Advancements in MS Proteomics and Its Applications
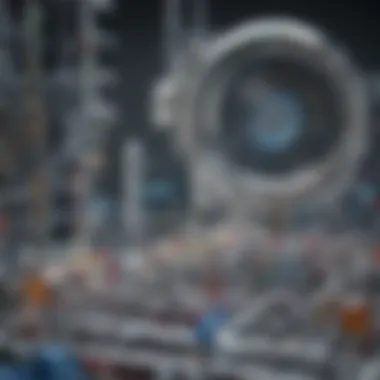
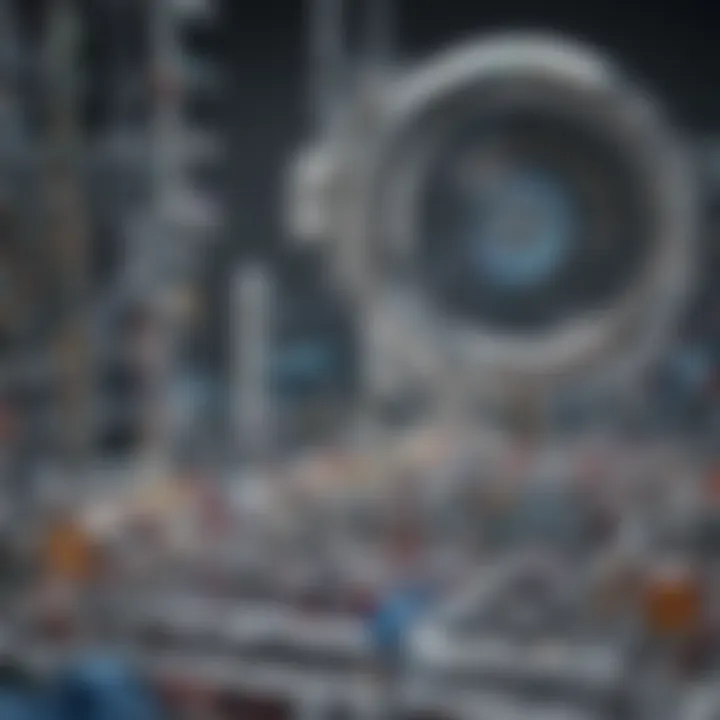
Intro
Mass spectrometry (MS) proteomics is a dynamic area of study that offers profound insights into biological systems. Over the years, this field has matured, showcasing its capabilities in protein identification, quantification, and characterization. The advancements in MS technologies have positioned the field at the intersection of fundamental research and clinical applications.
Understanding proteomics is essential for researchers and professionals alike. It encompasses the comprehensive analysis of proteins in various contexts, including their structure, function, and interactions. The accuracy and speed of mass spectrometry techniques have added a new dimension to how we approach biological questions.
In this article, we take a closer look at the global landscape of MS proteomics. Our goal is to dissect the methodologies that underpin this field and to explore its implications for medical and scientific advancements. By bringing together these elements, we aim to provide a thorough understanding of MS proteomics and its substantial role in enhancing knowledge in biological sciences.
Research Overview
Summary of Key Findings
Recent studies have demonstrated the ability of mass spectrometry to analyze complex protein mixtures with significant sensitivity and specificity. For instance, new tools and methods have emerged that allow for:
- Accurate identification of thousands of proteins from minute samples.
- Detailed quantification of protein expression levels under varying conditions.
- Insights into post-translational modifications that alter protein function.
These advancements are not only pushing the boundaries of what is technically possible, but they are also enhancing our fundamental understanding of biochemical processes.
Relevance to Current Scientific Discussions
The relevance of MS proteomics in contemporary discussions cannot be overstated. As researchers strive for precision in protein analysis, the demand for improved methodologies continues to grow. The conversation around proteomics also intersects with other cutting-edge domains such as:
- Genomics
- Metabolomics
- Systems biology
By integrating findings from these fields, scientists can develop holistic views of biological functions and disease mechanisms.
"The future of medicine lies in our ability to harness the power of proteomics for targeted therapies and personalized healthcare."
Methodology
Research Design and Approach
The study of MS proteomics often employs a multifaceted approach, combining quantitative and qualitative analyses. Different designs might focus on comparative studies, where protein expressions are analyzed under distinct experimental conditions, such as disease vs. healthy states.
