Assessing Radiation Blocking Technologies and Their Impact
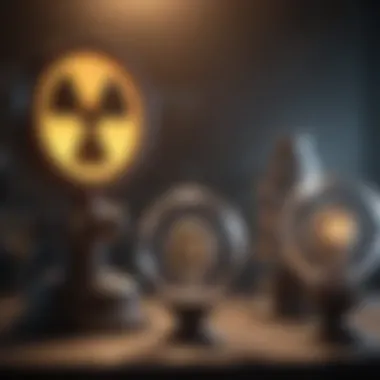
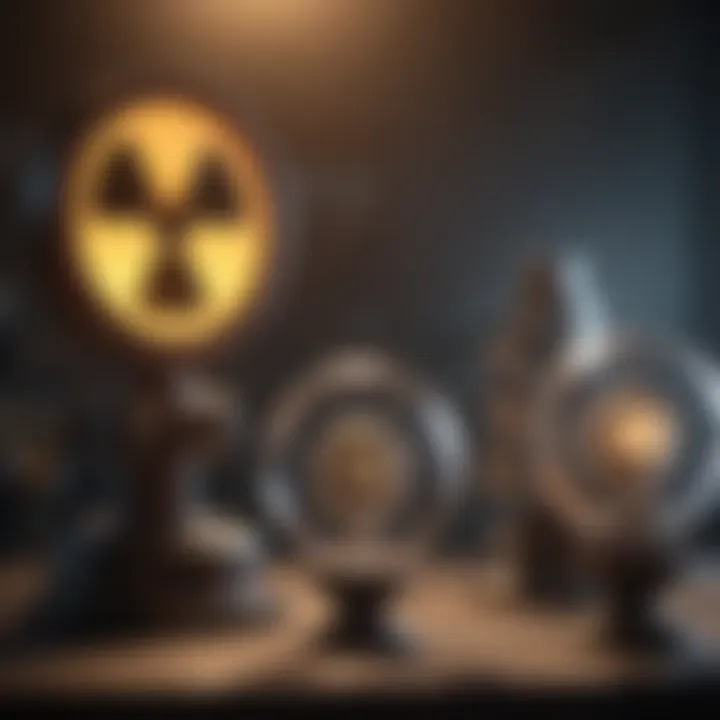
Intro
The necessity for safety in a world where technology is advancing faster than the eye can see seems to be more pressing than ever. As our reliance on various gadgets grows, so too does our exposure to radiation. From the smartphones in our pockets to the Wi-Fi routers in our homes, invisible waves of radiation permeate our daily lives. Given this, understanding the technology behind radiation blocking is essential.
Radiation can be broadly classified into ionizing and non-ionizing types, with each posing different health risks. Ionizing radiation, like X-rays, can cause cellular damage that may lead to conditions such as cancer. On the flip side, non-ionizing radiation, found in everyday devices, is often considered less harmful but has its share of concerns. Thus, an exploration into the efficacy of radiation blocking technologies becomes crucial.
The goal of this article is to dissect the various methods and materials employed in radiation shielding, evaluating their effectiveness and application in everyday environments and specialized industries. With advancements in research continually shaping the landscape, it's pertinent to remain informed about the innovations that aim to protect public health and the environment.
Research Overview
Summary of Key Findings
Radiation blocking technologies are not all created equal. The efficacy of different materials varies significantly based on several factors, including the type of radiation being blocked and the material’s thickness and density.
- Metal Shields: Materials like lead, steel, and aluminum have been used traditionally for shielding against ionizing radiation. Lead, in particular, is well-known for its high level of protection, although concerns about toxicity and environmental impact are rising.
- Polymer-Based Solutions: Innovations in polymer composites have led to new materials that are lightweight and effective against certain types of radiation, particularly in space applications.
- Natural Materials: Research is also revealing the potential of more eco-friendly alternatives, such as concrete and other earth-based substances, in shielding applications.
These findings signal crucial conversations in the scientific community, as researchers aim to balance effectiveness with sustainability in radiation shielding technologies.
Relevance to Current Scientific Discussions
In recent years, discussions have surged in scientific circles regarding the need for stricter regulations on radiation exposure in various industries, including healthcare and technology. Increasing screening for radiation exposure in workers handling radioactive materials is slated to become standard practice, a move the scientific community lauds.
Thus, innovations in radiation blocking technologies are not just a point of interest but a necessity to safeguard people's health as global standards evolve. Scientists are urged to consider not only the shielding materials' properties but also their environmental implications, resulting in a paradigm shift in how we understand radiation safety.
