Advancements in Fuel Cell Research and Their Implications
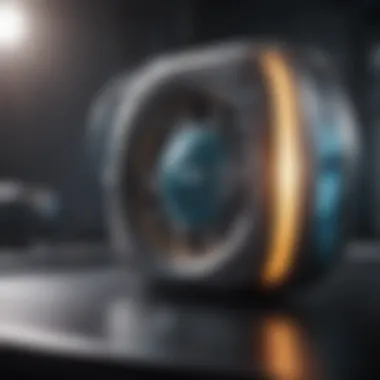
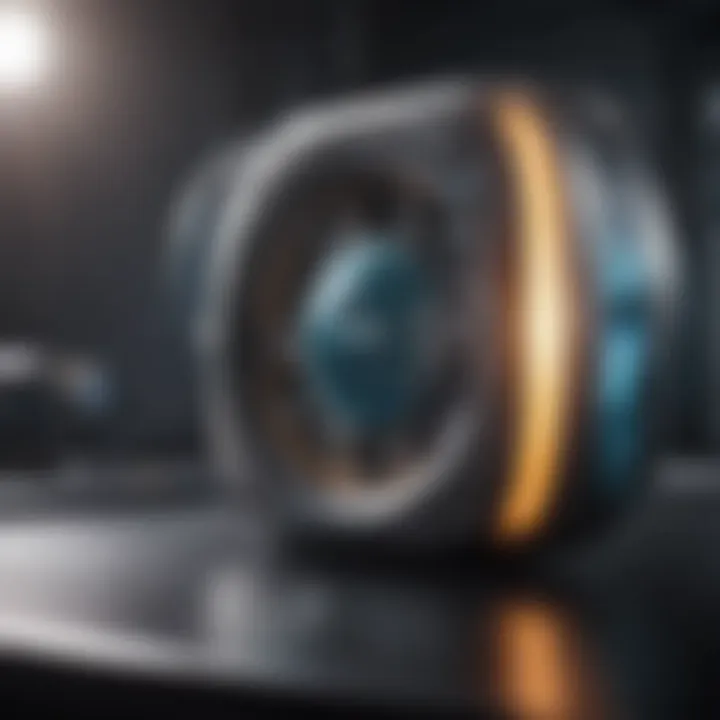
Intro
Fuel cell research is an essential discourse in the search for sustainable energy solutions. As the world grapples with climate change and energy security, it becomes critical to explore innovative technologies that can provide clean energy. Fuel cells represent a promising avenue, offering a means to convert chemical energy into electrical energy efficiently, with water as the main byproduct. This aspect of fuel cells is vital in addressing the increasing global energy demands, particularly as reliance on fossil fuels diminishes.
Research in this area encompasses various disciplines, including materials science, electrochemistry, and systems engineering. The effective application of fuel cell technology holds the potential to redefine our energy landscape. In the following sections, we will delve into the nuances of this research, highlighting various types of fuel cells, recent breakthroughs, methodologies, and future research directions.
Research Overview
Summary of Key Findings
Fuel cell technology has seen substantial advancements over recent years. Research has focused on improving efficiency, reducing costs, and enhancing the durability of fuel cells. Some notable findings include:
- Proton Exchange Membrane Fuel Cells (PEMFCs) have gained traction due to their high efficiency and versatility. New materials for membranes are being developed to extend their lifespan.
- Solid Oxide Fuel Cells (SOFCs) are being optimized for high-temperature operations, making them suitable for large-scale applications.
- Advances in electrocatalyst development have significantly reduced the amount of precious metals needed for reactions, helping to drive down costs.
These key advancements not only showcase the growing potential of fuel cells but also suggest avenues for future research.
Relevance to Current Scientific Discussions
As the world transitions toward greener energy practices, fuel cell technology plays a crucial role in scientific discussions. The integration of fuel cells with renewable energy sources, such as solar and wind energy, presents a compelling strategy for achieving energy sustainability. Moreover, as industries move toward decarbonization, fuel cells offer a pathway to reduce greenhouse gas emissions across various sectors, including transportation and power generation.
Methodology
Research Design and Approach
The methodology employed in fuel cell research varies substantially based on the focus area. Research often involves a mix of experimental and computational techniques. Some studies concentrate on material synthesis, where new catalysts and membrane materials are created and tested for their electrochemical properties. Other studies might focus on modeling fuel cell systems to predict performance under varying conditions.
Data Collection and Analysis Techniques
Data collection is pivotal in validating hypotheses and assessing progress. Common techniques include:
- Characterization Methods: Techniques such as scanning electron microscopy and X-ray diffraction help in examining materials at the microscopic level.