Data Collection and Analysis Techniques
Data collection in MS proteomics usually involves several steps, including:
- Sample preparation, which is critical for successful outcomes. This can include techniques like ultrafiltration, precipitation, or solid-phase extraction.
- MS data acquisition, which typically involves tandem mass spectrometry (MS/MS) for deeper insight.
- Bioinformatics tools for data processing and interpretation to manage the vast amounts of data generated. These tools aid in identifying proteins and understanding their roles in biological pathways.
In summary, mass spectrometry proteomics continues to advance rapidly, opening doors for new applications in both research and clinical settings. By embracing these developments, we contribute to a deeper understanding of life at the molecular level.
Foreword to MS Proteomics
Mass spectrometry (MS) proteomics represents a frontier in the analysis of proteins, offering insights that are pivotal in both basic and applied sciences. The integration of mass spectrometry into proteomics has transformed how researchers study protein structures, functions, and interactions within complex biological systems. As this article unfolds, we will explore the fundamental principles, methodologies, and wide-ranging applications that define MS proteomics, emphasizing its critical role in advancing biological and medical research.
Defining Proteomics
Proteomics refers to the large-scale study of proteins, particularly their functions and structures. It involves analyzing the entire protein complement of a cell, tissue, or organism, enabling researchers to understand biological processes at a molecular level. This discipline encompasses various aspects, from protein expression to the identification of post-translational modifications. The analysis of proteins complements genomics, providing a comprehensive understanding of cellular functions, pathways, and mechanisms.
By examining proteins, researchers can obtain valuable data regarding cellular responses to different stimuli, understand disease mechanisms, and identify potential therapeutic targets. Given the vast number of proteins and the complexity of their interactions, proteomics provides a more nuanced view of biology compared to other "omics" approaches, enhancing our understanding across various fields, such as cancer research, immunology, and neuroscience.
Importance of Mass Spectrometry
Mass spectrometry is a cornerstone technique in proteomics, offering high sensitivity and precision in protein analysis. This analytical method separates ions based on their mass-to-charge ratios, allowing researchers to identify and quantify proteins in complex mixtures. The importance of mass spectrometry in proteomics can be summarized through several key advantages:
- High Sensitivity: MS can detect proteins at very low concentrations, which is particularly valuable in clinical diagnostics where biomarker identification is crucial.
- Detailed Characterization: Mass spectrometry provides information about the molecular weight of proteins, their structure, and any modifications they may undergo.
- Speed and Efficiency: Modern mass spectrometers can process hundreds of samples quickly, making them suitable for high-throughput proteomics.
Moreover, the ability of mass spectrometry to analyze complex samples directly, without extensive sample preparation, is a significant benefit. This capability allows for the exploration of proteomic landscapes in their native states, offering more accurate representations than traditional methods. As research continues in this area, the evolution of mass spectrometry technologies hopes to further enhance sensitivity and resolution while also decreasing analysis time.
The Basics of Mass Spectrometry
Mass spectrometry (MS) forms the backbone of proteomics research. Understanding its fundamentals is crucial for appreciating its role in analyzing proteins. MS allows scientists to determine the mass-to-charge ratio of ions, aiding in the identification and quantification of biomolecules. In proteomics, this capability translates to the study of protein structure, function, and interactions. Knowledge of basic MS principles enhances the interpretation of complex biological systems, making it an essential area of focus.
Principles of Mass Spectrometry
Mass spectrometry operates on fundamental principles. First, it ionizes chemical species to generate charged molecules. The generated ions are then sorted based on their mass-to-charge ratios in a vacuum. Each stage of this process is critical. There are typically four main stages: ionization, acceleration, deflection, and detection. By measuring the time it takes for ions to travel through an electric or magnetic field, we can identify the corresponding mass of the molecules. This method provides a powerful means of analyzing varied samples.