"The protection against radiation is no longer just about shielding; it also encompasses the materials' sustainable production and disposal."
The relationship between evolving scientific findings, public safety, and environmental stewardship can't be overstated. As industries look to comply with new regulations, understanding the efficacy of radiation blocking technologies will remain paramount.
Understanding Radiation
Understanding radiation is crucial to grasp the implications and significance of the technologies designed to block or mitigate exposure. In this segment, we will break down the concept of radiation itself, the various forms it can take, and the potential health risks associated with it. This foundational knowledge sets the stage for understanding how effective radiation blocking technologies can be in protecting human health and the environment.
Definition and Types
Electromagnetic Radiation
Electromagnetic radiation consists of waves of energy produced by the movement of charged particles. It includes a broad spectrum ranging from gamma rays to radio waves. One key characteristic of this type is its ability to travel at the speed of light, allowing it to cover vast distances in a short amount of time. Electromagnetic radiation is widely recognized in several fields, like telecommunications and medical imaging, due to its useful applications. However, its unique feature—varying energy levels—means that while some segments can be harnessed safely, others, like X-rays and gamma rays, carry a risk of exposure that necessitates protective measures. The advantages of understanding electromagnetic radiation lie in its predictive power and utility in advancing technologies; yet, the disadvantages arise when one considers the potential harm from unmitigated exposure.
Particle Radiation
Unlike electromagnetic radiation, particle radiation involves the emission of particles such as alpha particles, beta particles, and neutrons. These particles have mass and can transfer significant energy to matter. A key characteristic of particle radiation is its ionizing nature, which can damage human cells and DNA. This type of radiation is particularly beneficial in the context of nuclear energy and certain medical treatments where targeted destruction of cells is desired. However, the unique feature of particle radiation is its capacity for harm, especially during unregulated exposure. Hence, while it is leveraged in beneficial ways, understanding its dangers is essential for promoting safer applications.
Natural vs Artificial Sources
Radiation can be broadly categorized into natural and artificial sources. Natural radiation comes from cosmic rays and rocks, while artificial sources originate from human-made technologies, like nuclear power plants or medical x-ray machines. The key characteristic of natural sources lies in their ubiquitous presence in our environment, effectively meaning that everyone is exposed to some level of it. This aspect plays into the idea that we need to understand our baseline exposure levels thoroughly when evaluating benefits and risks. On the other hand, artificial sources often come with safety regulations, highlighting the importance of developing effective shielding methods. The unique feature of distinguishing between these sources lies in the knowledge it brings to effectively managing risk versus benefit and ensuring public safety without triggering undue fear.
Health Risks Associated with Radiation Exposure
Acute Effects
Acute effects of radiation exposure occur almost immediately, presenting themselves in symptoms such as nausea, vomiting, and skin burns. These effects happen when high doses are absorbed in a short time. Understanding acute effects is key to immediate interventions and medical responses in scenarios like nuclear accidents. The unique feature here is the rapid onset of symptoms, which underscores the need for swift action in emergencies. While serious, recognizing these acute outcomes helps in educating people about safety precautions, making it a significant focus in discussions on radiation exposure risks.
Long-Term Health Consequences
Long-term health consequences of radiation exposure manifest years or even decades later and can include cancers, organ dysfunction, and genetic mutations. These consequences focus on the cumulative effects of low-dose exposure over time, making it a complex area to study and manage. The key characteristic to note is that these effects often arise from what might seem like innocuous levels of exposure, which can lead to misinformed public perception regarding safety. Awareness of these long-term risks plays an essential role in policy-making, shaping regulations, and advocating for more robust shielding technologies.
Vulnerable Populations
Certain populations, including children, the elderly, and individuals with preexisting health conditions, can be more severely impacted by radiation exposure. Understanding the specific risks they face is essential for creating targeted protective measures. By focusing on vulnerable populations, efforts can be channeled toward enhancing the efficacy of radiation shielding technologies. The distinctive feature here is the heightened sensitivity these groups have regarding radiation, which amplifies the need for rigorous regulatory oversight and tailored safety practices.
The depth of understanding radiation fosters the development of effective strategies and technologies to mitigate its harmful effects on health and well-being.
Principles of Radiation Blocking
Understanding the principles of radiation blocking is crucial for grasping how we can effectively protect ourselves from harmful exposures. This section delves into the fundamental mechanisms through which radiation is attenuated and the key parameters that affect the efficiency of this process. By exploring the underlying concepts, we can appreciate the significance of material choices, design strategies, and their applications in real-world scenarios.