- Electrochemical Testing: Methods like electrochemical impedance spectroscopy provide insight into the efficiency and reaction kinetics of fuel cells.
Data analysis typically involves statistical approaches to interpret results, ensuring that findings contribute meaningfully to the broader understanding of fuel cell technology.
"The advancements in fuel cell research stand as evidence of our ability to innovate and adapt in the face of energy challenges."
In summary, the exploration of fuel cell technology reveals a field filled with potential and vitality. With ongoing research, the pathway toward sustainable energy systems becomes clearer, encouraging further study and application of fuel cells in various contexts.
Preamble to Fuel Cells
Fuel cells represent a significant leap in our quest for efficient and sustainable energy solutions. As societies globally seek alternatives to conventional energy sources, fuel cell technology positions itself as a crucial player in the transition towards renewable energy systems. This section aims to elucidate the essence of fuel cells, their operational principles, and their historical significance, setting the stage for a deeper exploration of subsequent topics.
Definition and Functionality
A fuel cell is an electrochemical device that converts chemical energy directly into electrical energy through an electrochemical reaction. Unlike conventional batteries, which store energy, fuel cells continuously produce energy as long as they are supplied with fuel and an oxidizer.
Most commonly, hydrogen serves as the fuel, while oxygen from the air acts as the oxidizer. The reaction within a fuel cell results in the production of electricity, water, and heat. Importantly, this process is efficient and generates minimal emissions, which is a key advantage in the pursuit of eco-friendly energy solutions.
Fuel cells can operate under various conditions and are classified by the type of electrolyte they employ. This classification allows for specific applications ranging from small portable devices to large power plants, showcasing the versatility and potential of fuel cell technology in meeting diverse energy needs.
Historical Background
The concept of fuel cells is not a recent innovation. The earliest iteration dates back to 1839 when Sir William Grove, a British scientist, developed the first fuel cell, known as the Grove cell. However, it was not until the mid-20th century that fuel cell technology began to gain traction, primarily influenced by space exploration activities.
NASA utilized hydrogen-oxygen fuel cells in its Apollo missions, showcasing their reliability and efficiency. This pivotal moment highlighted the potential of fuel cells beyond mere theoretical applications. In the following decades, numerous research initiatives and investment in fuel cell technology emerged, spurred by the quest for alternatives to fossil fuels amid increasing environmental concerns.
In this historical context, understanding the evolution and the milestones in fuel cell research emphasizes why this technology is vital today. It signifies years of innovation that led to the advanced systems we see now, prompting both interest and investment from various sectors.
Types of Fuel Cells
Understanding the types of fuel cells is crucial for comprehending their various applications and advantages. Each type operates on distinct principles, utilizing different materials and processes to convert chemical energy into electrical energy. The diversity in fuel cells allows for tailored solutions to meet specific energy needs, ranging from stationary power generation to portable applications.
Proton Exchange Membrane Fuel Cells
Proton Exchange Membrane Fuel Cells (PEMFCs) are known for their efficiency and low operating temperature, typically around 80 degrees Celsius. They function by passing hydrogen through a membrane that allows protons to travel while blocking electrons. The electrons are directed through an external circuit, generating electricity. The simplicity of this design leads to a quick startup time, making PEMFCs suitable for vehicles like cars and buses. Furthermore, their lightweight and compact nature enhances their usability in applications where space is a constraint.
Solid Oxide Fuel Cells
Solid Oxide Fuel Cells (SOFCs) operate at higher temperatures, generally between 600 and 1,000 degrees Celsius. They utilize a solid electrolyte, which can be ceramic, to permit oxygen ions to move from the cathode to the anode side. This technology is appealing for stationary power generation due to its high efficiency and ability to use various fuels, including natural gas and biogas. The primary drawback is the high operating temperature, leading to challenges in material selection and system design, which impacts longevity and cost.