Key Components of a Mass Spectrometer
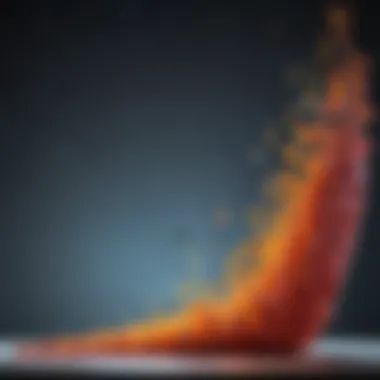
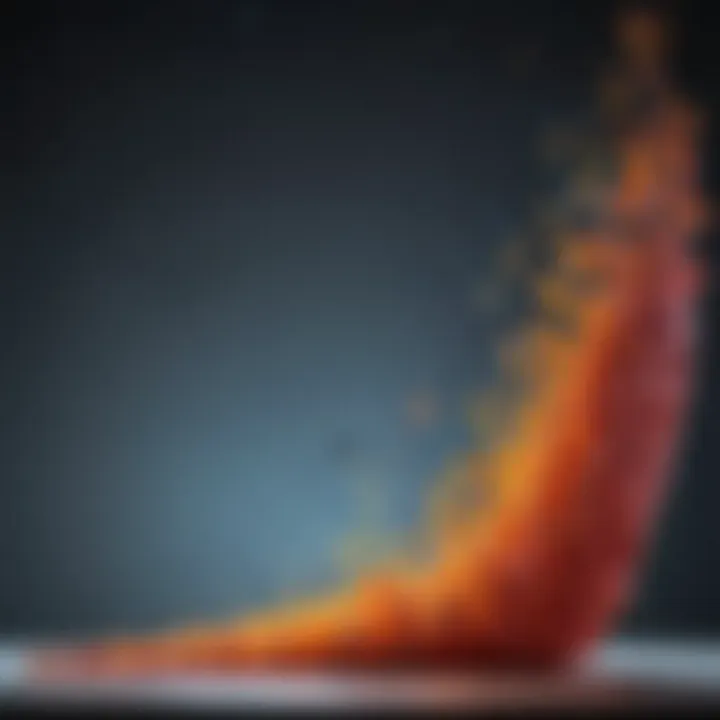
Mass spectrometers consist of several key components that work in tandem to analyze samples effectively. The three main parts are the ion source, mass analyzer, and detector.
Ion Source
The ion source is vital in generating ions from the sample. Various techniques exist, yet electrospray ionization is widely utilized due to its ability to produce ions from large biomolecules effectively. The importance of this aspect lies in how well it can ionize complex protein structures. This method allows for high sensitivity and is adaptable for diverse samples, a characteristic that distinguishes it in proteomics applications. However, challenges can arise, like the potential for ion suppression in complex mixtures.
Mass Analyzer
The mass analyzer is another crucial component. It sorts ions according to their mass-to-charge ratio. One widely used type is the time-of-flight (TOF) analyzer, known for its speed and efficiency. A unique feature of TOF analyzers is their ability to analyze a broad mass range with high resolution. Its increased throughput is a significant advantage, particularly beneficial in high-throughput proteomics studies. However, it may present challenges when working with very large or complex proteins.
Detector
Finally, the detector captures the sorted ions and generates a readable signal. One common detector is the electron multiplier, appreciated for its sensitivity. It allows scientists to detect even low-abundance proteins, making it suitable for various proteomics applications. The unique aspect lies in its rapid response time, enabling the analysis of dynamic processes. However, factors like dead time can impact overall performance in rapid succession experiments.
"Understanding the key components of mass spectrometry is essential for effective application in proteomics research."
To sum it up, the principles of mass spectrometry and its components define how effectively proteins can be analyzed in research. Mastery of these basics lays the groundwork for more advanced applications and methodologies in the realm of MS proteomics.
Methodologies in MS Proteomics
Methodologies in mass spectrometry (MS) proteomics are crucial for advancing our understanding of protein functions and their roles in complex biological systems. These methodologies are tailored to ensure accuracy in protein identification and quantification, which are essential for both basic and applied research. By focusing on well-defined methodologies, researchers can achieve reproducibility and reliability in their results, which is increasingly important in the field of proteomics. Furthermore, understanding these methodologies allows for better experimental design and application in clinical settings.
Sample Preparation Techniques
Protein Extraction
Protein extraction is a fundamental step in preparing samples for mass spectrometry analysis. This technique involves isolating proteins from biological matrices such as tissues or cells. The quality and type of extraction method significantly impacts downstream applications. Typical methods include sonication, ultrafiltration, and solvent precipitation. Each method has its characteristics.
One critical aspect of protein extraction is its efficiency in retaining the native state of the proteins, which is vital for accurate analysis. For example, using lysis buffers that maintain pH and ionic strength helps preserve protein function.
A beneficial characteristic of protein extraction methods is their adaptability to various sample types by adjusting lysis conditions. This versatility makes extraction techniques popular among researchers focused on different biological materials.