Mechanisms of Attenuation
The term attenuation refers to the reduction in the intensity of radiation as it passes through a material. The primary mechanisms responsible for this reduction are absorption, scattering, and reflection.
Absorption
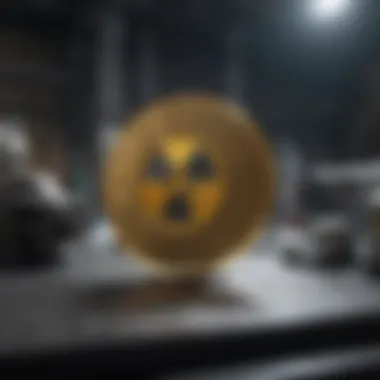
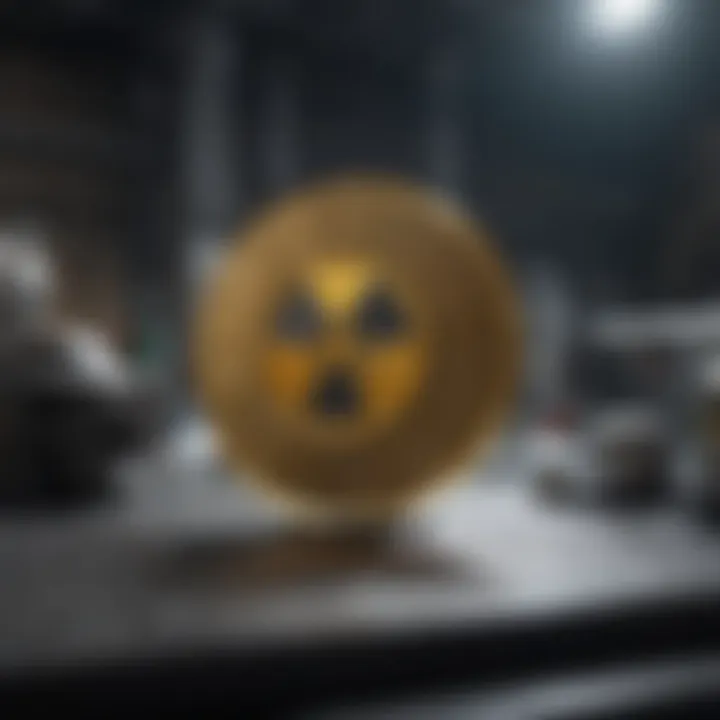
Absorption is the process where radiation energy is taken up by a material, converting it into another form of energy, typically heat. One significant characteristic of absorption is that it is effective across a wide range of radiation types, including electromagnetic and particle radiation. This versatility makes absorption a valuable strategy for shielding against radiation.
The unique feature of absorption lies in its ability to mitigate radiation exposure without recycling the energy back into the environment. This fundamental trait makes it particularly advantageous in settings where radiation exposure is frequent, such as medical facilities. However, absorbed energy can lead to increased material temperatures, which can sometimes limit the material's effectiveness over time, potentially creating safety concerns.
"Absorption is not just a barrier; it’s a transformative process that changes how radiation interacts with our surroundings."
Scattering
Scattering involves the deflection of radiation particles as they collide with atoms in a shielding material. Unlike absorption, which removes energy from the radiation, scattering alters its direction, dispersing it away from a designated area. One vital aspect of scattering is its dependency on the size and composition of the atoms within the material. This feature allows it to be tailored to block specific types of radiation.
For example, high atomic number materials can be particularly effective at scattering ionizing radiation. However, the downside to scattering is that, in some cases, it can lead to an incidental increase in radiation exposure if the scattered particles redirect toward vulnerable areas. Therefore, while scattering can enhance a passive shielding design, it must be used judiciously, considering the layout and potential pathways of scattered radiation.
Reflection
Reflection is a mechanism where radiation bounces off the surface of a material rather than being absorbed or transmitted. This characteristic of reflection is especially important in highly energetic radiation contexts, such as gamma rays, where reflective materials can prevent radiation from penetrating deeper into protective barriers.
What sets reflection apart is its ability to preserve the integrity of the shielding material over time. Since reflected radiation does not convert energy into heat, the material experiences less wear and tear, potentially extending its service life. However, the efficiency of reflection is greatly influenced by the surface properties of the material. Smooth, shiny surfaces tend to reflect radiation more effectively than rough or darker surfaces. Hence, while reflection can be an asset, careful attention to surface treatment and finish is critical for optimal performance.