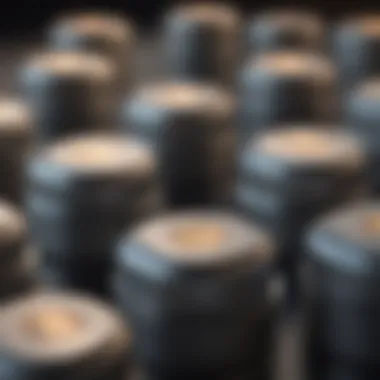
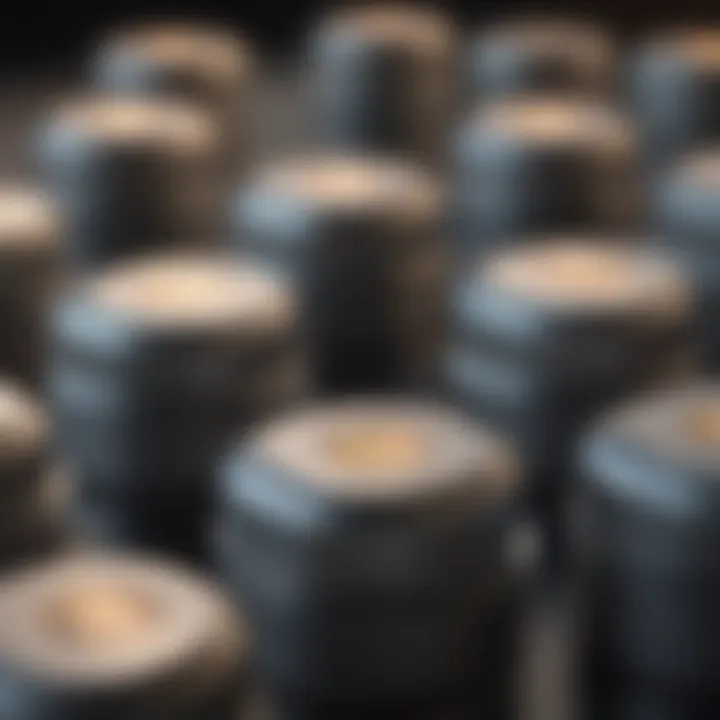
Molten Carbonate Fuel Cells
Molten Carbonate Fuel Cells (MCFCs) operate at even higher temperatures, around 600 degrees Celsius, and utilize a molten carbonate salt as the electrolyte. They can also utilize natural gas or biogas, transforming it into hydrogen and carbon dioxide. MCFCs are particularly suited for large-scale power plants because of their efficiency and versatility in fuel choices. However, they face challenges regarding corrosion and require significant investment for implementation in commercial settings.
Alkaline Fuel Cells
Alkaline Fuel Cells (AFCs) use an alkaline electrolyte, such as potassium hydroxide, and operate at lower temperatures (60-100 degrees Celsius). These cells are particularly sensitive to carbon dioxide, which can negatively affect their performance. First developed for the space program, AFCs are known for their simplicity and cost-effectiveness, making them attractive for specific uses. However, their reliance on pure hydrogen limits their practicality compared to other fuel cell types.
Mechanisms of Operation
Understanding the mechanisms of operation in fuel cell technology is vital for anyone interested in energy solutions. This section explores the core processes that fuel cells rely on, shedding light on their functionality and efficiency. Comprehending these principles helps to appreciate the technological advances and the challenges inherent in refining fuel cells for practical applications.
Electrochemical Reactions
At the heart of fuel cell functionality are the electrochemical reactions that convert chemical energy into electrical energy. These reactions occur between the fuel, typically hydrogen, and an oxidizer, usually oxygen from the air, facilitated by an electrolyte. During this process, electrons are released and can be harnessed to generate electricity.
The chief reaction in a hydrogen fuel cell can be summarized as follows:
- Hydrogen molecules split into protons and electrons at the anode.
- Protons traverse through the electrolyte to reach the cathode, while electrons flow through an external circuit, generating an electric current.
- At the cathode, the electrons reunite with the protons and combine with oxygen to create water and heat.
This reaction is essential not just for productivity, but also for the environmental benefits associated with fuel cell technology. By understanding these electrochemical interactions, researchers can explore ways to optimize them, leading to more effective energy conversion processes.
Thermodynamics and Efficiency
Thermodynamics plays a crucial role in evaluating the efficiency of fuel cells. It relates to how energy transfers and transformations occur within a fuel cell system. Efficiency may be compromised if energy losses arise during these transitions.
A key consideration in thermodynamics is the Gibbs free energy, which indicates the maximum usable energy available from the electrochemical reactions. The overall efficiency of a fuel cell can be affected by several factors:
- Operating Temperature: Different types of fuel cells operate at varying temperatures. For example, Solid Oxide Fuel Cells function at high temperatures, which can yield higher efficiencies but also pose challenges in material integrity.
- Fuel Purity: The presence of impurities in the fuel can result in catalysts becoming less effective, which ultimately reduces efficiency. It's crucial for researchers to investigate purification methods to enhance performance.