However, there are disadvantages as well; some extraction methods may lead to protein denaturation or loss of low-abundance proteins, which could skew results in proteomic studies. Thus, selecting an appropriate extraction technique is essential for achieving reliable results.
Digestion Methods
Digestion methods refer to the process of breaking down proteins into peptides, which are more suitable for analysis by mass spectrometry. This process is typically achieved using enzymes such as trypsin or chymotrypsin. Each enzyme has specific cleavage sites that determine the resulting peptide composition.
A key characteristic of digestion methods is their ability to generate peptides of optimal size for mass spectrometry, typically ranging from 5 to 30 residues. This size is crucial as it allows for effective ionization and detection.
Digestion methods are beneficial because they can be performed under controlled conditions, allowing for reproducible results. Furthermore, automated methods are now available, enabling high-throughput analysis.
On the downside, improper digestion can lead to incomplete cleavage, resulting in larger peptide fragments that are less identifiable. This variability can complicate data analysis and interpretation. Moreover, the choice of digestive enzyme affects the overall analysis, making method selection a significant consideration in proteomic studies.
Data Acquisition
Data acquisition encompasses the processes through which mass spectrometry captures, records, and analyzes the ions generated from digested peptides. It is a pivotal phase in proteomics as the quality of data acquired directly influences the outcome of the study.
Quantitative Analysis
Quantitative analysis in MS proteomics aims to determine the abundance of proteins within a sample. Utilizing techniques such as label-free quantification or isotopic labeling can facilitate this process. One distinguishing aspect of quantitative analysis is its ability to provide dynamic range across a wide spectrum of protein concentrations, which is highly valuable in comparative proteomics.
This aspect makes quantitative analysis a beneficial choice, allowing researchers to derive meaningful insights regarding protein expression changes in various conditions such as disease states or treatments.
However, one disadvantage is the potential for ion suppression effects, where the presence of highly abundant proteins can obscure the detection of less abundant ones. Therefore, careful experimental design is needed to improve the accuracy of quantitative analysis in MS.
Qualitative Analysis
Qualitative analysis focuses on identifying the proteins present in a sample rather than measuring their abundance. It often integrates database searching and spectrum matching to achieve this goal. Understanding the qualitative aspect is essential for protein characterization, particularly for discovering novel proteins and understanding post-translational modifications.
A significant characteristic of qualitative analysis is its capacity to uncover protein diversity, revealing modifications and interactions that suggest functional roles in biological processes.
Qualitative analysis is beneficial in exploratory studies because it provides a comprehensive overview of the protein landscape within a sample.
The downsides include the extensive computational efforts required and the need for robust databases, which can limit the interpretation of some experimental results. Furthermore, without quantitative measurements, it can be challenging to make strong biological conclusions based on qualitative data alone.
Data Analysis and Interpretation
Data analysis and interpretation are vital for translating raw mass spectrometry data into meaningful biological insights. This process involves the application of bioinformatics and statistical methods to tease apart complex datasets.
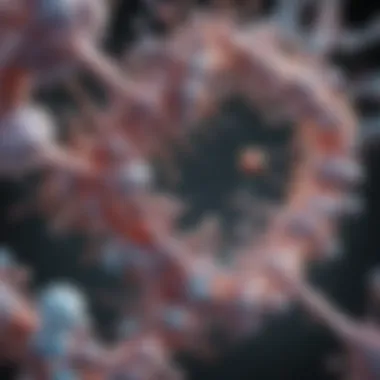
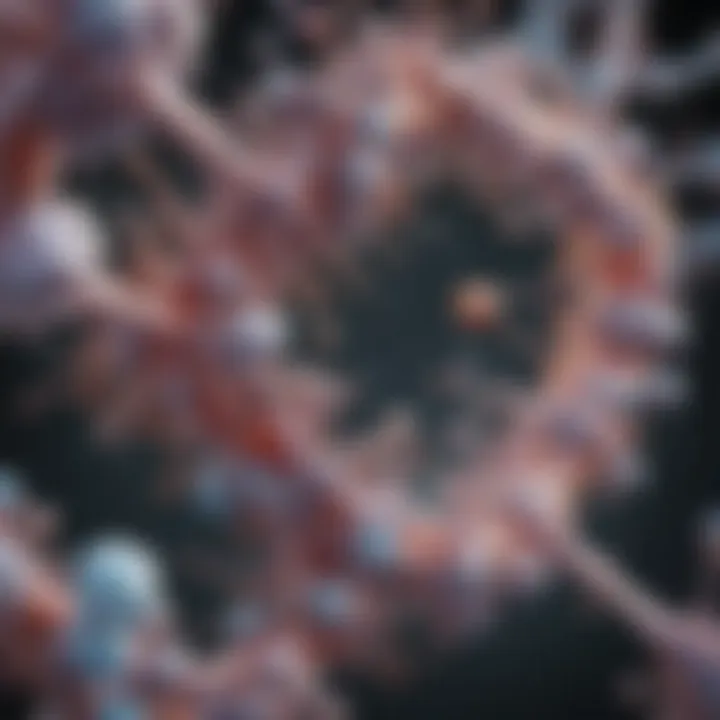
Bioinformatics Tools
Bioinformatics tools are software applications that aid in analyzing proteomic data. These tools help researchers search protein databases, assess post-translational modifications, and visualize data in an interpretable manner. A significant feature is their capacity to handle large datasets generated by modern mass spectrometry techniques.