Key Parameters Influencing Blocking Efficiency
Several factors shape the effectiveness of radiation blocking, and understanding these key parameters can lead to better choices in shielding material selection.
Material Density
Material density plays a pivotal role in blocking efficiency. Higher density materials can provide a greater likelihood of interaction with radiation, thereby enhancing attenuation. For instance, lead is favored in radiation shielding due to its substantial density and atomic number, making it ideal for environments such as X-ray rooms or nuclear facilities.
However, employing heavier materials can introduce complications, such as increased structural costs and challenges during installation. While high density is generally beneficial, considering the balance between efficacy and practical application is paramount in the design process.
Thickness of Shielding
The thickness of shielding material directly correlates to its ability to block radiation. Thicker barriers typically result in improved attenuation, but this comes at a cost—both financially and spatially. For example, a thicker lead wall will substantially improve shielding capabilities but might not be feasible in specific architectural contexts or where space is limited.
Ultimately, determining the optimal thickness requires a careful analysis of radiation type, exposure levels, and practical constraints to ensure an appropriate balance between safety and feasibility.
Energy Level of Radiation
The energy level of radiation is a critical element influencing blocking strategies. Different materials excel at attenuating distinct radiation energy levels. For example, low-energy photons can be effectively shielded using lighter materials, while higher-energy particles may necessitate denser and thicker materials.
This characteristic demands a nuanced approach when selecting shielding strategies, as one type of barrier might work well for certain wavelengths but fail for others. Hence, a thoughtful assessment of the energy profile of potential radiation sources is needed to make informed shielding choices, enhancing safety while optimizing resources.
Common Materials for Radiation Blocking
In the ever-evolving landscape of radiation shielding, utilizing the appropriate materials is crucial. Common materials for radiation blocking present distinct benefits and challenges, forming the foundation of effective radiation protection. By ensuring that these materials are well-understood, professionals can make informed decisions that promote safety in health, industrial, and research settings.
Lead
Properties and Applications
Lead stands out prominently when it comes to radiation shielding. One of its key characteristics is density. This density allows lead to absorb and attenuate various types of radiation efficiently. It has been the go-to material, especially in medical settings, where radiation exposure is a constant concern during procedures like X-rays and radiotherapy. Offering excellent protection, lead's flexibility in application makes it particularly desirable, whether in the form of sheets, bricks, or specialized garments.
Another unique feature of lead is its historical use. For decades, it's been the material of choice, instilling confidence in its protective qualities. However, its heaviness can be a drawback, making it cumbersome to handle in large quantities.
Limitations and Concerns
Despite its benefits, there are significant limitations and concerns surrounding lead use. One primary aspect is the health implications of lead exposure. As a toxic substance, lead can have detrimental effects on health if not handled properly. This toxicity has led to increasing scrutiny and calls for alternative materials or methods.
Furthermore, lead’s rigidity can be a disadvantage. The need for flexible, adaptive solutions in environments that are rapidly changing means that lead might not always be the best fit. Sort of an iron fist in a velvet glove, its overpowering presence may limit innovative applications in modern contexts.
Concrete
Use in Construction
Concrete, a staple in construction, offers notable advantages as a radiation shielding material. Its mass and density provide an effective barrier against radiation, making it a preferred choice in various facilities, such as nuclear power plants and hospitals. The use of concrete in constructing walls or protective bunkers significantly enhances safety while serving its primary structural purpose.
Adding to its appeal is concrete's cost-effectiveness; it is relatively inexpensive compared to specialized materials. Not only does it provide shielding, but it also contributes to the building's longevity and durability.
Comparative Effectiveness
When comparing concrete's effectiveness to other materials, it's important to consider the type of radiation. Concrete excels at blocking gamma rays but may fall short against neutron radiation unless specifically treated. Each factor plays a role in its utility.
In exploring its comparative effectiveness, one unique feature emerges: the ability to incorporate additives that improve its shielding properties. By mixing materials like barite or iron, concrete can be fine-tuned for specific shielding needs, enhancing its functionality.