- Waste Heat Recovery: Utilizing waste heat is an effective strategy for increasing overall system efficiency, allowing fuel cells to contribute more effectively to combined heat and power applications.
In summary, the thermodynamic factors that influence performance must be meticulously analyzed and addressed to improve the operational efficiency of fuel cells.
Fuel cells represent a transformative technology in our pursuit of sustainable energy, but understanding their operational mechanisms is crucial for maximizing performance.
Delving into these mechanisms provides a clearer picture of potential improvements and innovations, shaping the future landscape of fuel cell technology.
Recent Advances in Fuel Cell Research
Recent advances in fuel cell research have garnered significant attention in the renewable energy domain. With rising energy demands and environmental concerns, the evolution of fuel cells plays a crucial role in achieving sustainable solutions. Researchers are exploring new materials, enhancing durability, and implementing cost reduction strategies that can potentially shift the landscape of energy production.
Innovation in Materials
The development of new materials is a vital area in fuel cell research. Innovative compounds can lead to enhanced electrochemical performance, which directly improves efficiency and functionality. For instance, membrane electrode assemblies are evolving thanks to new polymer technologies. These advancements aim to create membranes that are more conductive while being less susceptible to chemical degradation over time. Moreover, catalysts made with low Platinum content or entirely non-precious metals are gaining traction. The focus on these materials not only reduces dependency on expensive raw materials but also lowers production costs.
- Key benefits of innovative materials include:
- Improved energy conversion efficiency
- Increased operational temperature range
- Enhanced resistance to degradation
Incorporating materials like graphene and nanostructured catalysts can be a game changer in advancing fuel cell technology.
Improved Durability and Longevity
Durability is critical for fuel cells because their applications span diverse environments, from automotive to stationary power generation. Recent research emphasizes not only enhancing durability but also understanding degradation mechanisms. Tools such as in-situ techniques are being used to monitor performance in real-time and diagnose issues that lead to failure. Improved durability results in longer life spans for fuel cells, making them more attractive for implementation.
The advantages of enhanced durability are significant:
- Reduced maintenance costs
- Increased system reliability
- Longer intervals between replacements
Studies continue to explore coatings and advanced production methods that resist corrosion and thermal stress, which are crucial for enduring performance.
Cost Reduction Strategies
Cost remains a barrier for wide-scale adoption of fuel cell technologies. Therefore, innovative cost reduction strategies are pivotal to making fuel cells a competitive alternative. Methodologies range from lean manufacturing processes to engineering improvements that simplify assembly. Research is focused on optimizing the use of raw materials and finding abundant alternatives, especially in catalyst development.
Implementing economies of scale in production is another valuable strategy. As fuel cell technologies mature, production facilities are designed to handle larger outputs with lower costs. Additionally, collaborative efforts across academia, industry, and government are essential to pooling resources and knowledge to drive down prices.
Some cost reduction strategies include:
- Utilization of abundant materials like copper instead of scarce resources like Platinum.
- Mass production technologies that harness automation and advanced machining.
- Streamlined supply chains that reduce transport and handling costs.
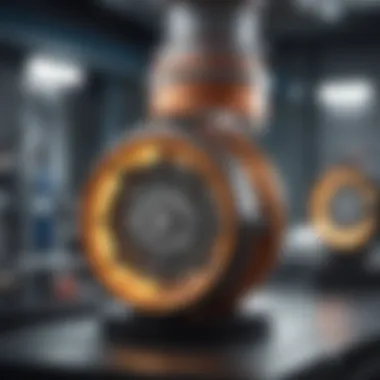
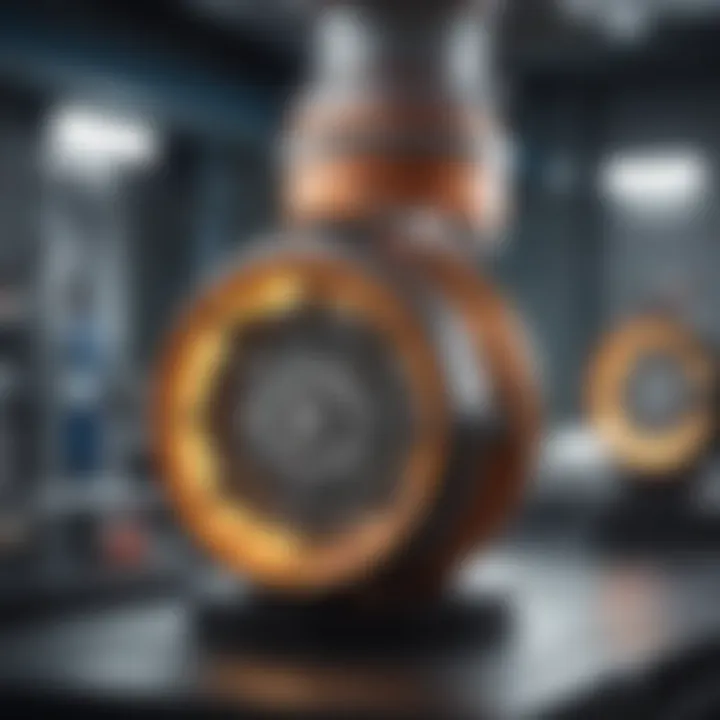
In summary, recent advances in fuel cell research are setting the stage for transformative impacts across multiple sectors. With focus on materials innovation, durability improvements, and cost efficiencies, the potential for fuel cells in energy systems becomes more pronounced, paving the way for wider acceptance and integration into our energy future.
