers and professionals in polymer chemistry, as it allows for the modulation of polymer characteristics according to specific applications.
Functional groups can affect solubility, reactivity, thermodynamic properties, and even mechanical strength of polymers.
Hydroxyl Groups
Hydroxyl groups (-OH) are common in many natural and synthetic polymers. They increase the polarity of the polymer, which enhances solubility in polar solvents. Hydroxyl groups can also participate in hydrogen bonding, affecting the overall physical properties. Moreover, they are crucial in chemical reactions, making it easier to modify polymers or create copolymers. For instance, polyvinyl alcohol contains hydroxyl groups, making it hydrophilic, thus broadening its usability in biomedical applications and as a packaging material.
The presence of hydroxyl groups is also significant in the context of the properties of wood, cellulose, and other natural materials.
Carboxyl Groups
Carboxyl groups (-COOH) are characterized by both a carbonyl and a hydroxyl group. Their presence in a polymer chain introduces acidity, which can affect the polymer's reactivity. Polymers with carboxyl functional groups tend to have higher melting points and better thermal stability than those without. They enhance interaction with other materials, which makes them suitable for various applications. For instance, Polyacrylic acid, a polymer containing carboxyl groups, is widely used in absorbent products and as a thickening agent in paints and coatings. The carboxyl groups facilitate the formation of gels and other structures that are valuable in many contexts.
Amine Groups
Amine groups (-N) are characterized by their nitrogen atom connected to one or more carbon atoms. These groups impart basicity to polymers, influencing their reactivity. Polymers containing amine groups can form strong bonds with other molecules, which is beneficial for adhesives and coatings. For instance, polyamine can be used in various formulations, including water treatment and as a flocculant. Furthermore, amine functional groups help improve the compatibility of polymers with other compounds, enhancing their performance in composite materials. Their versatility and reactivity make them vital components in the toolkit of polymer chemists.
The knowledge of functional groups allows chemists to design polymers tailored for specific applications, optimizing their performance in desired scenarios.
Polymerization Processes
Polymerization processes are fundamental in the field of polymer chemistry. These processes dictate how small molecules, known as monomers, combine to form larger, complex polymer structures. Understanding polymerization is crucial for several reasons. Firstly, the resulting polymer's properties—such as tensile strength, thermal stability, and elasticity—are intrinsically linked to the type of polymerization carried out. Secondly, different polymerization methods allow for varying degrees of control over the polymer's molecular weight and distribution, which in turn affects performance in practical applications. Lastly, recognizing the mechanisms involved in polymer formation is critical for tailoring materials to meet specific needs in various industries, including packaging, construction, and biotechnology.
Addition Polymerization
Addition polymerization occurs when monomers with unsaturated bonds, usually double bonds, react to form a polymer without the release of any small molecules. This process generally involves three key stages: initiation, propagation, and termination.
- Initiation: This stage requires an initiator, a compound that starts the reaction. Common initiators include peroxides and azo compounds, which decompose to produce free radicals.
- Propagation: Free radicals generated during initiation react with the monomers, creating new radicals and adding them to the growing polymer chain. This step can rapidly increase the length of the polymer chains.
- Termination: Polymer chains grow until they encounter another radical, leading to a recombination or disproportionation reaction. This halts the polymerization process and results in high-molecular-weight polymers.
Addition polymerization is widely used in producing materials like polyethylene and polystyrene, which play significant roles in everyday products such as containers, packaging films, and insulation materials.
Condensation Polymerization
Condensation polymerization involves the reaction of monomers containing two or more functional groups. Unlike addition polymerization, this method results in the loss of small molecules, often water, during the reaction. This process also has distinct stages, which include:
- Step Growth: In this mode of polymerization, any two monomers can react regardless of chain length, gradually forming larger polymers over time. This can lead to varying chain lengths and degrees of polymerization.
- Polymer Growth: As the reaction proceeds, the availability of functional groups decreases, slowing down the reaction. Eventually, most of the functional groups are consumed, ceasing further polymer growth.
- Byproduct Formation: The loss of small molecules, such as water or alcohols, is characteristic of this polymerization type. For instance, in the synthesis of polyesters, the reaction between carboxylic acids and alcohols leads to the formation of ester linkages and the concurrent release of water.