Bioinformatics tools have become beneficial as they assist in reducing manual workload and minimizing human error in data interpretation. They also allow for integration with other omics data, providing comprehensive insights into biological functions.
However, one disadvantage is that the reliance on algorithms and databases can lead to discrepancies if the available databases are outdated or incomplete. This can affect data validity and researcher confidence in their findings.
Statistical Methods
Statistical methods in MS proteomics are employed to determine the significance of observed changes in protein levels or structures. These methods can include t-tests, ANOVA, and more complex multivariate analyses. A key characteristic is their ability to provide a framework for hypothesis testing, which is fundamental in scientific investigation.
Statistical analysis is considered beneficial because it allows researchers to draw conclusions that are not purely observational but rather supported by robust data evaluation. It helps in identifying proteins of interest or biomarkers with statistical confidence.
The challenge with statistical methods lies in their complexity; an incorrect application can lead to misleading results. Additionally, ensuring proper sample size and distribution is crucial in obtaining reliable statistical outputs.
Applications of MS Proteomics
Mass spectrometry proteomics plays a crucial role in a wide range of fields, shedding light on the complexities of biological systems and improving healthcare. The applications span from foundational research to clinical settings and environmental studies, emphasizing the multifaceted capabilities of this technology. Each area not only contributes to our understanding of proteins but also has practical implications that can influence scientific and medical advancements.
Basic Research
Protein Function Studies
Protein function studies are pivotal in uncovering the roles of proteins in biological systems. This area focuses on determining how proteins interact with each other and with other molecules. Understanding these interactions is vital in identifying pathways that drive biological processes. The key characteristic of protein function studies is their emphasis on functional assays and techniques, which make them a popular choice in basic research.
One unique feature of this approach is its ability to mapped interactive networks between proteins, which is essential for understanding cellular mechanisms. The advantages of protein function studies are significant; they provide insights into how proteins exert their effects, guiding potential therapeutics in drug design. However, challenges exist, including the complexity of cellular environments and variations in protein expression.
Pathway Analysis
Pathway analysis represents a systematic approach to understanding the flow of biological information through networks of interacting proteins. This methodology identifies critical pathways involved in various physiological processes and diseases. The key characteristic of pathway analysis is the ability to synthesize data from multiple sources to reveal overarching trends in protein interaction and cellular signaling. It is a beneficial choice for researchers looking to uncover the connections between proteins and biological functions.
What sets pathway analysis apart is its capacity to integrate high-throughput data, facilitating a comprehensive view of cellular activities. The advantages of this approach include the ability to pinpoint disruptions in pathways that may lead to disease states. Nonetheless, a disadvantage is that pathway analyses can be overly reliant on existing databases, which may not always capture novel findings.
Clinical Applications
Biomarker Discovery
Biomarker discovery is one of the most transformative applications of MS proteomics. This process involves identifying proteins that serve as indicators of diseases or conditions, providing valuable information for diagnosis or prognosis. The emphasis on specificity and sensitivity makes biomarker discovery a popular topic among clinical researchers.
A unique aspect of biomarker discovery is its potential to use proteomic signatures to differentiate between healthy and disease states. This capability leads to early detection of diseases, which significantly improves clinical outcomes. The advantages include the possibility of personalized medicine, targeting therapies more effectively. However, challenges such as validation and reproducibility of biomarkers remain critical roadblocks.