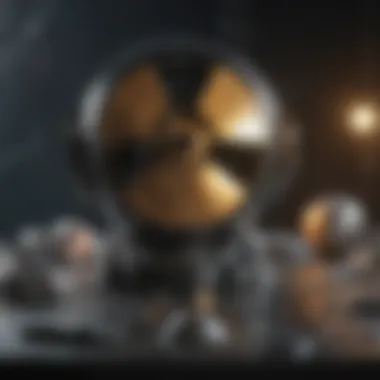
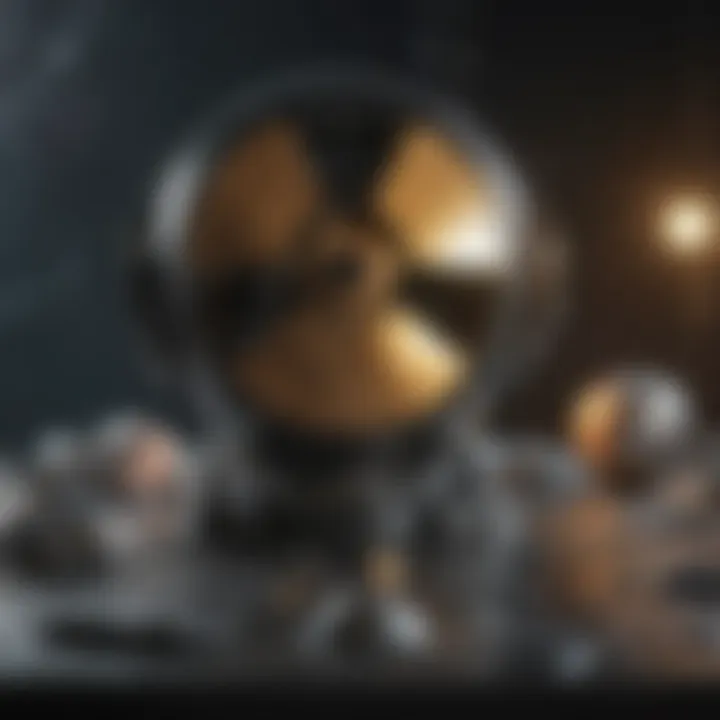
Polymer-Based Solutions
Flexible Options for Shielding
Polymers represent a fascinating shift in the landscape of radiation protection. Their flexibility is perhaps their most compelling attribute, enabling a range of applications from personal protective equipment to large-scale shielding solutions. These materials can be formulated into sheets that are lightweight and easy to manipulate, which is essential for industries that require adaptability, such as aerospace and healthcare.
A standout benefit of polymer-based solutions is their potential for customizing formulations. This tailoring allows for fine-tuning their shielding effectiveness based on specific radiation types and energy levels, making them versatile choices for various applications. Additionally, polymers are less toxic than lead, potentially minimizing health risks.
Performance Analysis
In terms of performance analysis, polymer-based solutions are becoming increasingly significant. Research is ongoing to determine how well these materials can absorb and scatter different radiation types, offering insights that could reshape how we approach shielding. Experimental findings showcase that some advanced polymers perform surprisingly well, often rivaling traditional materials under specific conditions.
One drawback, though, lies in their longevity. Depending on external environmental factors, polymers might degrade faster than metals or concrete, necessitating more frequent replacements and careful handling to maintain efficacy.
Through these materials, the article illustrates the complexities in selecting appropriate radiation shielding, focusing on innovations that hold promise for the future. Each material, whether it's the heavy-duty lead, trustworthy concrete, or the agile polymers, brings its own strengths and weaknesses, necessitating a tailored approach to shield against the unseen dangers of radiation.
Emerging Technologies in Radiation Blocking
In recent years, advancements in technology have fostered a new wave of radiation blocking solutions. These emerging technologies are critical not only for their potential applications in various fields but also for their impact on public health. The importance of these innovations lies in their ability to provide safer environments in workplaces, medical facilities, and even in our everyday lives. As we continue to unearth more about radiation exposure and its consequences, the need for better shielding becomes increasingly essential.
Nanotechnology Innovations
Nanoscale Materials and Shielding
Nanoscale materials hold significant promise in enhancing the efficacy of radiation shielding. The fundamental aspect of these materials is their incredibly small scale, often measured in nanometers. This minuscule size allows for unique interactions with radiation, resulting in improved absorption and scattering abilities.
The key characteristic of nanoscale materials is their tunability; scientists can manipulate their properties to suit specific shielding needs. This flexibility has made them a popular choice in the ongoing quest for effective radiation barriers.
One unique feature of nanoscale materials is their high surface area to volume ratio, which amplifies their effectiveness as a shielding agent. This characteristic can lead to lighter and thinner materials that maintain strong protective qualities. However, it's crucial to address some disadvantages, such as the potential environmental impacts of manufacturing and disposing of nanomaterials, as well as the challenges in testing their long-term stability in various conditions.