Condensation polymerization is essential in producing materials like nylon and polyesters. These polymers are often utilized in textiles, engineering plastics, and coatings, highlighting their significance in both consumer products and industrial applications.
Important Note: Understanding the differences between addition and condensation polymerization can help researchers and developers choose the right method for specific applications, optimizing material performance.
Through these polymerization techniques, scientists can create diverse polymeric materials tailored for various functions, emphasizing the importance of the polymerization process in the broader context of polymer chemistry.
Characterization of Polymer Composition
Characterization of polymer composition is crucial in understanding the properties and behavior of polymers. This section will discuss several important techniques that serve to reveal the intricate chemical structures and arrangements within polymer materials. By employing these methods, researchers and professionals can identify the composition and purity of materials, which is essential for quality control and development of new polymers. Understanding how polymers are characterized also offers insights into their applications in various fields such as medicine, engineering, and environmental science.
Spectroscopic Techniques
Spectroscopic techniques are fundamental in polymer characterization. They provide valuable information about molecular structure through interactions between light and matter. The three main forms of spectroscopy used in this domain are NMR spectroscopy, IR spectroscopy, and UV-Vis spectroscopy.
NMR Spectroscopy
Nuclear Magnetic Resonance (NMR) spectroscopy is often employed for analyzing the internal structure of polymers. The main aspect of NMR is its ability to provide detailed information about the arrangement of atoms in a molecule, which directly affects polymer properties. A key characteristic of NMR is its non-destructive nature, allowing samples to remain intact for further testing.
The unique feature of NMR is its capacity to distinguish between different chemical environments, which makes it particularly good for studying copolymers that consist of different monomer units. However, it requires high concentrations of the sample and may need expensive equipment, which can be a drawback for some applications.
IR Spectroscopy
Infrared (IR) spectroscopy measures the absorption of infrared light, which causes molecular vibrations. This technique helps in identifying functional groups within polymers, making it a popular choice for characterization. The primary advantage of IR spectroscopy is its simplicity and speed, allowing for rapid assessment of a sample’s chemical structure.
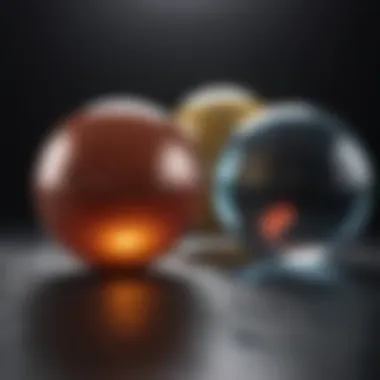
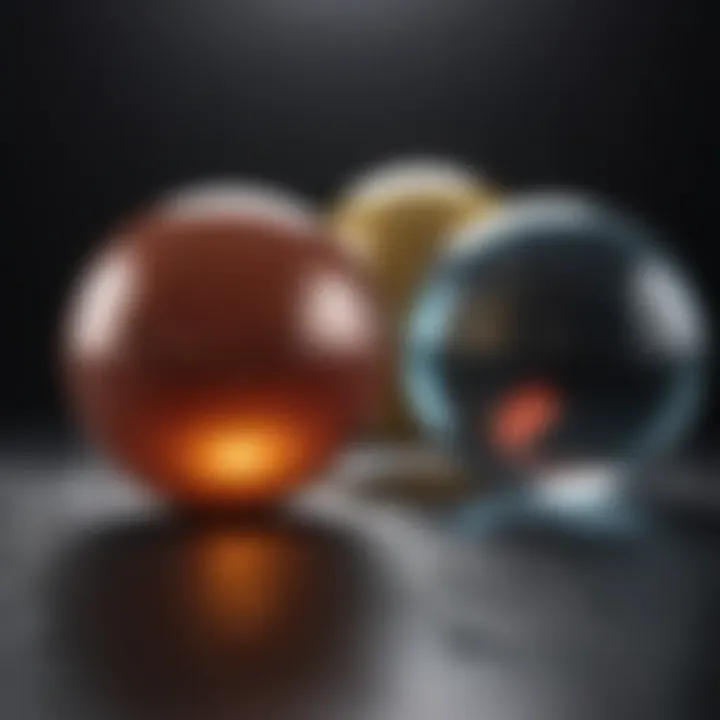
IR spectroscopy uniquely identifies the bond types present by detecting specific wavelengths for different functional groups. A disadvantage is that IR cannot provide detailed structural information about the polymer's exact arrangement, which may be necessary for some analyses.