Drug Development
Drug development benefits from MS proteomics through insight into how proteins respond to pharmaceutical compounds. Understanding these interactions is central to enhancing drug efficacy. The key characteristic of drug development through MS proteomics is its detailed analysis of protein-drug interactions, which supports rational drug design. Such detailed profiling provides a clear advantage in identifying targets for new drugs and optimizing lead candidates.
The special feature of this application lies in its ability to profile changes in the proteome due to drug treatment, revealing a wealth of knowledge on drug mechanisms. The benefits are clear; faster drug development timelines and informed decision-making during the drug discovery process. On the other hand, the complexity of biological systems can hinder the predictability of drug effects, leading to gaps in understanding.
Environmental Proteomics
Toxicology Studies
Toxicology studies harness MS proteomics to evaluate the impact of toxins on living organisms. This aspect is particularly relevant for understanding how environmental contaminants affect protein functions, cellular pathways, and overall health. The focus on real-world applications makes toxicology studies valuable in environmental science.
One unique feature of this research area is that it can link exposure to substances with biological responses, revealing critical insights into health risks. The advantages lie in its contribution to risk assessments and providing evidence for regulatory decisions. Nonetheless, data interpretation can be complex due to the diversity of environmental factors influencing protein behavior.
Biodiversity Assessment
Biodiversity assessment utilizes MS proteomics to explore the variety of proteins present in different species or ecosystems. This approach contributes significantly to conservation biology and ecological studies. The key characteristic of biodiversity assessment is its capacity to catalog and characterize various organisms at the molecular level, making it an advantageous tool in environmental proteomics.
What sets this application apart is that it allows for the assessment of ecosystem health and the effects of environmental changes on biodiversity. The advantages include better management practices for conservation and insights into species interactions. However, challenges include the need for comprehensive databases and the skills required to analyze complex datasets effectively.
Overall, the applications of MS proteomics demonstrate its versatility and impact across various respectable domains. Each application area not only enhances basic scientific understanding but also offers practical benefits that resonate in clinical, environmental, and research contexts.
Recent Advances in MS Proteomics Technology
Recent developments in mass spectrometry proteomics technology are crucial for improving our understanding of biological systems. The ongoing refinement of techniques significantly enhances sensitivity and specificity. These innovations open new avenues not only for basic research but also for translational applications in medicine and environmental sciences. As proteomics generates large volumes of data, advancements allow for more efficient data handling and analysis. These improvements facilitate more nuanced insights into protein interactions, functions, and modifications, ultimately driving the field forward.
New Ionization Techniques
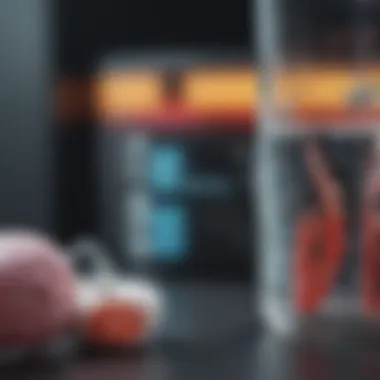
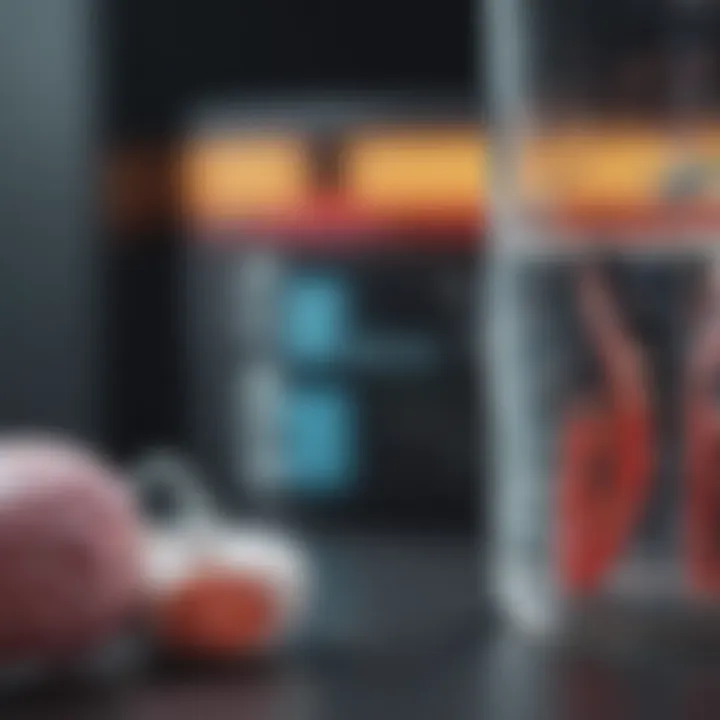
New ionization techniques have emerged, quickly becoming essential in mass spectrometry proteomics. Techniques like nano-electrospray and matrix-assisted laser desorption/ionization (MALDI) provide enhanced efficiency in ion generation from complex biological samples. These methods significantly increase the detectability of low-abundance proteins, enabling researchers to study a wider range of proteins in various samples. By optimizing ionization, scientists improve both the yield and quality of analytes, enabling more comprehensive analyses.