Research Developments
The development of research in the field of radiation blocking technologies is equally important. As scholars delve deeper into the characteristics and applications of these innovative materials, significant strides continue to be made. A primary focus of recent research has been the synthesis and application of novel composite materials that integrate nanotechnology and traditional shielding materials.
The key characteristic here is the combination of different properties into one composite, which could offer extensive protection against various types of radiation. The advancements make research developments a compelling area for exploration.
One unique aspect of this ongoing research is its interdisciplinary nature; it encompasses physics, materials science, and even environmental studies. However, an important disadvantage lies in the sometimes slow pace of regulatory approval for new materials, which can delay their introduction into the market and limit potential benefits.
Smart Materials
Adaptive Shields
Adaptive shields are at the forefront of innovation in radiation blocking technologies. This concept involves materials that can alter their properties in response to specific environmental cues, such as radiation intensity. The core feature of adaptive shields is their ability to provide on-demand protection, which dramatically enhances user safety in varying radiation environments.
One distinct advantage of adaptive shields is the potential for real-time monitoring and response. This capability not only increases safety but also reduces the need for bulky, static shielding structures. However, a notable disadvantage could be the complexity involved in creating reliable adaptive systems, which may raise costs and complicate implementation.
Response Mechanisms
The mechanisms by which adaptive shields respond to radiation can range from physical alterations to chemical changes. These response mechanisms are key to the operational efficiency of smart materials. A unique feature is their capacity to signal when the shield has been compromised, providing an added layer of safety for users.
Finally, while response mechanisms promise to revolutionize radiation blocking, they also introduce potential downsides. For instance, complications may arise during the integration of these systems into existing structures, necessitating careful planning and design.
"The future of radiation blocking technologies lies in marrying traditional materials with modern innovations—adaptability and effectiveness must go hand in hand."
Applications of Radiation Blocking
The applications of radiation blocking technologies are pivotal in safeguarding both public health and the environment. As radiation presents various risks across multiple fields, effective shielding solutions become a necessity rather than a luxury. These technologies are particularly crucial in domains like healthcare and industry, where exposure to radiation can have profound implications. When crafted thoughtfully, radiation blocking materials prove not only to protect but also to enhance operational efficiency in various settings.
Medical Field
Radiation Therapy Protections
Radiation therapy is a cornerstone of cancer treatment, effectively targeting malignant cells while attempting to preserve surrounding healthy tissues. The key characteristic of radiation therapy protections lies in their precision. One prominent aspect of these protections is their ability to limit unnecessary exposure to radiotherapy, thus minimizing acute side effects for patients.
In this regard, materials used in radiation therapy have unique features, such as lead aprons or specialized film screens, which absorb or deflect radiation effectively. One significant advantage of using these protective measures is the enhanced comfort and safety they offer patients and medical professionals alike, allowing for targeted treatment while mitigating risks. However, the disadvantage is the regular maintenance and potential costs associated with high-quality protective gear.
Diagnostic Imaging Shields
As diagnostic imaging techniques like X-rays and CT scans have become staples in medical diagnostics, the use of diagnostic imaging shields has surged. These shields are designed to reduce the risk of radiation exposure not only to patients but also to healthcare workers. The prominent feature of these shields is their mobility and adaptability, often comprising flexible materials for easy application during imaging procedures.
Such shields serve a significant purpose in ensuring that while patients receive necessary exams, health workers are also safeguarded against cumulative radiation exposure. An advantage of incorporating these shields is the automation in shielding, as many modern systems allow for real-time adjustments. However, their disadvantage lies in the potential for operator oversight, where shields may not be positioned effectively every time, leading to exposure risks.