UV-Vis Spectroscopy
Ultraviolet-Visible (UV-Vis) spectroscopy involves the absorption of UV or visible light by a polymer. This process gives insights into electronic transitions in the molecule. A significant characteristic of UV-Vis is its ability to analyze colored solutions, making it a beneficial choice for examining polymers that experience color changes with chemical reactions.
The unique feature of this technique lies in its sensitivity to conjugated systems, which is essential for studying polymers with delocalized electrons. However, it often requires pre-concentration of samples to be effective, which can complicate analysis in some situations.
Chromatographic Techniques
Chromatographic techniques are vital for separating and analyzing the components of polymer mixtures. These methods provide insights into molecular weights and distributions, essential for understanding polymer behavior in applications.
Gel Permeation Chromatography
Gel Permeation Chromatography (GPC) is a widely used technique for determining the molecular weight distribution of polymers. The main aspect of GPC involves separating molecules based on their size as they pass through a porous gel material. GPC is advantageous because it provides a clear profile of the molecular weights present in a sample, allowing researchers to assess its properties accurately.
A unique feature of GPC is its efficiency in analyzing high molar mass samples, which is often challenging with other methods. However, it usually requires calibration with standard samples, which may introduce errors if the standards are not representative of the polymer being tested.
Size Exclusion Chromatography
Size Exclusion Chromatography (SEC) shares similarities with GPC where it separates molecules based on size. This technique is particularly valuable for determining both hydrodynamic volume and molecular weights. Its key characteristic lies in its ability to provide information about the overall size and shape of polymer chains.
A notable feature of SEC is that it does not require specific interactions with the stationary phase, making it suitable for a wide range of polymer types. Nevertheless, it may not provide sufficient details about the chemical structure of the polymer and sometimes lacks resolution in separating closely sized components.
Understanding these characterization techniques is essential for advancing polymer research. By leveraging these analytical tools, scientists can develop polymers with desired properties and functions, paving the way for innovative applications in various industries.
Applications of Polymer Chemistry
The field of polymer chemistry plays a crucial role in various industries. Understanding the chemical composition of polymers allows scientists and engineers to design materials with specific properties for targeted applications. The integration of polymers into everyday products highlights their versatility and importance. These materials can be tailored to enhance strength, flexibility, and resistance to environmental factors, making them essential in the contemporary world.
Industrial Applications
Polymers are integral in multiple industrial sectors, including packaging, construction, automotive, and electronics. For example, polyethylene, widely used for plastic bags, offers a balance of strength and lightness. In the construction industry, polymers like polyvinyl chloride (PVC) serve as essential materials for pipes and flooring due to their durability and chemical resistance.
The automotive sector utilizes polymers for lightweight components, aiding in fuel efficiency. Additionally, advances in polymer chemistry are leading to the development of smart materials. These materials can respond to environmental stimuli, offering exciting possibilities for future industrial applications.
"Polymers redefine the boundaries of what materials can achieve, influencing both functionality and design across industries."
Biomedical Applications
In the biomedical field, polymers serve as a foundation for numerous applications, ranging from drug delivery systems to implants. Biocompatible polymers like polylactic acid have transformed the way medical devices are designed. They can be safely used within the human body, minimizing risks of rejection or adverse reactions.
Moreover, hydrogels, which are water-swellable polymers, are utilized in tissue engineering. These materials mimic natural tissue properties, enabling cell growth and repair. Polymers are also essential in creating controlled-release drug delivery systems, improving patient compliance and therapeutic effectiveness.
Environmental Considerations
The environmental impact of polymer usage is gaining attention. Although polymers provide convenience and utility, their persistence in the environment raises concerns. The challenges of plastic pollution have prompted researchers to explore biodegradable alternatives. Materials like polylactic acid are being developed to reduce long-term waste in landfills.