Advancements in Mass Analyzers
Advancements in mass analyzers, such as the development of high-resolution mass spectrometry, are revolutionizing how we approach mass spectrometry proteomics. Improved mass analyzers can distinguish proteins with slight differences in mass, facilitating the identification of post-translational modifications. This capability enables deeper investigations into protein function and interactions. The ability to achieve higher resolution also helps in complex mixtures, where distinguishing between closely related proteins is critical. These enhancements maximize the potential for discovery within biological research and diagnostics.
Integrative Approaches with Other Omics
Integrative approaches that combine proteomics with genomic and metabolomic data are gaining traction. This multidisciplinary strategy enhances the level of biological insight and understanding.
Genomics
Genomics plays a crucial role in identifying the genetic basis of protein expressions. Through gene sequencing, researchers uncover how genetic variations impact protein profiles, which can lead to understanding disease mechanisms. The key characteristic of genomics is its ability to link genetic information to protein expression, making it a powerful tool in MS proteomics. This synergy allows for a more holistic understanding of cellular functions. However, limitations exist, including the complexity of interpreting the functional implications of genomic data in a proteomic context.
Metabolomics
Metabolomics, the study of metabolic profiles, provides insight into cellular processes and metabolic pathways. This approach complements proteomics by revealing how proteins interact with metabolites. The key characteristic of metabolomics is its ability to detect small molecules, giving context to protein functions within metabolic pathways. This field is becoming increasingly important in deciphering the biochemical responses of organisms in various environments. While metabolomics offers vast amounts of information, challenges include data complexity and the need for sophisticated analytical tools to provide meaningful insights.
Challenges in MS Proteomics
Mass spectrometry (MS) proteomics faces multiple challenges that can hinder its effectiveness and applicability in addressing biomedical questions. Understanding these challenges is crucial for researchers in the field. They not only spotlight areas needing improvement but also explore the implications of the technology's constraints. Addressing these challenges is necessary for unlocking the full potential of MS proteomics.
Technical Limitations
The technical limitations of MS proteomics involve issues that arise from the equipment, sample processing, and the very nature of proteins. One key limitation is the sensitivity of mass spectrometers. For certain proteins present in small quantities, detection can become problematic. This limitation can alter research results, especially when studying rare proteins or those expressed in minute concentrations.
Another challenge is the resolution of mass analyzers. While modern instruments have made significant advancements, some low-abundance proteins or those that are structurally similar may remain undetectable or misinterpreted. The issues surrounding ionization efficiency can further compromise quantitative analysis. When proteins do not ionize well, their concentrations can appear inaccurately low.
Sample preparation also poses challenges. Protein extraction methods and digestion techniques may introduce variability. Differences in preparation can lead to inconsistent results, influencing data comparability across experiments. Thus, developing standardized protocols is vital for ensuring reproducibility in MS proteomics.
Data Complexity and Management
Data complexity in MS proteomics is a significant challenge due to the vast amount of information generated during experiments. A single run can yield thousands of data points. Managing this data requires sophisticated bioinformatics tools and statistical methods to ensure meaningful interpretation. With various experimentally derived datasets, integrating and analyzing such complex data can overwhelm even experienced researchers.
Additionally, data management processes can introduce biases and errors. When handling large datasets, it is easy to miss patterns or make false correlations due to sheer volume. Proper training in bioinformatics is crucial for researchers to navigate this complexity effectively.