Challenges in Fuel Cell Development
The development of fuel cells faces several challenges that hinder widespread implementation and acceptance. Understanding these challenges is crucial for stakeholders involved in research, industry, and policy making. The issues range from technical barriers to economic factors, and also include regulatory and safety concerns. Addressing these challenges could significantly enhance the viability of fuel cell technology in the energy market, making it a priority for researchers and companies alike.
Technical Barriers
Technical barriers represent one of the most significant challenges in fuel cell development. These barriers often relate to the technological limitations of current fuel cell designs. For instance, the efficiency and durability of fuel cells must meet specific thresholds to compete with traditional energy sources. Researchers face complex issues involving material innovation. Many existing membranes and catalysts used in fuel cells degrade over time, reducing efficiency and increasing maintenance needs.
Another aspect is the fuel cell operational requirements, including temperature and pressure conditions. Some fuel cells operate effectively only under specific conditions, limiting their applications. Lowering the operating temperature of Solid Oxide Fuel Cells, for instance, can improve accessibility but leads to efficiency loss. Such trade-offs complicate the design process and can deter potential investors.
Economic Factors
Economic factors are another layer of challenges affecting fuel cell development. The cost of production and installation remains high, which can deter potential users. While costs have decreased in recent years, they are still significant compared to conventional energy sources like fossil fuels. Making more affordable fuel cells requires an understanding of material costs, manufacturing processes, and supply chain management.
Moreover, there is a risk of market volatility. Fluctuations in the pricing of critical materials, such as platinum used in catalytic processes, impact the overall cost-effectiveness of fuel cells. To make them a viable alternative, strategies for cost reduction must continue to evolve. This includes seeking alternative materials that could provide similar efficiency at lower prices.
Regulatory and Safety Concerns
Regulatory and safety concerns contribute to the hesitance surrounding fuel cell technology. Existing regulations can be cumbersome and challenging, especially in comparison to more established energy technologies. New standards and guidelines need to be developed to ensure safe operation and installation of fuel cells. This adds complexity and time to the deployment process.
Furthermore, safety cases must be constructed, particularly around hydrogen fuel storage and handling. Hydrogen is highly flammable, which creates a need for stringent safety protocols. Developing these protocols presents its own set of challenges, often requiring extensive testing and validation before fuel cells gain acceptance in various sectors.
The development of solutions to these challenges can lead to more widespread adoption of fuel cell technology, helping transition to sustainable energy systems.
Applications of Fuel Cells
The applications of fuel cells are critical to harnessing their potential for a sustainable energy future. Fuel cells have gained attention due to their high efficiency and low emissions, making them an attractive option across various sectors. Their versatility allows them to serve many purposes, from transportation to stationary power generation and even portable electronics. This section will explore how fuel cells are changing the landscape of energy use and their implications for society.
Transportation Sector
Fuel cells are making significant strides in the transportation sector, particularly with hydrogen fuel cell electric vehicles (FCEVs). Unlike conventional internal combustion engines, FCEVs emit only water vapor as a byproduct. This characteristic positions fuel cells as a cleaner alternative that can help reduce air pollution and greenhouse gas emissions.
Major automotive companies like Toyota and Honda are investing heavily in fuel cell technology, developing models such as the Toyota Mirai and the Honda Clarity. These vehicles offer long driving ranges and quick refueling times, resembling traditional gasoline-powered cars. Moreover, advancements in hydrogen production and storage are necessary for these applications to scale up effectively.
"Hydrogen fuel cell vehicles could potentially reduce dependence on fossil fuels while addressing environmental concerns associated with petrol and diesel vehicles."