Moreover, recycling technologies for polymers are advancing. Innovations in circular economy practices aim to repurpose waste back into the manufacturing cycle. The focus on sustainable practices is crucial as we seek to mitigate adverse effects on ecosystems and promote greener production methods.
Polymers are essential in modern life, with applications spanning across multiple fields. Understanding their chemical composition and characteristics is vital for advancing technology while balancing environmental responsibilities.
Future Directions in Polymer Research
Understanding future directions in polymer research is essential for advancing the field of polymer chemistry. As technology evolves, the demand for new materials with enhanced properties increases. This section will explore innovations and sustainable development approaches that can shape the future of polymers.
Innovations in Polymer Chemistry
Innovations in polymer chemistry are driven by the need for materials that meet new challenges. One of the key areas of focus is the development of functionalized polymers. These materials exhibit specific properties that can be tailored for various applications. For example, smart polymers that respond to environmental stimuli like temperature or pH are gaining attention. This responsiveness makes them suitable for use in drug delivery systems and sensors.
Furthermore, advanced polymer processing techniques, such as 3D printing, are revolutionizing the manufacturing landscape. This technology allows for precise control over polymer structures, resulting in the creation of complex geometries and reduced waste. These innovations enhance the ability to design materials with specific mechanical and thermal properties, ultimately leading to improved performance in real-world applications.
In addition, the integration of nanotechnology into polymer science opens new possibilities. Nanocomposites, which combine polymers with nanoparticles, can achieve superior strength, thermal stability, and barrier properties. Research is ongoing to optimize these materials for use in electronics, automotive, and aerospace applications.
Sustainable Polymer Development
Sustainability is an increasingly critical consideration in polymer research. The environmental impact of conventional synthetic polymers has prompted scientists to explore biodegradable alternatives. Developing polymers from renewable resources not only reduces reliance on fossil fuels but also helps in addressing waste management issues.
Some promising approaches include using bio-based feedstocks, such as cornstarch or sugarcane, to create polymers like polylactic acid (PLA). These materials decompose into non-toxic byproducts, making them more environmentally friendly compared to traditional plastics.
Moreover, recycling advancements are crucial in reducing the ecological footprint of polymers. Innovative methods are being researched to improve the recyclability of polymer products. For instance, reversible polymerization techniques may allow for the breakdown of polymers back into their monomers, facilitating recycling processes.
"The future of polymer chemistry lies not only in innovation in material properties but also in our ability to create sustainable solutions that address global challenges."
Closure
The examination of polymer chemistry in this article reveals the vital role of understanding chemical composition in the real world. The intricate details surrounding polymer structures and their formation processes underscore their importance, particularly as they relate to both natural and synthetic materials. The array of polymers studied demonstrates their diverse applications, from industrial uses to biomedical innovations.
Importance of Chemical Composition
By focusing on specific elements such as carbon, hydrogen, oxygen, and nitrogen, it becomes clear how these basic building blocks contribute to the unique properties of various polymers. Understanding the chemical composition allows researchers and professionals to predict behaviors like thermal stability, elasticity, and durability. This insight is beneficial not just academically but also in practical applications where specific characteristics of materials are crucial.
Benefits of Comprehensive Knowledge
- Material Selection: A deeper understanding helps in selecting appropriate materials for specific applications. Whether in construction, textiles, or pharmaceuticals, knowing the composition aids in choosing polymers that meet performance criteria.
- Innovation in Applications: Recognizing how functional groups affect the properties of polymers can drive innovation. For example, developing new coatings that resist corrosion or creating biocompatible materials for medical devices often relies on detailed knowledge of functional chemistry.
- Sustainability Considerations: In current research, the emphasis on sustainable development has grown, making the chemical composition more relevant. As the industry drives toward greener alternatives, the ability to understand and modify existing polymers is essential in creating more environmentally friendly products.
"The future of polymer chemistry rests on our understanding of its fundamental chemical composition."
Final Considerations
The future of materials science and polymer development is promising, built on a robust foundation of knowledge regarding chemical composition.