Industrial Use
Nuclear Facilities
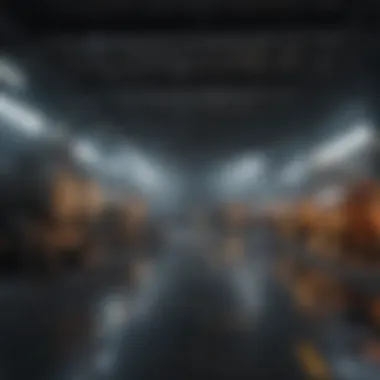
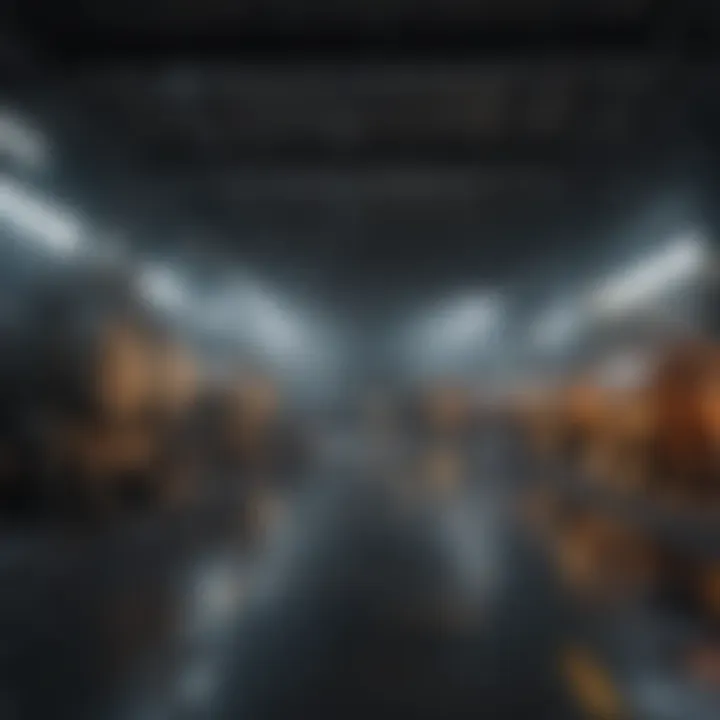
The construction and operation of nuclear facilities wield a significant need for robust radiation blocking technologies. These settings often require environmental safety protocols to ensure that radiation emissions do not adversely affect workers or surrounding communities. The notable characteristic of nuclear facilities is their use of extensive shielding systems, often employing thick concrete walls and specialized barriers made from lead and polyethylene.
These robust systems present a strong advantage in significantly curtailing potential radiation leakage, promoting a safe working environment. However, an inherent challenge lies in the upkeep and compliance with rigorous safety standards, ensuring that the shielding remains effective over time and complies with evolving regulations.
Radiation Monitoring Technologies
In industries where radiation exposure is a persistent concern, radiation monitoring technologies play a critical role in protecting workers. These systems include devices that measure radiation levels in real-time, providing immediate feedback about exposure levels. The key feature of these monitoring systems is their ability to trigger alerts when radiation levels exceed safe thresholds.
This real-time monitoring contributes greatly to workplace safety and enables employers to respond promptly to any radiation incident, ensuring that exposure remains within regulatory limits. An advantage of utilizing these technologies includes enhanced transparency in safety practices, although a disadvantage is the reliance on continuous calibration and monitoring to ensure accuracy and functionality.
In sum, the applications of radiation blocking encompass vital sectors such as healthcare and industry, where robust protective measures not only shield individuals from harmful radiation but also underpin operational integrity.
The advancements in these technologies reflect a growing recognition of radiation safety's significance and the need for continued research and development in the field.
Regulations and Safety Standards
Regulations and safety standards play a crucial role in the field of radiation blocking technologies. They ensure that the materials and approaches employed for radiation shielding are both effective and safe for human use. In this domain, adherence to guidelines is necessary not merely for compliance but also for fostering trust and integrity within the industries that utilize these technologies. Establishing a solid framework of regulations can help avert mishaps and promote a culture of safety that ultimately benefits society as a whole.
International Guidelines
WHO Recommendations
One significant aspect of the World Health Organization (WHO) recommendations is their holistic approach to radiation protection. The WHO emphasizes a comprehensive methodology that involves assessing exposure risks and implementing protective measures based on those assessments. This guideline is a beneficial choice for this article as it brings to light the importance of context and informed decision-making in radiation safety. A unique feature of the WHO recommendations lies in their adaptability; they are tailored for diverse settings, from healthcare facilities to industrial applications. This flexibility allows various stakeholders to apply these guidelines effectively. However, while the recommendations are widely accepted, their success hinges on the commitment to rigorous training and continuous education on radiation safety for all personnel involved—a challenge that cannot be overlooked.
IAEA Protocols
The International Atomic Energy Agency (IAEA) protocols offer another pivotal layer of regulatory framework in radiation protection. The core aspect of IAEA protocols focuses on enhancing global safety standards for radiation use in medical, industrial, and research contexts. One of the key characteristics of these protocols is their emphasis on standardized methodologies for risk assessment and mitigation—ensuring consistency across different national contexts. This makes IAEA protocols a popular choice in discussions surrounding radiation shielding efficacy. Their structured approach allows countries to develop and implement solutions compliant with international norms. However, a unique feature of the IAEA protocols is their emphasis on cross-border collaboration, which sometimes clashes with national regulations and creates tension as governments struggle to balance local needs with global standards.