Several cities are also implementing hydrogen fuel cell buses in public transport systems, demonstrating the adaptability of fuel cell technology for urban mobility. The integration of fuel cells into public transportation can result in cleaner air in densely populated areas, making them an essential component of sustainable urban planning.
Stationary Power Generation
In stationary power generation, fuel cells offer solutions for both residential and commercial applications. They can provide a reliable energy source while enhancing the overall resilience of the power grid. Fuel cells can operate independently or in conjunction with renewable energy systems, making them an excellent choice for distributed energy resources.
For instance, companies are using solid oxide fuel cells (SOFCs) for combined heat and power (CHP) systems, which improve overall energy efficiency. Systems like those from Bloom Energy are already being deployed in various industries, supplying electricity and heat on-site while minimizing emissions.
Additionally, fuel cells can serve as backup power systems for critical infrastructure. Hospitals, data centers, and emergency services benefit from the continuous power supply that fuel cells can provide during grid outages. This reliability enhances energy security, especially in regions prone to natural disasters.
Portable Electronics
The demand for portable electronics has risen dramatically in recent years. Fuel cells are now being developed for mobile devices, offering an alternative to traditional batteries. These fuel cells can provide longer run times and quicker refueling capabilities than the typical lithium-ion batteries found in most consumer electronics today.
For example, the innovative Snapdragon fuel cell system developed by Qualcomm aims to extend battery life for smartphones and tablets. This technology can significantly reduce reliance on traditional charging methods and improve the user experience.
Portable fuel cells are also being explored for use in small-scale applications, such as powering drones and portable medical devices. Their lightweight design and efficient energy output make them ideal for these contexts. As the development of micro fuel cell technology progresses, we may soon see a broader integration of fuel cells into everyday electronic products.
Role of Fuel Cells in Renewable Energy Integration
The integration of fuel cells within renewable energy systems is critical for advancing modern energy solutions. Fuel cells convert chemical energy directly into electricity, offering efficiency that is often superior to traditional combustion processes. This aspect is particularly important when considering the variable nature of renewable energy sources like solar and wind. Factors such as intermittency make fuel cells valuable as they can provide a stable and continuous electricity supply when renewable generation is low.
Fuel cells serve multiple purposes in the broader context of renewable energy. They are essential not only for generating energy but also for ensuring reliable power delivery that can adapt to fluctuating supply and demand scenarios. The technology offers significant advantages that underlie its relevance in energy transition.
Intermittent Energy Solutions
Renewable energy sources, such as wind and solar power, are often subject to fluctuations depending on weather conditions and time of day. This variability can lead to periods when energy generation is insufficient to meet demand. Fuel cells provide an effective solution by functioning as a buffer for excess energy produced during peak generation times. For instance, in scenarios where solar energy generation surpasses usage, the excess electricity can be utilized to produce hydrogen through electrolysis, which is then stored for later use in fuel cells.
Key benefits of fuel cells in addressing intermittent energy include:
- Stability: They can quickly adjust to changes in energy demand, helping stabilize the electrical grid.
- Efficiency: Fuel cells can convert hydrogen back to electricity with notable energy efficiency, reducing losses associated with energy storage.
- Scalability: They can be deployed at various scales, from small portable applications to larger grid-connected systems.
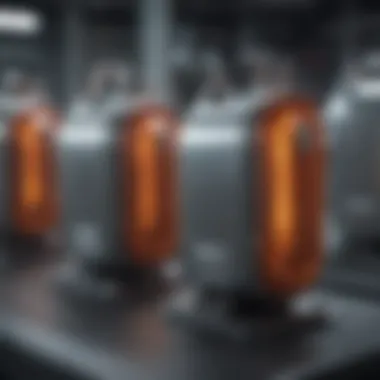
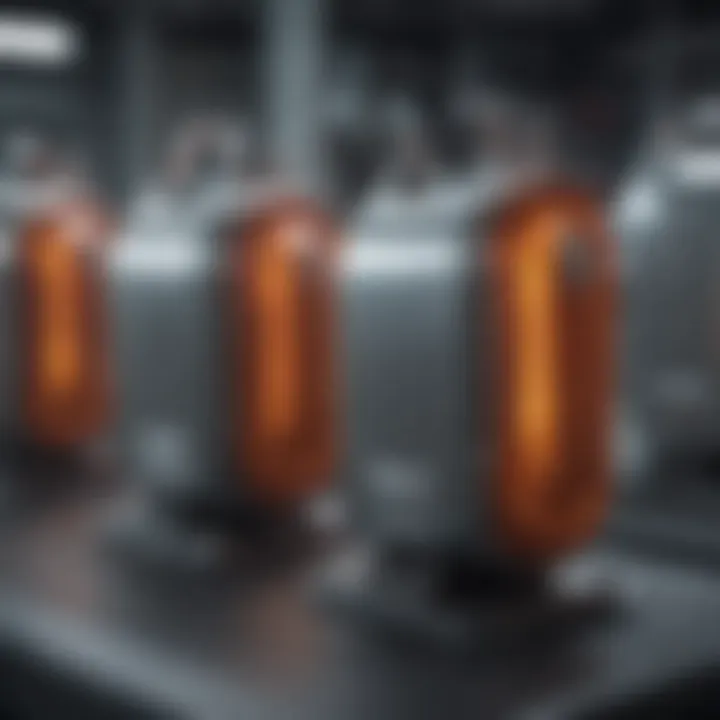
Energy Storage and Distribution
Energy storage is a pivotal aspect of integrating renewable sources into the energy landscape. Fuel cells can store hydrogen for extended periods, overcoming one of the main challenges associated with renewable energy โ its categorically non-continuous nature.