Moreover, the interpretation of MS proteomics data demands precise contextual knowledge. Proteins can undergo post-translational modifications, which complicates the identification and quantification processes significantly. This level of intricacy necessitates interdisciplinary collaboration, combining domain knowledge from biology, bioinformatics, and statistics.
"As researchers strive to interpret complex proteomics data, the risk of misanalysis increases, underscoring the need for robust data management strategies."
Future Perspectives in MS Proteomics
The future of mass spectrometry (MS) proteomics holds remarkable promise, marked by important advancements and myriad applications. With rapid technological evolution, MS proteomics is positioned to revolutionize biological research and clinical settings. The growing need for precise protein analysis in various fields, from academia to industry, underscores the relevance of this topic. As we explore future perspectives, it is essential to highlight emerging trends, potential applications in personalized medicine, and the implications this field may have in shaping future healthcare paradigms.
Emerging Trends
Several emerging trends are influencing the field of MS proteomics. Notably:
- Integration of Artificial Intelligence: The incorporation of machine learning and artificial intelligence is making data analysis more efficient. These technologies can help in identifying patterns in large datasets, improving predictive capabilities.
- Advancement in High-Resolution Mass Spectrometry: Improved resolution enables better identification of complex peptide mixtures. This leads to more accurate proteomic profiles and is crucial for understanding biological processes.
- Miniaturization and Automation: Smaller, automated systems allow for high-throughput analysis. This increases the accessibility of proteomics in routine diagnostics and research.
- Cross-Omics Approaches: The integration of proteomics with genomics and metabolomics offers comprehensive insights into biological systems, further enriching our understanding of health and disease.
These trends showcase a shift towards more sophisticated methodologies that enhance the robustness and applicability of MS proteomics in various domains.
Potential for Personalized Medicine
Personalized medicine represents a monumental shift in healthcare, emphasizing tailored treatment strategies based on individual patient profiles. MS proteomics plays a pivotal role in this transformation by:
- Identifying Biomarkers: Reliable biomarkers for diseases can be detected through proteomic analyses. These markers assist in early diagnosis and prognosis, leading to better treatment outcomes.
- Understanding Drug Response: MS proteomics can elucidate how different patients respond to specific drug treatments, providing insights that lead to more effective therapies tailored to an individual's unique proteomic profile.
- Monitoring Disease Progression: Continuous proteomic profiling allows for monitoring changes in protein expression over time. This helps in understanding disease dynamics and adjusting treatment plans accordingly.
- Enhancing Clinical Trials: By including proteomics in clinical trial design, researchers can better stratify patient populations based on proteomic signatures, optimizing the chances for successful outcomes.
"The application of mass spectrometry in personalized medicine could transform how diseases are diagnosed and treated, providing thorough insights based on individual protein profiles."
As we move forward, the continued collaboration between proteomics and other fields will likely drive more innovative applications, making it essential for the scientific community to remain engaged and informed.
Ending
The conclusion serves as a crucial summary of the insights and advancements in mass spectrometry (MS) proteomics discussed throughout this article. It encapsulates the essence of the findings and their implications for both current and future research. A robust understanding of MS proteomics not only highlights its methodologies but also showcases its applications across various fields, from basic research to clinical practices.
Summary of Key Findings
Mass spectrometry proteomics has revealed significant advancements that enhance protein analysis. Key findings include the improvement of sample preparation techniques, which streamline the extraction and digestion of proteins, ultimately leading to more accurate data acquisition. The integration of advanced data analysis tools has also been critical, allowing researchers to interpret complex proteomic data more effectively. Additionally, the article outlined the versatile applications of MS proteomics in biomarker discovery, drug development, and ecological assessment.
- Enhanced methodologies for protein extraction and digestion.
- Sophisticated bioinformatics tools aiding data interpretation.
- Diverse applications ranging from clinical to environmental studies.
Implications for Future Research
Looking ahead, the potential of MS proteomics in transforming biological research is noteworthy. The data generated from this technology can inform personalized medicine strategies and offer valuable insights into disease mechanisms. Future research could explore the method optimization in proteomic workflows to further increase sensitivity and specificity. Moreover, as technological advancements occur, the coupling of mass spectrometry with other omics techniques promises to provide a more holistic understanding of biological systems.
- Personalized medicine driven by insights from proteomic data.
- Optimization of methodologies for enhanced proteomic analysis.
- Integration with genomics and metabolomics for comprehensive insights.