National Regulations
EPA Standards
The Environmental Protection Agency (EPA) standards form a key segment of national regulations addressing radiation safety. In particular, these standards highlight environmental impacts and the management of radioactive materials. The key characteristic of EPA standards is their thoroughness—they apply to numerous aspects of radiation monitoring and control. This makes them a vital component of our discussion on radiation blocking technologies. What is unique about EPA standards is their focus not only on human health but also on ecological consequences, urging industries to factor in environmental protection alongside worker safety. While beneficial, one disadvantage is that the complexity of these standards can sometimes lead to confusion among smaller organizations lacking the requisite resources to navigate such regulations.
OSHA Regulations
Occupational Safety and Health Administration (OSHA) regulations specifically address the protection of workers in environments where exposure to radiation is a risk. An important aspect of OSHA regulations is the emphasis on establishing safe workplace practices and ensuring proper training for employees. This focus on worker safety makes OSHA regulations a critical part of this article, as it underscores the need for education and safety protocols in everyday operations involving radiation. A unique feature of OSHA regulations is their proactive approach—requiring companies to implement effective monitoring and reporting mechanisms. However, while these regulations are imperative for workplace safety, they may also pose challenges for compliance, especially for smaller companies that might struggle with the associated costs and administrative burdens.
In summary, regulations and safety standards form the bedrock of effective radiation blocking technologies. They not only protect human health but also foster responsible practices across industries. Exploring these guidelines illuminates the complexities and responsibilities that come with managing radiation exposure, highlighting the intersection of public policy, technological advancement, and community well-being.
Considerations for Future Research
Future research into radiation blocking technologies is crucial due to the evolving landscape of radiation exposure and shielding materials. As our daily lives increasingly integrate more technologies, the risks associated with radiation also transform. Understanding how to effectively block various types of radiation remains paramount for safeguarding human health and the environment.
Researchers need to bridge knowledge gaps that exist in the understanding of vulnerable populations and the performance of current materials under different conditions.
Addressing Gaps in Knowledge
Identifying Vulnerabilities
Identifying vulnerabilities in existing radiation blocking strategies is a key component in developing better protective measures. Vulnerabilities, in this context, refer to specific weaknesses in materials or situations where current shielding technologies may not perform effectively.
For instance, take healthcare environments where new medical imaging technologies are being employed. These technologies may expose staff and patients to new types of radiation, the characteristics of which might not be well-understood yet. By pinpointing these vulnerabilities, researchers can address underlying issues that could lead to health risks.
A significant characteristic of identifying these vulnerabilities is proactive safety enhancement. It creates a beneficial feedback loop where initial findings prompt further research and ultimately improved shielding solutions. However, there's a challenge – constantly evolving technologies require ongoing assessments, which could strain research resources and require timely updates to standards.
Enhancing Material Efficiency
Enhancing material efficiency is vital in developing effective radiation shielding solutions. As highlighted earlier, not all materials provide the same level of protection; therefore, optimizing these materials can result in significant advancements. For example, researchers are exploring composite materials that combine the strengths of lead and polymers.
The beauty of enhancing efficiency lies in sustainable solutions: using less material while maintaining high protection levels can reduce costs and decrease environmental impact. Yet, optimizing traditional materials might also lead to increased complexity in production processes, which could limit widespread adoption.
The Intersection of Policy and Technology
Research efforts need to navigate the gap between policy and technological innovation, which emphasizes the importance of creating an atmosphere where safety measures evolve alongside technological advancements.
Balancing Safety and Innovation
A balanced approach between safety and innovation is paramount in the domain of radiation blocking technologies. Striking this balance signifies that while new technologies emerge, they do not compromise safety measures already in place.
This intersection is crucial; if regulations become too prohibitive, the technological advancements could slow down. On the other hand, if innovation is prioritized without sound regulations, we could face dire consequences. The challenge lies in crafting flexible yet effective regulatory frameworks that adapt to new findings while ensuring a high standard of safety.
Funding for Research Initiatives
Funding for research initiatives in the radiation blocking field cannot be understated. Financial resources drive innovation, allowing researchers to explore new materials, conduct significant experiments, and ultimately advance the field.
However, securing funding can be a double-edged sword. While public funding may drive significant projects, it might necessitate navigating bureaucracy that could slow research progress. Furthermore, private investment might lead researchers to focus on commercially viable products rather than the most critical needs of the community.
Future research can determine protective measures essential for health and environmental safety.