Additionally, fuel cells facilitate smart energy distribution by ensuring a steady output regardless of direct solar or wind activity.
The advantages include:
- Long-term storage: Hydrogen produced through surplus renewable energy can be stored indefinitely, providing a reliable backup supply.
- Flexibility in use: Hydrogen can be converted back to electricity using fuel cells or used as a fuel for transportation, contributing to the versatility of energy systems.
- Decentralized applications: Fuel cells can power local microgrids or home power solutions, reducing reliance on centralized energy production.
Integrating fuel cells with renewable energy systems is not just about technology; it also involves innovative thinking to address contemporary energy demands. However, collaboration among industry stakeholders, researchers, and policymakers will be essential for overcoming challenges in deployment and scaling of this integration, ensuring a sustainable energy future.
"Fuel cells represent a bridge between renewable energy generation and consumption, paving the way for a more resilient energy infrastructure."
By continuing to advance fuel cell technology and integrating it effectively with our existing energy systems, we move closer to achieving a cleaner and more sustainable energy landscape.
Global Impact of Fuel Cell Technology
Fuel cell technology stands at the intersection of environmental sustainability and economic development. Its global impact is substantial, informing policies and practices across various sectors. Fuel cells represent a clean energy alternative, offering solutions to some pressing challenges in the energy landscape. They have the potential to significantly reduce greenhouse gas emissions and can integrate seamlessly with renewable energy sources. Given the escalating urgency of climate change, understanding fuel cells' implications is vital for advancing sustainable practices worldwide.
The widespread adoption of fuel cells can enhance energy security. Since they can be powered by diverse fuels, including hydrogen, their versatility aids in reducing dependency on fossil fuels. Consequently, this technology fosters energy independence. Additionally, as governments and businesses strive to meet emissions targets, fuel cells become a crucial element in their strategies. It is essential to evaluate both the environmental benefits and economic implications of transitioning to fuel cell technology.
Environmental Benefits
Fuel cells offer significant environmental advantages. They produce electricity through electrochemical reactions, emitting only water vapor and heat as byproducts. This characteristic positions them as an attractive alternative to traditional combustion-based generators, which release pollutants into the atmosphere.
- Reduction in Greenhouse Gas Emissions: The implementation of fuel cells can substantially lower CO2 emissions. For instance, hydrogen-powered vehicles contribute little to air pollution compared to internal combustion engine vehicles.
- Conservation of Resources: By utilizing renewable energy sources to produce hydrogen, the reliance on non-renewable natural resources is diminished. This creates a more sustainable energy cycle, promoting resource conservation.
- Improved Air Quality: Fuel cells do not emit harmful pollutants such as nitrogen oxides and sulfur dioxide. Their integration into urban environments can enhance air quality, benefiting public health and reducing healthcare costs associated with pollution-related diseases.
Understanding and implementing these benefits is pivotal for cities aiming for cleaner environments.
Economic Implications
The economic implications of fuel cell technology are equally significant. As this technology matures, it creates opportunities for innovation and job creation, bolstering economic growth across sectors.
- Job Creation: Fuel cell manufacturing and supporting industries can generate a considerable number of jobs. As businesses invest in fuel cell technologies, they stimulate local economies and create diverse employment opportunities.
- Market Expansion: The demand for fuel cells is on the rise, driven by various applications ranging from transportation to stationary power generation. This expanding market presents new business prospects and attracts investments.
- Long-Term Cost Savings: Although the initial investment in fuel cell technology may be high, its operational efficiency leads to long-term savings. The reduced fuel and maintenance costs can offset the upfront expenses, making it an economically viable option over time.
"The transition to fuel cells not only represents a shift to cleaner energy but also provides a pathway for economic resilience and growth."
In summary, the global impact of fuel cell technology highlights its dual role in promoting environmental sustainability and facilitating economic development. By understanding these aspects, stakeholders can make informed decisions regarding future investments and policies.
Future Directions in Fuel Cell Research
The future of fuel cell research is vital in shaping sustainable energy solutions. As this technology gains traction, understanding its trajectory is crucial. New advancements can enhance efficiency, lower costs, and broaden applications of fuel cells. Researchers, educators, and industry specialists are paying attention to emerging trends that promise significant benefits. These trends can lead to innovative solutions that address energy demands and environmental impacts.
Emerging Trends
Several key trends are rising within the field of fuel cell research:
- Hybrid Systems: There is a growing interest in integrating fuel cells with other technologies, such as batteries and renewable energy sources. Hybrid systems can optimize energy use, providing reliable power while leveraging the strengths of each technology.
- Advanced Materials: Research continues into alternative materials for fuel cells. Novel catalysts and membranes can improve performance and longevity, driving down the costs associated with fuel cell systems. For example, developments in nanomaterials may allow for more efficient electrochemical reactions.
- Micro Fuel Cells: As demand for portable energy sources increases, miniaturization of fuel cell technology becomes more relevant. Micro fuel cells can power small devices like smartphones and grasp market share from traditional batteries.
- Integration with Hydrogen Infrastructure: Hydrogen production, storage, and transportation play crucial roles in fuel cell viability. Future efforts are likely directed towards creating a robust hydrogen infrastructure, supporting widespread fuel cell adoption.
Research in these areas can lead to breakthroughs that enhance the practicality of fuel cells in daily applications, making this direction both exciting and necessary.
Collaborative Research Initiatives
Collaboration is a fundamental aspect driving fuel cell research forward. Different institutions and industries are coming together to tackle the complex challenges in this sector. Here are some notable examples of such initiatives:
- University Partnerships: Many universities are developing research alliances focusing on fuel cell technology. Collaborating with industry leaders allows for sharing of resources and expertise, accelerating innovation.
- Public-Private Collaborations: Joint initiatives between government and private sector can lead to extensive funding and support for large-scale projects. These partnerships are essential for moving from pilot projects to commercial viability.
- International Research Networks: With fuel cell technology being a global concern, international collaborations are crucial. Addressing environmental issues requires a collective effort, and numerous countries are joining forces to share findings and technology.
- Industry Consortia: Various organizations form consortia to create standards and promote best practices across the industry. This collaborative space encourages communication, allowing for faster progress in technology development.
"The future of fuel cells depends not only on technological innovation but also on the collaboration between multiple disciplines and industries."
In summary, the future directions in fuel cell research are dynamic and multifaceted. Focusing on emerging trends and enhancing collaborative initiatives can lead to groundbreaking advances in this field, ultimately contributing to a more sustainable energy framework.
End
In summary, the exploration of fuel cell research reveals essential insights into the future of sustainable energy. This article has highlighted the multifaceted nature of fuel cells, from their design and operation to their potential applications across various sectors. In particular, the combination of environmental and economic benefits presents a strong case for further investment in this technology.
Summation of Findings
The findings indicate that fuel cells, particularly in the areas of proton exchange membrane and solid oxide types, are becoming increasingly viable alternatives to traditional energy sources. Key advancements in materials have shown potential for increased durability and reduced costs. The challenges identified, however, must not be overlooked. Technical hurdles, economic factors, and regulatory issues are critical to address in order to foster a healthy ecosystem for fuel cell technology.
Call to Action for Further Research
Further research is needed to overcome existing barriers and refine fuel cell technology. Collaborative efforts among universities, industries, and governments will be crucial in this pursuit. Academia should focus on innovative materials and scalable designs to enhance efficiency. Additionally, studies aimed at understanding the economic dynamics surrounding fuel cells will aid in creating a favorable market environment.
It is critical that researchers and practitioners alike consider the extensive capabilities of fuel cells in combatting climate change and meeting global energy demands. Continued efforts in this domain are vital to harness the true potential of fuel cells and realize their integration with renewable energy systems. In doing so, we can drive the transition towards a more sustainable and secure energy future.